Insular Cortex: Structural and Functional Neuroanatomy
Although the insula was described by Brodmann and von Economo early in the 20th century, there has been a recent surge in interest in this area. Comprising only approximately 2% of cortical surface area, research over the last two decades supports participation of the insular cortex in a wide range of functional circuits.9 There is growing evidence that the insula is important to neuropsychiatric disorders, especially in relation to dysfunctions involving higher order cognitive, emotional, and social networks. The insula’s primary role is believed to be that of multimodal integration. With this increased research attention, knowledge regarding the insula’s role in clinical syndromes has also increased.
Location and Macroscopic Structure
The insula (Latin for “island” and also called the Island of Reil or the insular cortex) is a triangular area of neocortex that lies underneath the lateral (Sylvian) fissure (Cover and Figure 1). The insular cortex is not visible on an exterior view of the brain, as it is fully covered laterally by opercula of the parietal, frontal, and temporal lobes. Directly medial to the insula are the extreme capsule and the claustrum. The central sulcus of the insula is the most inferior extension of the Rolandic fissure (central sulcus) that separates the frontal and parietal lobes. The central sulcus is the dividing line between the anterior and posterior sectors of the insula. Posterior to the central sulcus is an area of cortex comprised of the two long posterior insular gyri (or gyri longus).2,10,11 Anterior to the central sulcus is a series of shorter gyri (or gyri brevis) (Figure 1). The anterior gyri converge at the ventral-oriented apex. Interindividual variation is seen most prominently with the size and presence of the accessory gyrus and the transverse gyrus, both situated in the anterior lobule.
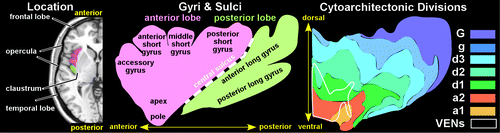
Cover and FIGURE 1. (Cover) The approximate location and extent of the insula is illustrated on sagittal, coronal, and axial MRI scans. The insula is divided by the central sulcus into anterior (pink) and posterior (green) lobes. (Left) The insula’s location between the opercula and the claustrum is illustrated on an axial MRI. (Center) A simplified sagittal drawing of the insula depicts the major gyri. (Right) One way to identify possible functional subdivisions within an area is to create maps of the cellular structure (cytoarchitecture). A recent study in humans reported a progression in cellular structure from the dorsal-posterior granular region (G and g) to the ventral-anterior agranular region (a2–a1) with a transition zone of dysgranular cortex (d3–d1). The locations and extents of the seven cytoarchitectonic subdivisions identified are approximated on a simplified sagittal drawing.1 The locations containing the majority of von Economo neurons (VENs) are indicated (region enclosed by white line).
Microscopic Structure
The insula is isocortex (neocortex) comprised of six distinct layers. Brodmann divided the insula into two regions, separated by the central sulcus: an anterior agranular region (containing pyramidal neurons in layers II and IV) and a posterior granular region (containing granular cells in layers II and IV).2 Later studies led to the development of a concentric model in which the agranular region is located ventral-anteriorly and the granular region is located dorsal-posteriorly, separated by a large band of dysgranular cortex.2 Although there is both species and interindividual variability, the concentric model has been generally supported by more contemporary research.1,12,13 Structural parcellation of the primate insula is an area of active research and debate. Recent studies identified seven distinct subdivisions in the human insula (Figure 1) and 15 in the macaque monkey.1,14
An unusual feature of the insula is the presence of large bipolar (spindle shaped) projection neurons called von Economo neurons (VENs).1,2,15 These are most common in the very anterior region of the insula (also called frontoinsular cortex) (Figure 1). VENs are much larger than nearby pyramidal neurons and have symmetric long narrow apical and basal dendritic arbors.16 On the basis of their size, researchers have suggested that VENs project to other areas of the brain as a fast relay transmission system. Postmortem studies in humans indicate that VENs primarily express transcription factors consistent with involvement in interoceptive functions (e.g., pain, immune, visceral) and subcortical projection targets (e.g., striatum, superior colliculus, basal pons, spinal cord).17,18 Coexpression of other transcription factors was also found, which may indicate secondary projections to intracortical areas.18
Functional Anatomy
Multiple types of evidence and different types of data analyses have elucidated understanding of the heterogeneous and complex functional anatomy of the insula. Multiple impairments have been reported following injury to the insula in humans, including alterations in autonomic functioning, sensory (e.g., gustatory, olfactory, auditory, somatosensory, multimodal) functioning and body awareness, emotional functioning, and language.19,20 Blood supply to the insula is from a main segment of the middle cerebral artery, so isolated insular stroke is unusual.19 Improvement in empathetic functioning has been reported following resection of insular gliomas.21
The starting point for a more detailed understanding of functional anatomy in the human is provided by studies in nonhuman primates. It is important to bear in mind, however, that differences are apparent both when comparing across species of nonhumans primates and from nonhumans primates to humans.22,23 For example, a study comparing of human tractography with monkey tract tracing found that, although a number of tracts were consistent across species, notable differences were observed in others (e.g., arcuate fasciculus, inferior fronto-occipital fasciculus).24 Moreover, intraoperative electrophysiological studies suggest vastly increased reciprocal intrainsular connectivity in humans compared with nonhumans primates.22
Structural Connectivity
In nonhuman primates, evidence supports a posterior to anterior organization of sensory-related areas within the dorsal insula and a limbic-related area in ventral insula.2,25 In the rhesus monkey, projections from vestibular and somatosensory areas go to posterior insula, viscerosensory input goes to midinsula, and gustatory input goes to anterior insula (Figure 2).2 Input from limbic-related areas (e.g., amygdala, temporal polar, parahippocampal, orbitofrontal, and anterior cingulate cortices) goes to the ventral insula (Figure 2).2 In the awake macaque monkey, stimulation of the dorsal posterior insula evoked sensorimotor responses (simple movements of the mouth, face, hand, or limbs).25 Stimulation of the dorsal anterior insula evoked eating-related responses (chewing, mouthing), whereas stimulation ventral to the eating-related region evoked disgust responses (upper lip curling, nose wrinkling, food refusal). Stimulation in the middle region of the ventral insula evoked affiliative behaviors (lip smacking), but only when there was simultaneous eye contact between the monkey and experimenter.25
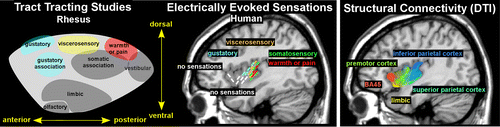
FIGURE 2. (Left) The posterior-anterior progression of functional areas within the dorsal region of the insula is similar across primates, as illustrated here with comparative maps based on tracing of afferents in rhesus monkeys and intraoperative electrical stimulation in humans.2,3 Note that sensory functions are localized primarily to the posterior lobe in human compared with spanning almost the entire insula in rhesus. The most anterior portion of the human insula has been related to nonsensory functions, such as cognition, emotion, and social functioning. (Right) A similar posterior-anterior progression has been demonstrated using diffusion tensor imaging tractography, which provides a way to assess structural connectivity in vivo. A simplified rendition of selected results from a recent study (darker shades indicate stronger connectivity; lighter shades indicate weaker connectivity) is overlaid onto a sagittal MRI.4 The authors of this study emphasized that, although the connections of the anterior and posterior regions differed in expected ways, transitions were gradual rather than abrupt.
Two recent studies using intraoperative electrophysiology in patients with intractable epilepsy provide evidence for a similar posterior to anterior functional organization, but with sensory areas concentrated mostly within posterior insula (Figure 2).3,26 In one study, depth electrodes were used to deliver stimulation to various regions within the insula sufficient to induce symptoms.3 The most posterior locations evoked somatosensory symptoms, with sensations of warmth and/or pain localized more dorsally (Figure 2). Central locations evoked viscerosensory symptoms, with gustatory sensations localized more anteriorly. Anterior locations did not evoke consistent symptoms.3 In the other study, depth electrodes were used to deliver stimulation below threshold for sensations but sufficient to induce cortico-cortical evoked potentials (recorded at other depth electrodes).26 Most of the expected connections between the posterior dorsal insula and sensorimotor areas (e.g., motor, somatosensory and parietal cortices) were confirmed, but not all (e.g., posterior cingulate and supplementary motor cortices). Expected connections between the anterior dorsal insula and cognitive-emotion related areas were also confirmed for some (e.g., hippocampus, temporal pole, frontal operculum, orbitofrontal cortex) but not others (e.g., amygdala, entorhinal and anterior cingulate cortices). As noted by the authors, differences from what has been reported for nonhuman primates could be true species differences and/or a result of low sampling.26
Several groups have used diffusion tensor imaging–based tractography to explore the structural connectivity of the insula in humans.4,27–30 The study that used clustering (k mean) to divide the insula into two functionally distinct regions based on similarities in structural connectivity (strength of connections to other areas) noted that the division between the posterior and anterior subdivisions occurred in the region of the middle short gyrus, following neither expected cytoarchitectonic or gyral boundaries.28 The study that used seven insular regions of interest (dorsal portions of the anterior short, middle short, posterior short, anterior long, posterior long gyri; ventral anterior and ventral posterior) as seeds for tractography identified three subdivisions that agreed more closely to the results of tract-tracing studies in nonhuman primates.27 The anterior region (anterior short gyrus and anterior ventral insula) was primarily connected with orbitofrontal, inferior frontal, and anterior temporal cortices. The posterior region (posterior long gyrus and posterior ventral insula) was primarily connected with temporal cortices. Between was a transition area (middle short, posterior short, anterior long gyri) with mixed frontal and temporal connectivity.27 A study that used voxel-based probabilistic tractography and a different clustering technique (Laplacian eigenmaps) found the expected differences in patterns of connectivity between the posterior and anterior insula but also reported a more complex progression that is reminiscent of cytoarchitectonic mapping (Figure 2).4 Transitions in connectivity were gradual rather than abrupt, and individuals differed considerably in extent and locations of subdivisions. The general anterior-posterior and dorsal-ventral patterns of connectivity were mostly in agreement with expected connections based on nonhuman primates. Although no human diffusion tensor imaging studies have yet confirmed connections to the anterior cingulate cortex known to be present in at least some nonhuman primates, this may be due to the present limitations of tractography.4,30
Functional Connectivity
Whereas structural connectivity studies provide evidence of anatomical connections between brain areas, functional connectivity studies examine which brain regions coactivate (based on time series data) with the area of interest (seed region or voxel) during either task-free (resting state, also called intrinsic functional connectivity) or task-activated conditions. There are multiple differences across studies (e.g., subject selection and number, equipment and data acquisition methods, and data analytic decisions) that make comparisons of results challenging. For these reasons, meta-analyses based on querying the large databases of neuroimaging studies (e.g., NeuroSynth, BrainMap, and 1000 Functional Connectomes Project) that are now available provide a more robust method of examining insular functional connectivity than reviewing single site studies alone.
Resting State Connectivity
Although both seed- and voxel-based approaches have been used to subdivide the insula based on similarities in resting state functional connectivity, a strength of voxel-based approaches is the potential to provide more detailed parcellations.5–7,31 One meta-analysis agreed with an earlier single site study that three subdivisions (dorsal anterior, ventral anterior, posterior) were the optimal solution, although there were differences in the anatomic boundaries reported (Figure 3) and in the areas reported to be functionally connected to each subdivision.5,7 The other meta-analysis examined multiple solutions (2–15 subdivisions) and identified several that had high consistency.6 The authors suggested that a number of valid models of functional differentiation can be obtained depending on level of specificity desired.6
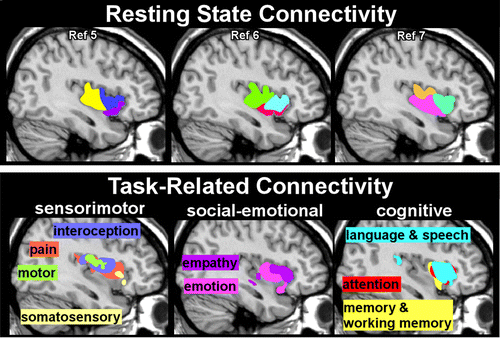
FIGURE 3. Neuroimaging measures of functional connectivity are based on using time series data to identify areas that are changing in activity at the same time and in the same way as the area of interest (seed region of interest or voxel). Two types of functional MRI (fMRI) studies have been used to divide the insula into functional subdivisions based on shared patterns of functional connectivity. (Upper row) In resting state fMRI studies, considered to be measuring intrinsic connectivity, the participants are simply relaxing. Although three functional subdivisions (tripartite) are the commonly reported pattern, the borders between subdivisions vary. The subdivisions reported by a single site study5 and two meta-analyses6,7 are approximated on sagittal MRIs to provide a rough visual comparison. (Lower row) In task-activated studies fMRI studies, participants are performing a specific task. Functional subdivisions can be identified by where the task-related activations occur. These illustrations are based on a recent meta-analysis that segmented the insula into subdivisions with functional specificity by grouping multiple tasks into four functional domains.8 The areas activated by each task for the three domains are approximated on sagittal MRIs.
Task-Related Connectivity
As noted above, this type of connectivity identifies areas that are simultaneously active during a specific task. It is important to bear in mind that an area within the insula might be activated only by a specific functional domain of tasks (low diversity, suggesting functional specificity) or might be activated by a wide range of task domains (high diversity, suggesting multimodal integration and/or domain-general functions).32 Similarly, a specific functional domain of tasks may be associated with activation within one region or within several. Several studies have used the three subdivisions defined in resting state connectivity studies, clustering higher cognitive functions (e.g., executive control) with the dorsal anterior subdivision; emotional processing, chemosensory (e.g., gustatory, olfactory), and autonomic functions with the ventral anterior subdivision; and sensorimotor and language functions with the posterior subdivision.5,7,32 However, the most recent of these meta-analyses noted that all 32 BrainMap task domains engaged each of the three putative subdivisions some of the time, suggesting that a more nuanced approach is required.32
Some studies have grouped tasks into functional domains and identified functional subdivisions by where the task-related activations occurred.6,8,32 One meta-analysis focused on the 13 functional categories (emotion, empathy, olfactory, gustatory, interoception, pain, somatosensation, motion, attention, language, speech, working memory, memory) most frequently associated with activations within the insula. They grouped these into four domains (social-emotional, olfacto-gustatory, cognitive, sensorimotor) to identify four subdivisions with functional specificity.8 No area was identified that activated to all functional domains. A small area in the dorsal anterior insula was identified as supporting multimodal integration because it was activated across all domains other than somatosensory and motor. Another meta-analysis assessed both resting state functional connectivity and task-related connectivity based on BrainMap’s six primary behavioral domains (action, cognition, perception, emotion, interoception, pharmacology).6 Evaluation of cross-modal agreement between these two types of functional data indicated that agreement was best for two, nine, and 13 subdivisions. The authors emphasized that there was a high degree of overlap in subregions activated by particular behavioral domains, consistent with presence of integrative functions (Figure 3).6
A number of theories have arisen that attempt to unify the functional entirety of the insula. In Craig’s “sentient self” model, information moves anteriorly across the insula.33,34 A broad range of somatotopically organized interoceptive information is received by the posterior insula. Moving to the midinsula, the interoceptive information is integrated with input from higher sensory cortices and the limbic system. This stage of processing adds homeostatic information and emotional salience to the interoceptive representation. In the anterior insula, the representation is further enriched by integration of input from a large number of cortical areas, forming a unified coherent representation that may be the neural basis for the sense of self. Menon and Uddin35 proposed that the insula functions in identifying salient external events, modulating the switch from basic attention to working memory and the activation of the sympathetic nervous system, and initiating the anterior cingulate cortex to quickly generate a motor response. Nieuwenhuys2 proposed that the anterior insula together with the anterior cingulate comprise a “core control network that guides all mental activity and behavior in humans.” (p. 132). It has also been proposed that the insula subsumes two main meta-functions: an emotional-salience/attentional control network and a skeletal-motor integration network.31 In any case, more research is needed, and sources seem to agree that the insula is involved in complex and multimodal functions.
Neuropsychiatric Disorders
In addition to having a wide range of functions, the insula has a prolonged developmental course that continues well into adolescence.29 Thus, it is not surprising that this area has been implicated in many neuropsychiatric disorders. Studies in specific disorders are helpful in understanding the insula’s contributions to behavior.
Frontotemporal dementia (FTD; now called frontotemporal neurocognitive disorder) refers to several related conditions resulting from frontotemporal lobar degeneration. The anterior insula is particularly vulnerable to pathological processes seen in FTD, most notably with behavioral variant FTD (bvFTD), in which gray matter loss in frontal and anterior insular regions has been shown early in disease progression.36 Poor judgment, impulsivity/disinhibition, and lack of empathy are clinical features of bvFTD, deficits consistent with the functions of the anterior insula region. Additionally, VENs, which are found primarily in anterior insular and anterior cingulate cortices, seem to be at increased vulnerability in this disorder.37 Loss of these specialized neurons may help explain the clinical manifestation of bvFTD.37 In a small but interesting study comparing patients with bvFTD to patients with Alzheimer’s disease and healthy control subjects, recall of details (multiple choice recognition memory) after a 1-hour delay was compared for an emotionally arousing story versus a neutral story.38 Both the control and Alzheimer’s disease groups had better memory for the emotional story, but the bvFTD group demonstrated a similar recall of details for both stories indicating compromise of the expected enhancement of memory conferred by emotional content. Structural imaging analysis (voxel-based morphometry) indicated that emotional enhancement of memory correlated with integrity of different regions in Alzheimer’s disease (hippocampus, parahippocampal, fusiform and frontal polar cortices) and bvFTD (orbitofrontal cortex, amygdala, insula).
Decreased volume of the insula has also been implicated in schizophrenia. A meta-analysis found medium effect sizes for reduced insular volume in schizophrenia compared with control subjects, with more substantial differences in the anterior than posterior insula.39 However, there were not significant differences across the stage of illness, suggesting that volume reduction might be a predisposing risk factor. In contrast, an MRI study on 80 monozygotic twin pairs found reduced posterior insular gray matter volume in twins with schizophrenia compared with siblings without the illness.40 Functionally, the insula seems to activate with positive symptoms. Impaired insular function may relate to an inability to differentiate the self from the nonself in patients with schizophrenia, thus driving delusional symptoms.41–43 The insula has also been linked to hallucinations,44 perhaps due to impaired salience network functioning.45,46
Conclusions
Due to the recent surge in interest in the insula, it is becoming clear that this region is quite complex. This small cortical area is important in a variety of higher-level and multimodal integrative functions. As additional studies are completed, the role of the insula in normal functioning and in neuropsychiatric conditions should be further elucidated.
1 : The human insula: Architectonic organization and postmortem MRI registration. Neuroscience 2013; 236:117–135Crossref, Medline, Google Scholar
2 : The insular cortex: a review. Prog Brain Res 2012; 195:123–163Crossref, Medline, Google Scholar
3 : Functional neuroanatomy of the insular lobe. Brain Struct Funct 2011; 216:137–149Crossref, Medline, Google Scholar
4 : Probabilistic tractography recovers a rostrocaudal trajectory of connectivity variability in the human insular cortex. Hum Brain Mapp 2012; 33:2005–2034Crossref, Medline, Google Scholar
5 : Three systems of insular functional connectivity identified with cluster analysis. Cereb Cortex 2011; 21:1498–1506Crossref, Medline, Google Scholar
6 : A convergent functional architecture of the insula emerges across imaging modalities. Neuroimage 2012; 61:1129–1142Crossref, Medline, Google Scholar
7 : Decoding the role of the insula in human cognition: functional parcellation and large-scale reverse inference. Cereb Cortex 2013; 23:739–749Crossref, Medline, Google Scholar
8 : A link between the systems: functional differentiation and integration within the human insula revealed by meta-analysis. Brain Struct Funct 2010; 214:519–534Crossref, Medline, Google Scholar
9 : Once an island, now the focus of attention. Brain Struct Funct 2010; 214:395–396Crossref, Medline, Google Scholar
10 : The insular lobe of Reil—its anatamico-functional, behavioural and neuropsychiatric attributes in humans—a review. World J Biol Psychiatry 2004; 5:176–200Crossref, Medline, Google Scholar
11 : The insula: anatomic study and MR imaging display at 1.5 T. AJNR Am J Neuroradiol 2004; 25:222–232Medline, Google Scholar
12 : Cytoarchitecture and probabilistic maps of the human posterior insular cortex. Cereb Cortex 2010; 20:1448–1461Crossref, Medline, Google Scholar
13 : The insula of Reil revisited: multiarchitectonic organization in macaque monkeys. Cereb Cortex 2012; 22:175–190Crossref, Medline, Google Scholar
14 : Modular architectonic organization of the insula in the macaque monkey. J Comp Neurol 2014; 522:64–97Crossref, Medline, Google Scholar
15 : Functional anatomy of cortical areas characterized by Von Economo neurons. Brain Struct Funct 2013; 218:1–20Crossref, Medline, Google Scholar
16 : The von Economo neurons in frontoinsular and anterior cingulate cortex in great apes and humans. Brain Struct Funct 2010; 214:495–517Crossref, Medline, Google Scholar
17 : Biochemical specificity of von Economo neurons in hominoids. Am J Hum Biol 2011; 23:22–28Crossref, Medline, Google Scholar
18 : Human von Economo neurons express transcription factors associated with layer V subcerebral projection neurons. Cereb Cortex (Epub ahead of print, Aug 19, 2013)Google Scholar
19 : Clinical effects of insular damage in humans. Brain Struct Funct 2010; 214:397–410Crossref, Medline, Google Scholar
20 : Highlights in basic autonomic neuroscience: Insular cortex injury leads to cardiovascular dysfunction. Auton Neurosci 2014Crossref, Medline, Google Scholar
21 : Recovery of empathetic function following resection of insular gliomas. J Neurooncol 2014; 117:269–277Crossref, Medline, Google Scholar
22 : Intrainsular functional connectivity in human. Hum Brain Mapp 2014; 35:2779–2788Crossref, Medline, Google Scholar
23 : A volumetric comparison of the insular cortex and its subregions in primates. J Hum Evol 2013; 64:263–279Crossref, Medline, Google Scholar
24 : Monkey to human comparative anatomy of the frontal lobe association tracts. Cortex 2012; 48:82–96Crossref, Medline, Google Scholar
25 : Functional organization of the insula and inner perisylvian regions. Proc Natl Acad Sci USA 2012; 109:10077–10082Crossref, Medline, Google Scholar
26 : Functional connectivity of insular efferences. Hum Brain Mapp 2014; 35:5279–5294Crossref, Medline, Google Scholar
27 : The variation of function across the human insula mirrors its patterns of structural connectivity: evidence from in vivo probabilistic tractography. Neuroimage 2012; 59:3514–3521Crossref, Medline, Google Scholar
28 : Connectivity-based parcellation reveals interhemispheric differences in the insula. Brain Topogr 2012; 25:264–271Crossref, Medline, Google Scholar
29 : Development of insula connectivity between ages 12 and 30 revealed by high angular resolution diffusion imaging. Hum Brain Mapp 2014; 35:1790–1800Crossref, Medline, Google Scholar
30 : Differential structural and resting state connectivity between insular subdivisions and other pain-related brain regions. Pain (Epub ahead of print, July 15, 2014)Google Scholar
31 : Functional connectivity of the insula in the resting brain. Neuroimage 2011; 55:8–23Crossref, Medline, Google Scholar
32 : Beyond the tripartite cognition-emotion-interoception model of the human insular cortex. J Cogn Neurosci 2014; 26:16–27Crossref, Medline, Google Scholar
33 : How do you feel—now? The anterior insula and human awareness. Nat Rev Neurosci 2009; 10:59–70Crossref, Medline, Google Scholar
34 : The sentient self. Brain Struct Funct 2010; 214:563–577Crossref, Medline, Google Scholar
35 : Saliency, switching, attention and control: a network model of insula function. Brain Struct Funct 2010; 214:655–667Crossref, Medline, Google Scholar
36 : Frontal paralimbic network atrophy in very mild behavioral variant frontotemporal dementia. Arch Neurol 2008; 65:249–255Crossref, Medline, Google Scholar
37 : von Economo neurones are selectively targeted in frontotemporal dementia. Neuropathol Appl Neurobiol 2013; 39:572–579Crossref, Medline, Google Scholar
38 : Frontal and temporal lobe contributions to emotional enhancement of memory in behavioral-variant frontotemporal dementia and Alzheimer’s disease. Front Behav Neurosci 2014; 8:225Crossref, Medline, Google Scholar
39 : Systematic meta-analysis of insula volume in schizophrenia. Biol Psychiatry 2012; 72:775–784Crossref, Medline, Google Scholar
40 : Regional gray matter volume in monozygotic twins concordant and discordant for schizophrenia. Biol Psychiatry 2010; 67:956–964Crossref, Medline, Google Scholar
41 : The insula-claustrum region and delusions in schizophrenia. Schizophr Res 2011; 133:77–81Crossref, Medline, Google Scholar
42 : Exploring the neural correlates of delusions of reference. Biol Psychiatry 2011; 70:1127–1133Crossref, Medline, Google Scholar
43 : At the boundary of the self: the insular cortex in patients with childhood-onset schizophrenia, their healthy siblings, and normal volunteers. Int J Dev Neurosci 2014; 32:58–63Crossref, Medline, Google Scholar
44 : Structural correlates of auditory hallucinations in schizophrenia: a meta-analysis. Schizophr Res 2012; 137:169–173Crossref, Medline, Google Scholar
45 : Aberrant dependence of default mode/central executive network interactions on anterior insular salience network activity in schizophrenia. Schizophr Bull 2014; 40:428–437Crossref, Medline, Google Scholar
46 : Reality distortion is related to the structure of the salience network in schizophrenia. Psychol Med 2011; 41:1701–1708Crossref, Medline, Google Scholar