Glucagon-Like Peptide 1: An Introduction and Possible Implications for Neuropsychiatry
Glucagon-like peptide 1 (GLP-1) is an incretin, a gut-derived hormone released from enteroendocrine L-cells (distributed along the entire gastrointestinal tract) after food intake, leading to the secretion of insulin from pancreatic beta cells in a nutrient-dependent manner (12). GLP-1 is also secreted from alpha cells in the pancreas and multiple regions in the central nervous system. There are two bioactive forms of this peptide hormone in the bloodstream: GLP-1-(7–36)-NH2, the more abundant form (approximately 80%), and GLP-1-(7–37), the less abundant form (approximately 20%). GLP-1 has a relatively short half-life of approximately 2–5 minutes once released into plasma. This is a result of (in part) the action of the enzyme dipeptidyl-peptidase-4 and GLP-1’s low molecular weight, which accelerates renal clearance (5, 13).
Both GLP-1-(7–36)-NH2 and GLP-1-(7–37) interact with a specific GLP-1 receptor, a class B G protein–coupled receptor (2). GLP-1 receptors are widely expressed throughout multiple human tissues (e.g., muscles, bones, and adipose) and organs (e.g., gastrointestinal tract, pancreas, kidneys, lungs, heart, blood vessels, and brain) (14, 15). GLP-1 receptors mediate the physiological actions of GLP-1, which include the regulation of glucose homeostasis (decreasing glucose secretion), appetite control (i.e., cravings and satiety), body weight management, metabolism, glycogen synthesis in skeletal muscle and liver, gastric peristalsis, lipid metabolism and modulation of fat absorption, and cardiovascular and neurologic function, among others (2–5).
The extensive anatomical distribution and range of physiological roles of GLP-1 suggest its important pharmacotherapeutic potential. GLP-1 receptor agonist therapies have been used mainly to treat type 2 diabetes mellitus and obesity (3). More recently, new medications (e.g., semaglutide) have become popular because of their efficacy in reducing body weight in obese patients with and without diabetes (16, 17). At present, there are seven GLP-1 receptor agonists approved as medications for type 2 diabetes, cardiovascular disease, and obesity: albiglutide, dulaglutide, exenatide, extended-release exenatide, liraglutide, lixisenatide, and semaglutide (18). The Food and Drug Administration (FDA) approved the first GLP-1 receptor agonist medication, exenatide, in 2005. Semaglutide was the most recent, receiving FDA approval in 2019. Most of these medications are injected subcutaneously, but semaglutide can also be administered orally (typically once daily) (18). Dulaglutide is indicated for hemoglobin A1C management in patients at high risk of cardiovascular disease events. Liraglutide (at a high dose) was approved for obesity management in patients over 12 years old with a body mass index above 30. In 2021, the FDA approved high-dose semaglutide injections for chronic weight management (18). At present, GLP-1 receptor agonist pharmacotherapies are being evaluated for other clinical uses (3). For example, they are promising neuroprotective agents, preventing neuroinflammation and supporting cognitive improvement (19–21).
GLP-1 in the Brain
GLP-1 and its analogs can readily cross the blood-brain barrier (BBB), where its neuroactive properties can benefit nervous tissue (22). This neuropeptide is also synthesized in some brain regions, including hypothalamic nuclei, the nucleus tractus solitarius, and the caudal brainstem (23). GLP-1 receptors are expressed in the caudate, putamen, globus pallidum, hypothalamus, amygdala, hippocampus, cerebellum, and spinal cord (Figure 1) (1). GLP-1 has important neurophysiological attributes, mediating neuronal processes such as mitochondrial function, protein aggregation, and synaptic plasticity (9, 14, 22). In recent years, studies have described a central role for GLP-1 in regulating food intake, providing neuroprotection, decreasing neuroinflammation, amplifying signal transduction, and potentiating cognitive function by counteracting learning dysregulation (3, 5, 24).
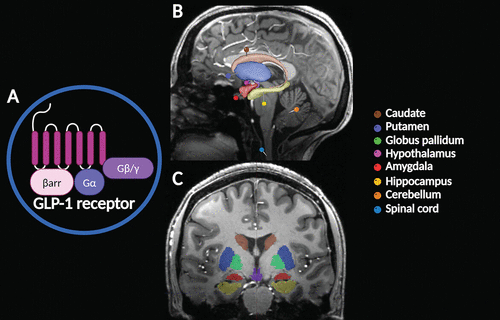
FIGURE 1. Distribution of glucagon-like peptide 1 (GLP-1) receptors in the brain. GLP-1 receptors have a seven-transmembrane structure that interacts with alpha, beta, and gamma subunits of G proteins (Gα, Gβ, and Gγ, respectively) when activated and is inhibited by β-arrestin (βarr) (A). GLP-1 receptors are expressed throughout the central nervous system. Relevant structures are color coded and overlayed onto a sagittal section (B) and a coronal section (C) of the brain (1). GLP-1 receptors mediate physiological actions of GLP-1, which include the regulation of glucose homeostasis (decreasing glucose secretion), appetite control (i.e., cravings and satiety), body weight management, metabolic regulation, glycogen synthesis in skeletal muscle and liver, gastric peristalsis (reducing gastric emptying), lipid metabolism regulation and modulation of fat absorption, cardiovascular regulation, and neurological function (2–5).
GLP-1’s ability to regulate food intake and appetite was originally linked to the midbrain (hypothalamic nuclei) and hindbrain (the nucleus tractus solitarius) (5). However, animal studies revealed that GLP-1 receptors are also expressed in mesolimbic regions of the brain such as the ventral tegmental area and nucleus accumbens, where it mediates (in part) the neural mechanisms of reward and motivation associated with food intake (Figure 2) (6–9). In rodents, the GLP-1 receptor agonist exendin-4 induced anorexia and attenuated alcohol-mediated behaviors through activity in the mesolimbic reward system (7, 25–27). Interactions between GLP-1 and alcohol appear to involve a comprehensive neural circuit, encompassing limbic reward and motivation areas and hindbrain structures (27). In the hindbrain, GLP-1 is synthesized in the neurons of the nucleus tractus solitarius, which project to the reward centers of the brain (6). GLP-1 is a multifaceted neuropeptide that can modulate important behavioral and neurochemical physiological responses linked to alcohol use and the use of other drugs, such as nicotine, opioids, cocaine, and amphetamines (28). GLP-1 analogs have the potential to help treat addictions by inhibiting dopamine release in the reward centers, consequently reducing withdrawal effects and relapses (29). Taken together, evidence from preclinical and clinical studies suggests that the administration of GLP-1 analogs could become a future therapeutic approach to treat substance use disorders (27, 28).
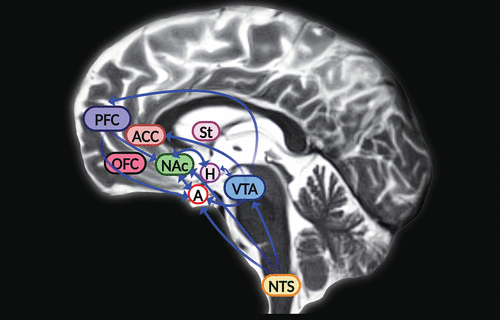
FIGURE 2 and COVER. Schematic of the mesocorticolimbic pathways that mediate eating behavior and appetite (simplified), overlayed onto a sagittal section of the human brain. Glucagon-like peptide 1 (GLP-1) is synthesized in the neurons of the nucleus tractus solitarius (NTS), which project to the reward centers of the brain. GLP-1 receptors are also expressed in mesolimbic regions of the brain, such as the ventral tegmental area (VTA) and the nucleus accumbens (NAc), where GLP-1 mediates (in part) the neural mechanisms of reward and motivation associated with food intake (6–9). The mesolimbic circuit relays dopaminergic signaling from the VTA to the NAc, an area linked to motivation. The VTA and the striatum (St) are linked to reward behavior. The cortical portion of the reward circuit (mesocortical pathway) connects the VTA to the frontal cortex, which includes the anterior cingulate cortex (ACC), prefrontal cortex (PFC), and orbitofrontal cortex (OFC). The OFC is a key area for cognitive processes such as decision making and memory (10). The amygdala (A) is linked to regulation of appetite in response to emotions. GLP-1 receptor agonist activity in the central amygdala is speculated to mediate (in part) anorexigenic effects and mechanisms linked to emotional and motivational feeding (11). The VTA is connected mostly to the lateral hypothalamus (H), which processes signals from various peripheral organs linked to reward signaling and modulation of food intake and energy consumption to maintain basal metabolic functions.
The dopaminergic pathways of the amygdala (among other limbic structures) mediate responses in the reward circuit of the mesolimbic system (30). In the amygdala, dopamine signaling is increased during food ingestion, and GLP-1 is involved in the regulation of feeding behavior (31). Animal studies have shown robust expression of GLP-1 receptors in the central portion and the capsular and lateral regions of the amygdala (11, 32). In rodents, GLP-1 receptors appear to have an important function in feeding motivation, but whether these receptors are expressed in the human amygdala remains unclear (11).
The central amygdala is a complex neuroanatomical region, with a mixed population of orexigenic and anorexigenic cell bodies (11). It receives projections from the nucleus tractus solitarius (11, 33). Thus, the activity of GLP-1 receptor agonist medications in the central amygdala are speculated to mediate (in part) the anorexigenic effects of these compounds. In addition, these medications may regulate mechanisms linked to emotional and motivational feeding (11).
Anatomically and physiologically, astrocytes are among the most important components of the BBB. Astrocytes are central regulators of glucose metabolic processes in the brain (34), and they play a key role in responses to acute ischemic stroke, altering the molecular structure of the BBB and secreting inflammatory chemicals (35). GLP-1 receptors are also expressed in hypothalamic astrocytes (34). Exedin-4 protects the BBB in acute ischemic stroke by decreasing inflammatory responses (36). Recent clinical studies suggest that GLP-1 receptor agonist therapies can reduce the effects of stroke among individuals with type 2 diabetes (37–39).
Neurodegenerative conditions such as Alzheimer’s disease and Parkinson’s disease have been linked to type 2 diabetes and insulin dysregulation in the central and peripheral nervous systems (40, 41). Brain insulin resistance can occur without peripheral insulin resistance, and both have been linked to cognitive decline (42, 43). Furthermore, GLP-1 increases insulin signaling in the brain (44). GLP-1 receptor expression in the brain supports hippocampal synaptic transmission and regulates cellular apoptosis, potentiating the regulation of neurological and cognitive functions (1, 14, 45). GLP-1 receptor deficiency has been linked to a higher risk of seizures and neurodegenerative processes, whereas its overexpression promotes neuroprotection and improved cognition (20, 46). GLP-1 receptor agonist compounds have shown relevant neurostimulatory and neuroprotective activity in animal and human trials. Some studies have indicated that their pleiotropic properties appear to ameliorate aging-related neurodegenerative diseases, particularly Alzheimer’s disease and Parkinson’s disease (20, 44, 47, 48).
Many individuals living with severe mental illness (e.g., schizophrenia spectrum disorders, bipolar disorder, and major depressive disorder) rely on antipsychotic medications (49). However, these therapies provide only limited symptomatic relief from a subset of symptoms and can cause side effects, with some medications increasing the risks of type 2 diabetes, metabolic syndrome, obesity, and cardiovascular diseases (10, 50). In addition, antipsychotics do not target cognitive impairments, which typically accompany some mental illnesses (21, 51, 52).
GLP-1 is a powerful brain-signaling molecule with potential as a neuropsychiatric pharmacotherapy (21, 53). GLP-1 exerts neurostimulatory and neuromodulatory effects, regulating the release of several neurotransmitters, such as serotonin, dopamine, gamma-aminobutyric acid, and glutamate, which mediate (in part) important neurophysiological mechanisms linked to depression-related behaviors and those associated with other psychiatric disorders (54). Results from preliminary studies in animals have indicated that GLP-1 receptor agonists can mediate microglial activity, prevent neuroinflammation, and help improve anxiety-related emotional behaviors (55, 56).
Given the evidence thus far, administration of GLP-1 receptor agonists appears to support cognitive function and may improve mood symptoms among individuals with anxiety and depression (20, 44, 47, 48, 53–56). The proven systemic benefits of GLP receptor agonists can help reestablish overall homeostasis by balancing glycemic control, improving metabolism, and supporting healthy body weight (3–5, 21). Furthermore, GLP-1 receptor agonist therapies may help reverse or alleviate the metabolic side effects of antipsychotic medications (Figure 3) (21, 53).
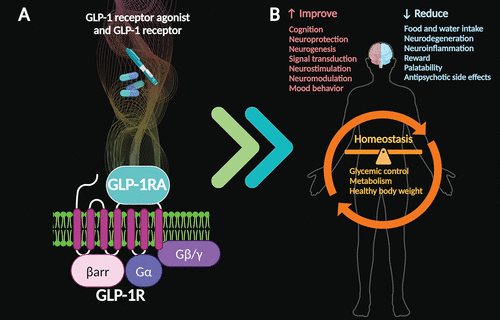
FIGURE 3. Effects of glucagon-like peptide 1 (GLP-1) receptor agonist medications. GLP-1 receptor agonists (GLP-1RA) interact with GLP-1 receptors (GLP-1R), which activate G proteins (Gα, Gβ, and Gγ, respectively) and are inhibited by β-arrestin (βarr) (A). Postulated effects of GLP-1 receptor agonist therapies mediated by the brain include improvements and reductions in particular processes (B). The systemic effects of this activity can help reestablish overall homeostasis.
All images were created with Canva, VH Dissector, and BioRender.com, for which the Mid-Atlantic (VISN 6) MIRECC holds renewable registrations.
Conclusion
GLP-1 is a potent pleiotropic substance, mediating a wide range of organism-level homeostatic mechanisms. GLP-1 receptor agonists are innovative medications with multiple pharmacological actions that influence systemic conditions such as type 2 diabetes, obesity, and cardiovascular disease. The evidence suggests that use of GLP-1 receptor agonist pharmacotherapies may be expanded to treat cerebral vascular accidents, Alzheimer’s disease, and Parkinson’s disease, as well as neuropsychiatric disorders, including anxiety, depression, and substance use disorders. However, more clinical trials are needed to address important factors, such as optimal dosing, gender differences, routes of administration, and undesirable side effects.
1. : Glucagon-like peptide-1 and the central/peripheral nervous system: crosstalk in diabetes. Trends Endocrinol Metab 2017; 28:88–103Crossref, Medline, Google Scholar
2. : Glucagon-like peptide-1 and its class B G protein–coupled receptors: a long march to therapeutic successes. Pharmacol Rev 2016; 68:954–1013Crossref, Medline, Google Scholar
3. : GLP-1 receptor agonists: beyond their pancreatic effects. Front Endocrinol 2021; 12:721135Crossref, Medline, Google Scholar
4. : The extra‐pancreatic effects of GLP‐1 receptor agonists: a focus on the cardiovascular, gastrointestinal and central nervous systems. Diabetes Obes Metab 2014; 16:673–688Crossref, Medline, Google Scholar
5. : Glucagon-like peptide 1 (GLP-1). Mol Metab 2019; 30:72–130Crossref, Medline, Google Scholar
6. : GLP-1 neurons in the nucleus of the solitary tract project directly to the ventral tegmental area and nucleus accumbens to control for food intake. Endocrinology 2012; 153:647–658Crossref, Medline, Google Scholar
7. : Glucagon-like peptide 1 receptors in nucleus accumbens affect food intake. J Neurosci 2011; 31:14453–14457Crossref, Medline, Google Scholar
8. : GLP-1/dexamethasone inhibits food reward without inducing mood and memory deficits in mice. Neuropharmacology 2019; 151:55–63Crossref, Medline, Google Scholar
9. : Distribution of pre-pro-glucagon and glucagon-like peptide-1 receptor messenger RNAs in the rat central nervous system. J Comp Neurol 1999; 403:261–280Crossref, Medline, Google Scholar
10. : Exploring patterns of disturbed eating in psychosis: a scoping review. Nutrients 2020; 12:3883Crossref, Medline, Google Scholar
11. : Anatomical and functional characterization of central amygdala glucagon-like peptide 1 receptor expressing neurons. Front Behav Neurosci 2021; 15:724030Crossref, Medline, Google Scholar
12. : Incretins in obesity and diabetes. Ann N Y Acad Sci 2020; 1461:104–126Crossref, Medline, Google Scholar
13. : From the incretin concept and the discovery of GLP-1 to today’s diabetes therapy. Front Endocrinol 2019; 10:260Crossref, Medline, Google Scholar
14. : GLP-1 receptor localization in monkey and human tissue: novel distribution revealed with extensively validated monoclonal antibody. Endocrinology 2014; 155:1280–1290Crossref, Medline, Google Scholar
15. : Human epicardial fat expresses glucagon-like peptide 1 and 2 receptors genes. Horm Metab Res 2017; 49:625–630Crossref, Medline, Google Scholar
16. : Wegovy (semaglutide): a new weight loss drug for chronic weight management. J Investig Med 2022; 70:5–13Crossref, Medline, Google Scholar
17. : Effects of once-weekly semaglutide on appetite, energy intake, control of eating, food preference and body weight in subjects with obesity. Diabetes Obes Metab 2017; 19:1242–1251Crossref, Medline, Google Scholar
18. : Compare and contrast the glucagon-like peptide-1 receptor agonists (GLP1RAs); in StatPearls. Treasure Island, Fla, StatPearls Publishing, 2023. http://www.ncbi.nlm.nih.gov/books/NBK572151/ Google Scholar
19. : Alternative role of glucagon-like peptide-1 receptor agonists in neurodegenerative diseases. Eur J Pharmacol 2023; 938:175439Crossref, Medline, Google Scholar
20. : Brain uptake pharmacokinetics of incretin receptor agonists showing promise as Alzheimer’s and Parkinson’s disease therapeutics. Biochem Pharmacol 2020; 180:114187Crossref, Medline, Google Scholar
21. : GLP-1 agonists: superior for mind and body in antipsychotic-treated patients? Trends Endocrinol Metab 2022; 33:628–638Crossref, Medline, Google Scholar
22. : The glucagon-like peptide 1 (GLP) receptor as a therapeutic target in Parkinson’s disease: mechanisms of action. Drug Discov Today 2016; 21:802–818Crossref, Medline, Google Scholar
23. : Expression and distribution of glucagon-like peptide-1 receptor mRNA, protein and binding in the male nonhuman primate (Macaca mulatta) brain. Endocrinology 2015; 156:255–267Crossref, Medline, Google Scholar
24. : The glucagon-like peptide-1 receptor agonist reduces inflammation and blood-brain barrier breakdown in an astrocyte-dependent manner in experimental stroke. J Neuroinflammation 2019; 16:242Crossref, Medline, Google Scholar
25. : The glucagon-like peptide 1 (GLP-1) analogue, exendin-4, decreases the rewarding value of food: a new role for mesolimbic GLP-1 receptors. J Neurosci 2012; 32:4812–4820Crossref, Medline, Google Scholar
26. : The glucagon-like peptide 1 analogue exendin-4 attenuates alcohol mediated behaviors in rodents. Psychoneuroendocrinology 2013; 38:1259–1270Crossref, Medline, Google Scholar
27. : Alcohol-mediated behaviours and the gut-brain axis; with focus on glucagon-like peptide-1. Brain Res 2020; 1727:146562Crossref, Medline, Google Scholar
28. : The therapeutic potential of glucagon-like peptide-1 for persons with addictions based on findings from preclinical and clinical studies. Front Pharmacol 2023; 14:1063033Crossref, Medline, Google Scholar
29. : GLP-1a: going beyond traditional use. Int J Mol Sci 2022; 23:739Crossref, Medline, Google Scholar
30. : The brain’s reward system in health and disease. Adv Exp Med Biol 2021; 1344:57–69Crossref, Medline, Google Scholar
31. : Dopamine signaling in the amygdala, increased by food ingestion and GLP-1, regulates feeding behavior. Physiol Behav 2014; 136:135–144Crossref, Medline, Google Scholar
32. : A novel mouse model of glucagon-like peptide-1 receptor expression: a look at the brain. J Comp Neurol 2020; 528:2445–2470Crossref, Medline, Google Scholar
33. Central Nucleus (Amygdala): An Overview. ScienceDirect Topics, 2014. https://www.sciencedirect.com/topics/medicine-and-dentistry/central-nucleus-amygdala Google Scholar
34. : GLP-1 receptor signaling in astrocytes regulates fatty acid oxidation, mitochondrial integrity, and function. Cell Metab 2020; 31:1189–1205.e13Crossref, Medline, Google Scholar
35. : Blood-brain barrier breakdown in acute and chronic cerebrovascular disease. Stroke 2011; 42:3323–3328Crossref, Medline, Google Scholar
36. : Neuroprotective mechanisms of glucagon-like peptide-1-based therapies in ischemic stroke: an update based on preclinical research. Front Neurol 2022; 13:844697Crossref, Medline, Google Scholar
37. : Benefits of GLP-1 (glucagon-like peptide 1) receptor agonists for stroke reduction in type 2 diabetes: a call to action for neurologists. Stroke 2022; 53:1813–1822Crossref, Medline, Google Scholar
38. : Reducing ischemic stroke in diabetes: the role of GLP-1 RAs. J Fam Pract 2023; 72:S55–S60Medline, Google Scholar
39. : Protection against stroke with glucagon-like peptide 1 receptor agonists: a systematic review and meta-analysis. Eur J Neurol 2019; 26:559–565Crossref, Medline, Google Scholar
40. : Shared links between type 2 diabetes mellitus and Alzheimer’s disease: a review. Diabetes Metab Syndr 2016; 10:S144–S149Crossref, Medline, Google Scholar
41. : Antidiabetic agents as a novel treatment for Alzheimer’s and Parkinson’s disease. Ageing Res Rev 2023; 89:101979Crossref, Medline, Google Scholar
42. : Demonstrated brain insulin resistance in Alzheimer’s disease patients is associated with IGF-1 resistance, IRS-1 dysregulation, and cognitive decline. J Clin Invest 2012; 122:1316–1338Crossref, Medline, Google Scholar
43. : Insulin resistance in peripheral tissues and the brain: a tale of two sites. Biomedicines 2022; 10:1582Crossref, Medline, Google Scholar
44. : Glucagon-like peptide-1 (GLP-1) signalling in the brain: from neural circuits and metabolism to therapeutics. Br J Pharmacol 2022; 179:600–624Crossref, Medline, Google Scholar
45. : Glucagon-like peptide-1: a focus on neurodegenerative diseases. Front Neurosci 2019; 13:1112Crossref, Medline, Google Scholar
46. : Novel dual GLP-1/GIP receptor agonists show neuroprotective effects in Alzheimer’s and Parkinson’s disease models. Neuropharmacology 2018; 136:251–259Crossref, Medline, Google Scholar
47. : Therapeutic potential of semaglutide, a newer GLP-1 receptor agonist, in abating obesity, non-alcoholic steatohepatitis and neurodegenerative diseases: a narrative review. Pharm Res 2022; 39:1233–1248Crossref, Medline, Google Scholar
48. : Glucagon-like peptide-1 (GLP-1) receptor agonists and neuroinflammation: implications for neurodegenerative disease treatment. Pharmacol Res 2022; 186:106550Crossref, Medline, Google Scholar
49. : Improving current treatments for schizophrenia. Drug Dev Res 2016; 77:357–367Crossref, Medline, Google Scholar
50. : Comparative effects of 18 antipsychotics on metabolic function in patients with schizophrenia, predictors of metabolic dysregulation, and association with psychopathology: a systematic review and network meta-analysis. Lancet Psychiatry 2020; 7:64–77Crossref, Medline, Google Scholar
51. : Cognitive dysfunction in bipolar disorder and schizophrenia: a systematic review of meta-analyses. Neuropsychiatr Dis Treat 2015; 11:3111–3125Medline, Google Scholar
52. : Antipsychotic drugs: challenges and future directions. World Psychiatry 2018; 17:170–171Crossref, Medline, Google Scholar
53. : Treating cognitive impairment in schizophrenia with GLP-1RAs: an overview of their therapeutic potential. Expert Opin Investig Drugs 2021; 30:877–891Crossref, Medline, Google Scholar
54. : Alleviation of depression by glucagon-like peptide 1 through the regulation of neuroinflammation, neurotransmitters, neurogenesis, and synaptic function. Front Pharmacol 2020:11:1270Crossref, Medline, Google Scholar
55. : The effect of glucagon like peptide-1 receptor agonist on behavioral despair and anxiety-like behavior in ovariectomized rats: modulation of BDNF/CREB, Nrf2 and lipocalin 2. Behav Brain Res 2022; 435:114053Crossref, Medline, Google Scholar
56. : The GLP-1 receptor agonist exenatide ameliorates neuroinflammation, locomotor activity, and anxiety-like behavior in mice with diet-induced obesity through the modulation of microglial M2 polarization and downregulation of SR-A4. Int Immunopharmacol 2023; 115:109653Crossref, Medline, Google Scholar