An Analysis of Regional Cerebral Blood Flow in Impulsive Murderers Using Single Photon Emission Computed Tomography
Not all aggression turns to violence, as aggressive postures can be strategic and calculated to realize specific nonviolent goals; and likewise, not all violence results from threat-driven aggression but instead from disaffected predatory interests. 1 Our research interest here is to look at the neurophysiology of violence as a result of impulsive aggression. However, we must reconcile defensive aggression, which is arguably fear-driven, and impulsive aggression, which is arguably anger-driven.
Brain imaging studies are uncovering the neurophysiology of both fear and anger. The fear circuitry implicates the amygdala in concert with the striatum and frontotemporal cortex. 2 , 3 Imaging studies have found amygdala activation in subjects shown pictures of faces that expressed fear 4 , 5 and sadness, 6 and lesions in the amygdala have been associated with subjects’ inability to perceive threats and interpret emotional expressions and social cues. 7 By contrast, the anger circuitry involves the prefrontal orbital and anterior cingulate cortices. Anterior cingulate serotonin transporter availability has been found to be lower in subjects who exhibit aggressive impulsivity, 8 and in studies where anger was induced in healthy comparison subjects, positron emission tomography (PET) imaging found activations in both the prefrontal orbital and anterior cingulate. 9 , 10 These results have led researchers to speculate that activations in these areas mitigate impulsive expressions of anger and would thus be attenuated in violent, aggressive individuals. 9 , 11 Davidson et al. 11 theorize that dysregulation of the amygdala circuit is connected with a disinhibition of impulse control networks, resulting in an inability to control angry, violent behavior.
Our study offers a further test of the link between violence and impulsivity. Using brain single photon emission computed tomography (SPECT) imaging, which measures relative blood perfusion, we examined a group of murderers, each of whom committed an act of impulsive violence. Prior research using PET found lower glucose metabolism among murderers in the prefrontal and parietal lobes, combined with increased occipital lobe metabolism and abnormally asymmetrical amygdala metabolism, when subjects were given a concentration challenge. 12 Given these results, we hypothesized that, when compared to healthy subjects as a group during resting scans, murderers would show perfusion deficits in the networks implicated in emotional regulation and impulse control, specifically, in the prefrontal orbital, anterior cingulate, and anterior medial-temporal cortices, and increased perfusion in the occipital lobes. Further, we anticipated that these differences would be exacerbated when the two groups were scanned during a computerized go/no-go task that challenged them to allocate attentional resources and inhibit impulsive responses.
METHOD
Over a 7-year period, 46 male murderers between the ages of 17 and 71 sought and received SPECT scanning at a brain imaging clinic prior to their sentencing. Of these, 27 were right-handed men who had received both a resting and concentration scan (via the method described below), and whose crimes were impulsive, nonpredatory acts of violence. Because of changes in brain activity known to be correlated with age, 13 , 14 it is important that a comparison group be precisely age-matched; this is especially true when analyzing relative blood flow wherein an age-related change in regional cerebral blood flow (rCBF) necessarily affects rCBF in other regions. The distribution of ages among murderers was highly positively skewed (skewness=1.44) and non-normally distributed (Shapiro-Wilk W=0.85, prob<0.001). We thus divided the 27 into two groups, under age 30 (N=11) and over age 30 (N=16). Our under 30 group was now normally distributed by age (skewness=0.57; W=0.93, prob=0.46), while our over 30 group still was not (skewness=1.29; W=0.81, prob=0.003). Therefore, although we shall briefly report on our results from analyses on the over 30 group, it is the under 30 group that was our primary experimental focus in this study.
Of the 11 in the under 30 group (mean age=23.7 years, SD=2.9), eight had a history of drug abuse, including cocaine, marijuana, methamphetamine, heroin, ecstasy, and alcohol; three reported prior head trauma without loss of consciousness; two had a history of physical abuse; one had received a diagnosis of schizoaffective disorder prior to seeking a scan, and one had been treated for bipolar disorder prior to seeking a scan. Three subjects had been taking tricyclic antidepressants leading up to the time of their scans (one of whom was also taking benztropine), and one had been taking a serotonin and norepinephrine reuptake inhibitor (SNRI); these subjects had been off of their medications with an appropriate wash-out period prior to their scans (tricyclic antidepressant washout=15 days; SNRI washout=4 days). One other had previously been on a selective serotonin reuptake inhibitor (SSRI). One subject was scanned while taking an antipsychotic. One subject was scanned while taking the thyroid medication euthyroid. Six of the 11 were Caucasian and five were Hispanic. All subjects had the procedure explained prior to their first scan, whereupon they gave written informed consent allowing for their scan data to be used in future anonymous research.
We chose to exclude nonmurderers with a history of impulsive violence for the following reasons. First, murderers constitute a clean experimental group whose history and actions are well-documented, allowing us to more easily categorize them as impulsive or predatory. Second, if we consider the concept of “aggressive violence” as occurring on a continuum, most acts of murder would tend to fall near one extreme. By studying only murderers, we reduce much of the variability in the construct. Finally, murderers are a highly understudied population.
Comparison subjects were scanned in accordance with an Institutional Review Board-approved protocol over a 3-year-period, contemporaneous with the final 3 years of acquiring the experimental group. The comparison group comprised 11 healthy, right-handed men who were age-matched to the experimental group (mean=23.8 years [SD=3.6]); however, we were unable to match for IQ, education, or socioeconomic status. Ten of the 11 were Caucasian and one was Hispanic. Comparison subjects received a clinical history and were screened for significant head trauma, prior drug and alcohol abuse, and psychiatric, psychological, and neurological disorders among themselves and their first-degree relatives according to DSM-IV. In addition, comparison subjects were screened using the Structured Clinical Interview for DSM-IV and the Minnesota Multiphasic Personality Inventory–I. All comparison subjects gave written informed consent.
SPECT Image Acquisition and Rendering
SPECT was used to capture functional images of rCBF as a surrogate for brain activity. In each study, an age- and weight-appropriate dose of technetium technetium-99m exametazime was administered intravenously. Photon emission was captured using a high resolution Picker (Phillips) Prism 3000 triple-headed gamma camera with fan beam collimators. Data were acquired in 128x128 matrices, yielding 120 images per scan with each image separated by 3° spanning 360°, and attenuation correction was performed using general linear methods.
Each subject received two scans, one at rest and one during a concentration challenge, with the second scan being acquired between 24 and 48 hours after the first. Resting images were acquired in the following manner. Experimental subjects and comparison subjects sat upright in a quiet, dimly lit room with eyes open; the bolus was injected at 10 minutes. The subject then sat for an additional 10 minutes postinjection. Concentration images were acquired during a 15-minute computerized go/no-go task that measures inattentiveness, motor impulsivity, and reaction time, Conners’ Continuous Performance Test (CCPT). Again, subjects sat upright in the same light as in the baseline condition. The injection was administered 3 minutes into the CCPT. Unfortunately, some CCPT data were not recorded among subjects in the experimental group.
All images were processed using Odyssey software and all voxels were scaled to the brain maximum. Transaxial slices were oriented horizontally to the anterior-commissure/posterior commissure (AC-PC) line. Coronal, sagittal, and transaxial slice images (6.6 mm apart, unsmoothed) were then rendered in the Picker Odyssey software, which grades voxels based on their relative frequencies of photon emission.
Study Design and Data Analysis
All scans were converted to Analyze format and then coregistered and normalized to a standard anatomical space using SPM2 software. In order to minimize small, aberrant but statistically significant results, we smoothed all scans to 8 mm 3 . We then ran a voxel-by-voxel two-tailed t test comparing scans from the experimental and comparison groups in both the resting and concentration conditions. We constrained the SPM2 analyses to retain only those significant differences comprising at minimum eight contiguous voxels. We used a relative threshold mask of 80%, and excluded voxels outside the brain from the analyses. Correction for multiple comparisons was made using a family wise error (FWE) adjustment 15 with a significance threshold of p=0.05.
RESULTS
SPM2 analysis of resting scans found no significant increases or decreases among the under 30 murderers relative to the comparison subjects. When we removed the constraint of correcting for multiple comparisons, we did see areas of lower rCBF throughout the brain among the murderers, primarily in the orbital and frontal cortices, which were significant at the uncorrected voxel level of p=0.001. However, the two groups showed no statistically significant cluster differences at p=0.05 with the FWE adjustment.
In the analysis of concentration scans, we found no areas of significant increased rCBF among the murderers relative to the comparison subjects. However, we found notable decreased rCBF in the murderers throughout the brain, comprising 27 clusters of decreased activity meeting the eight-voxel minimum ( Figure 1 ). The most notable deficit was a large 71,665-voxel cluster spanning most of the orbital and dorsolateral prefrontal cortices bilaterally, extending through the dorsal and subgenual cingulate gyrus, bilaterally through the caudate nuclei and striatum, and bilaterally into the anteromedial temporal lobes and right uncus. Additional large (100 + voxel) deficits had minima in the left uncus (129 voxels) spanning the ventral and lateral left temporal lobe); the left precuneus (169 voxels), spanning the occipital lobes and anterolateral parietal lobes; and the medulla (192 voxels). All results are summarized in Table 1 .
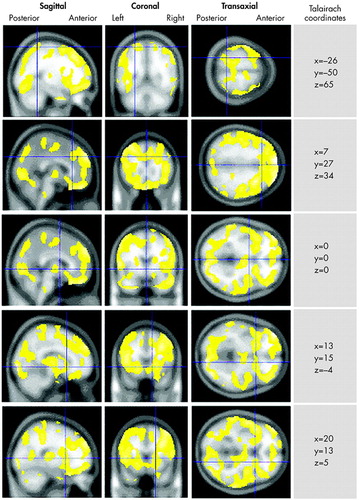
![]() |
We performed similar analyses on the over 30 murderers group, comparing it to a group of 13 age-matched, healthy right-handed men. The family-wise error-adjusted result showed no areas of higher perfusion among murderers during concentration, but it did show a single 156,177-voxel cluster of decreased perfusion (t=9.2, prob<0.0001) covering nearly the entire brain (the minimum was located in the anterior corpus callosum). Similar results obtained when the two groups’ resting scans were compared.
DISCUSSION
In support of our hypothesis, we found marked differences at concentration consistent with previous imaging research on violent subjects. Though we found no areas of higher rCBF among murderers, we saw significantly lower perfusion in brain regions implicated in impulse control, the anterior cingulate and orbital cortices. This circuitry is implicated in anger management, 9 , 11 and deficits here indicate a relative inability to utilize resources involved with inhibition, self-censorship, planning, and future consequences; 16 in this case, when challenged with a concentration task. In addition, we found a bilateral deactivation in the parahippocampal gyri and unci, adjacent to the amygdala. This agrees with Viet et al., 17 who found decreases in the right amygdala coupled with decreased left prefrontal and bilateral anterior cingulate activity in functional magnetic resonance imaging (fMRI) studies on violent psychopaths. 17
In our resting condition analysis, however, we found no clusters of differential rCBF—either increased or decreased—that could distinguish the two groups statistically, a somewhat surprising result given previous resting PET studies that found significantly lower temporal lobe metabolism in violent offenders with personality disorders 18 and schizoaffective disorders. 19 While this may be a function of the different imaging media used, it also highlights the role of impulsivity in our experimental subjects, as when their cognitive resources go untaxed they appear “normal.” Only when they are challenged by the go/no-go CPT do their brains look significantly different from healthy subjects. Thus, unlike the personality and schizoaffective samples studied previously, our sample required an environmental stimulus before neurophysiological deficits became evident.
Consistent with Raine et al., 12 we also found large clusters of decreased bilateral parietal lobe activity among murderers. However, in contrast with this same research, which found significant increases in occipital lobe activity using PET, we found large areas of decreased occipital rCBF among murderers. This disparity may be a function of the different imaging media used in each study, although recent SPECT research has found occipital increases in patients with attention deficit hyperactivity disorder, a disorder having a large impulsivity component. 20 Given the global nature and large size of the deficits we found, particularly in the frontal and parietal lobes, it may be this group’s inability to activate frontally during the CPT that took much of the remaining cortex offline along both dorsal and ventral pathways.
An important limitation to this study was our inability to control for the history of our murder subjects. Although we were able to scan them while they were off of their medication, their disparate histories of psychiatric disorders, drug use, head injury, and their past and present medication use made them a very heterogeneous group. With a sample size of 11, we did not have the power to control for these factors as covariates.
In addition, it could be argued that a more appropriate comparison group would have been a patient sample with a history of drug and alcohol abuse. Unfortunately, matching for the comorbidity and drug history among our murderers was beyond our capacity for this study. It could be further argued that a comparison group composed of murderers who planned their crime might have better isolated the neurology underlying impulsivity. However, the class of calculated violent acts, while not impulsive, is too complex to parse for the purposes of this study: these acts can be disaffected or driven by a range of emotions other than anger; they can be sociopathic, revenge-seeking, or materially self-serving, for example. Our simple goal was to explore the circuitry of anger-driven impulsive aggression, and we feel a healthy reference group allowed us to do this.
As a final point, is interesting to note that the prefrontal deactivations among murderers in this study occurred in response to an emotionally neutral task, a computerized CPT. Davidson et al. 11 have speculated that it is dysregulation of the emotional circuitry, the amygdala/frontal/striatal network, which results in the orbital cortex going offline in violent aggressors. Our results suggest that an emotionally laden stimulus is not required to disrupt the frontal circuitry in violent individuals. Further studies might explore whether the opposite is, in fact, true: that prefrontal dysregulation results in dysfunctional emotional circuitry. In conclusion, our results suggest that murderers who commit acts of impulsive violence show a marked inability to utilize important cognitive resources when challenged by emotionally neutral tasks.
1 . Scarpa A, Raine A: Psychophysiology of anger and violent behavior. Psychiatr Clin North Am 1997; 20:375–394Google Scholar
2 . Armony JL, LeDoux JE: How the brain processes emotional information. Ann N Y Acad Sci 1997; 821:259–270Google Scholar
3 . Blair RJR, Morris JS, Frith CD, et al: Dissociable neural responses to facial expressions of sadness and anger. Brain 1998; 122:883–893Google Scholar
4 . Phillips ML, Young AW, Senior C, et al: A specific neural substrate for perceiving facial expressions of disgust. Nature 1997; 389:495–498Google Scholar
5 . Whalen PJ, Rauch SL, Etcoff NL, et al: Masked presentations of emotional facial expressions modulate amygdala activity without explicit knowledge. J Neurosci 1998; 18:411–418Google Scholar
6 . Wang L, McCarthy G, Song AW, et al: Amygdala activation to sad pictures during high-field (4. tesla) functional magnetic resonance imaging. Emotion 2005; 5:12–22Google Scholar
7 . McDonald AJ: Cortical pathways to the mammalian amygdala. Prog Neurobiol 1998; 55:257–332Google Scholar
8 . Frankle WG, Lombardo I, New AS, et al: Brain serotonin transporter distribution in subjects with impulsive aggressivity: a positron emission study with [11C]McN 5652. Am J Psychiatry 2005; 162:915–923Google Scholar
9 . Dougherty DD, Shin LM, Alpert NM, et al: Anger in healthy men: a PET study using script-driven imagery. Biol Psychiatry 1999; 46:466–472Google Scholar
10 . Kimbrell TA, George MS, Parekh PI, et al: Regional brain activity during transient self-induced anxiety and anger in healthy adults. Biol Psychiatry 1999; 46:454–465Google Scholar
11 . Davidson RJ, Putnam KM, Larson CL: Dysfunction in the neural circuitry of emotion regulation: a possible prelude to violence. Science 2000; 289:591–594Google Scholar
12 . Raine A, Meloy JR, Bihrle S, et al: Reduced prefrontal and increased subcortical brain functioning assessed using positron emission tomography in predatory and affective murderers. Behav Sci Law 1998; 16:319–332Google Scholar
13 . Giedd JN, Blumenthal J, Jeffries NO, et al: Brain development during childhood and adolescence: a longitudinal MRI study. Nat Neurosci 1999; 2:861–863Google Scholar
14 . Sowell ER, Thompson PM, Holmes CJ, et al: In vivo evidence for post-adolescent brain maturation in frontal and striatal regions. Nat Neurosci 1999; 2:859–861Google Scholar
15 . Penny W: SPM Short Course; notes. http://www.fil.ion.ucl.ac.uk/spm/course/slides05/ppt/infer.pptGoogle Scholar
16 . Bechara A, Damasio H, Damasio AR: Emotion, decision making and the orbitofrontal cortex. Cereb Cortex 2000; 10:295–307Google Scholar
17 . Veit R, Flor H, Erb M, et al: Brain circuits involved in emotional learning in antisocial behavior and social phobia in humans. Neurosci Lett 2002; 328:233–236Google Scholar
18 . Seidenwurm D, Pounds TR, Globus A, et al: Abnormal temporal lobe metabolism in violent subjects: correlation of imaging and neuropsychiatric findings. AJNR 1997;18:625–631Google Scholar
19 . Wong MTH, Fenwick PBC, Lumsden J, et al: Positron emission tomography in male violent offenders with schizophrenia. Psychiatry Res 1997; 68:111–123Google Scholar
20 . Kim BN, Lee JS, Shin MS, et al: Regional cerebral perfusion abnormalities in attention deficit hyperactivity disorder. Eur Arch Psychiatry Clin Neurosci 2002; 252:219–225Google Scholar