Hypocretin/Orexin: A Molecular Link Between Sleep, Energy Regulation, and Pleasure
There has been a flurry of activity since the Hcrt-narcolepsy link was made in 1999. The search for genetic alterations in human narcolepsy has demonstrated that Hcrt and Hcrtr mutations are rare. This is not surprising as most human narcolepsy is not familial and is discordant in identical twins. In 2000, Mignot’s group reported one Hcrt mutation in a case of early onset narcolepsy out of 74 narcoleptic patients studied. 6 What they did find, however, was a global deficiency in Hcrt. In situ hybridization studies revealed an absence of Hcrt mRNA in the hypothalami of narcoleptic patients, and radioimmunoassays showed low levels of Hcrt in the CNS. This was consistent with a previous report from Mignot’s group that 7 out of 9 narcoleptic patients studied had low CSF Hcrt. 7 The likely cause of narcolepsy in humans was found to be a degeneration of Hcrt neurons. An immunohistochemical study of 16 human hypothalamus samples, four narcoleptics, and 12 neurologically healthy subjects, revealed an 85% to 95% reduction in the number of Hcrt neurons in narcoleptic hypothalami. 8 The neuronal loss was limited to Hcrt neurons as melanin-concentrating hormone (MCH) neurons, which are intermixed with Hcrt cells, were unaffected. This raised the possibility of narcolepsy being a neurodegenerative disease such as Alzheimer’s disease or Parkinson’s disease. The association between narcolepsy and HLA-DQB1*0602 made autoimmune disease a possible etiology but to this day, little evidence of immune-mediated neuronal degeneration has been found. 9 However, brain samples are often examined late in the disease process, possibly past the point of detecting any sign of autoimmune disease. Despite strong evidence from animal and human studies that hypocretin plays a role in the development of narcoleptic symptoms, the exact pathophysiological link between hypocretin and narcolepsy in humans remains unclear. Animal data clearly demonstrate that Hcrt knockout mice develop narcolepsy-like symptoms, and the canine model of narcolepsy involves mutated Hcrt receptors. However, the disease process is clearly different in humans and one cannot make inferences based on the animal data. In addition, a recent study showed that 11% of narcoleptics with cataplexy have normal CSF Hcrt levels. 10 What the authors do know is that most humans with narcolepsy have a reduction in Hcrt-producing neurons in the hypothalamus, but the authors do not know whether this is causative of the disease. Studies performed in recent years have demonstrated that there is much more to Hcrt function than sleep regulation ( Figure 1 ) and that the Hcrt peptide system is altered in disease processes other than narcolepsy. Hcrt has a number of autonomic and endocrine functions and has been shown to influence cardiovascular function, reproductive and stress hormone secretion and appetite and energy balance. 11 In addition to narcolepsy, decreased levels of Hcrt have been found in patients with Guillain-Barré syndrome (GBS), acute lymphocytic leukemia (ALL), intracranial tumors, craniocerebral trauma, and CNS infections. 12
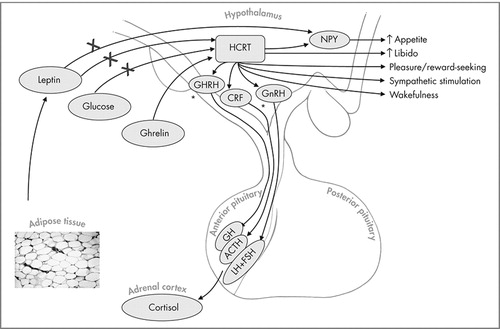
Hypocretin (Hcrt) neurons are present in the dorsal and lateral hypothalamus. Hcrt excretion is inhibited by leptin and glucose and increased by ghrelin. Increased Hcrt promotes wakefulness, increases appetite, is involved in reward/pleasure-seeking behaviors, and stimulates sympathetic activity. Hcrt also promotes corticotropin-releasing factor (CRF) release from the hypothalamus which causes adrenocorticotrophic hormone (ACTH) release from the anterior pituitary. In vitro and in vivo studies have shown increased growth hormone releasing hormone (GHRH) and growth hormone (GH) release from the hypothalamus and anterior pituitary respectively. Increased gonadotropin releasing hormone (GnRH) release from the hypothalamus and follicle stimulating hormone (FSH) and luteinising hormone (LH) from the anterior pituitary have also been shown but the physiological relevance of these interactions remains unclear. As an illustrative example, an individual who consumes large quantities of sweets will have elevated blood glucose levels which would downregulate Hcrt levels. Decreased Hcrt levels would decrease appetite, libido, and cause sleepiness. Over time, if the individual continues on such a diet, increased body fat would cause the down-regulation of Hcrt via leptin.
Autonomic Functions of Hypocretin
When exogenous Hcrt was administered to reverse the symptoms of narcolepsy, the animals experienced an increase in blood pressure and heart rate. 13 , 14 This is not entirely surprising as regions of the hypothalamus and brainstem that are involved in cardiovascular regulation contain Hcrt neurons and express Hcrt receptors. 2 , 15 These effects are caused by activation of the sympathetic branch of the autonomic nervous system (ANS) 13 , 14 but it is also possible that Hcrt can influence the dorsal motor nucleus of the vagus nerve, which is a major parasympathetic center. 16 The ability of Hcrt to stimulate sympathetic activity is fairly well established, however, some groups report depressor effects especially when Hcrt is directly injected into other regions of the brain. de Oliveira et al. 17 report that Hcrt-1 in the nucleus tractus solitarus (NTS) increases vagal activity to the heart and inhibits sympathetic activity. The same group reported similar findings with Hcrt-1 in the nucleus ambiguus. 18 These contradictory findings can be explained by the inherent limitation of in situ experiments with neurotransmitters. Site-specific delivery is not anatomically localized or functionally specific and does not represent true physiological conditions. In vivo experiments seem to indicate that Hcrt has primarily a sympathostimulatory effect. Knockout mice deficient in Hcrt have a significantly lower mean arterial blood pressure (MAP) than normal mice. The difference in MAP in wild-type and mutant mice can be cancelled with α-adrenergic blockade and ganglionic blockade. These treatments lowered the MAP of wild-type mice significantly more than that of mutant mice resulting in MAPs that were statistically identical. Conversely, treatment with angiotensin-converting enzyme (ACE) inhibition or vasopressin antagonism affected the MAP of both wild-type and mutant mice in a similar manner. This suggests that the cardiovascular status of the knockout mice is due to decreased sympathetic vasoconstrictor tone. 19 In human narcoleptics, subnormal heart rate, blood pressure, and forearm blood flow responses to muscle contraction are observed as well as subnormal heart rate response to the Valsalva maneuver. 20
Endocrine Functions of Hypocretin
Experiments involving direct injection of Hcrt into animal brains, including the hypothalamus, have shed light into its role in modulating neuroendocrine pathways. The presence of Hcrt-positive axons in regions of the brain important in anterior pituitary function and the presence of Hcrtr-1 and Hcrtr-2 in the pituitary glands of rats suggest that Hcrt could play an important role in the hypothalamo-pituitary-axis. 21 To date, Hcrt has been found to modulate the release of the hypothalamic hormones, gonadotropin releasing hormone (GnRH), and corticotropin releasing factor (CRF). GnRH-producing neurons express Hcrtr-1 in rat hypothalamus and 75% to 85% of these neurons come in contact with Hcrt fibers. 22 Hcrt-1 has been shown to stimulate GnRH release from rat hypothalamic explants in vitro and modulate LH release in vivo. 23 It remains doubtful, however, that these interactions are physiologically relevant as Hcrt knockout mice are fertile, 5 implying that their LH and FSH levels are normal, despite the fact that they lack Hcrt. Other studies have linked Hcrt to regulation of prolactin (PRL) and growth hormone (GH) release, but these interactions also have yet to be shown to be physiologically relevant. One neuroendocrine interaction that may be important is Hcrt’s effect on stress hormone secretion. Central administration of Hcrt in rats results in increased plasma adrenocorticotrophic hormone (ACTH) and corticotropin-releasing factor (CRF); this increase does not occur if the animal is pretreated with a CRF antagonist. 24 , 25 These animals become obese and are at an increased risk of developing diabetes, as is the case in humans with excess corticosteroid.
Appetite Regulation
The location of Hcrt-positive neurons, namely the lateral hypothalamus, is also known as the “hunger center” of the brain. Lesions in this region decrease eating behavior while stimulation increases eating. Hcrt itself acts as a weak-to-moderate appetite stimulator, but it also interacts with other neuropeptides to modulate feeding behavior. The most convincing evidence of Hcrt’s role in feeding behavior comes from mouse studies in Sakurai’s laboratory. Their group generated transgenic mice in which Hcrt-containing neurons are ablated by expression of a disease gene product, ataxin-3, that is only expressed in Hcrt-containing cells. Over time, these mice become obese, despite eating less, much as human narcoleptics have been described to do. 26
Neuropeptide Y (Npy) is widely recognized as an extremely potent stimulator of feeding behavior and its blockade by antibody or antisense RNAs(1) disrupts this behavior. Many groups have shown that the effect of Hcrt on feeding is, at least in part, mediated by Npy. Hcrt-positive axons connect to Npy neurons in the arcuate nucleus and the Npy neurons express Hcrt receptors. 27 Lopez et al. 28 demonstrated that administration of Hcrt transiently increased Npy expression in the arcuate nucleus of rat hypothalamus. Pretreatment with an Npy antagonist abrogated the appetite-stimulatory effect of Hcrt suggesting the Npy pathway is downstream from Hcrt. Also upstream from Npy is a protein hormone called leptin. Leptin is primarily expressed by adipocytes which allows the body to produce a measure of nutritional status. Leptin receptors are primarily expressed in the hypothalamus. Increased leptin expression decreases synthesis of Npy and, thus, inhibits feeding. Hcrt neurons also express leptin receptors and appear to be regulated by leptin. Human narcoleptics matched for age, sex, body mass index, waist/hip ratio, and fat mass have been shown to have plasma leptin levels about half of those found in control subjects, possibly due to sleep/wake disturbances. 29 Leptin also regulates energy expenditure as measured by oxygen consumption and body temperature. This is consistent with what is observed in human narcoleptics who eat less but still become obese, as well as the Sakurai mice that lose the Hcrt neurons that express the leptin receptor. 26 Decreased Hcrt levels decrease appetite via down-regulation of Npy while increased weight gain may be a result of decreased energy expenditure and metabolism from low circulating plasma leptin. Sakurai’s group has also characterized other metabolic cues that regulate Hcrt neuron activity. Glucose, leptin, and monoamines inhibit Hcrt neurons while ghrelin, a feeding-stimulatory peptide that rises during fasting, stimulates them. The discovery and characterization of the Hcrt peptide system demonstrates the intricate relationship between wakefulness and feeding. Its purpose may be to promote wakefulness when facing an energy shortage.
Pleasure/Reward Pathways
The most recent function attributed to Hcrt is its role in modulating pleasure/reward pathways in the brain. The lateral hypothalamus, in addition to regulating appetite, metabolism and sleep, has been known to be involved in reward-seeking and motivation and has been implicated in addiction and drug-seeking behavior. The neurotransmitters involved in these processes have been unknown up to this point. However, the recent work of Harris et al. 30 suggests that Hcrt is an important mediator of these brain functions. Using a two-chamber, nonbiased, conditioned place-preference (CCP) model to measure the rewarding properties of morphine, cocaine, or food, Harris et al. measured preference for reward by counting the number of times rats would choose the reward chamber versus the nonreward chamber. Using immunohistochemistry, expression of Hcrt was measured during the expression of preference. The amount of Hcrt expression correlated with intensity of reward-seeking while non-Hcrt neuronal activity did not. Harris et al. also report that Hcrt can reinstate an extinguished preference. Rats conditioned to morphine had their preference extinguished by repeated exposure to nonmorphine chambers. Activation of Hcrt neurons by direct injection of rPP (rat pancreatic polypeptide, shown to stimulate Hcrt neurons) into the lateral hypothalamus robustly reinstated the morphine place preference. Interestingly, injection of rPP into other regions of the hypothalamus did not reinstate the behavior. The reinstatement also did not occur when Hcrt receptors were blocked with the Hcrt antogonist, SB 334867. The Hcrt system may also be involved in the mechanism that causes decreased libido in obese individuals. Obese men and women report decreased interest in sex and dissatisfaction with their sex lives. 31 , 32 Postcoital somnolence could also involve Hcrt in a manner similar to postprandial somnolence. The work of Harris et al. demonstrates that food-seeking and drug-seeking behavior is not only linked geographically in the brain, but also by a common neurotransmitter. Understanding the molecular pathways that control food and drug-seeking behaviors will ultimately lead to novel and perhaps more effective approaches in the treatment of addictions. The discovery of a single neurotransmitter that regulates sleep, appetite, metabolism, and reward-seeking behaviors also demonstrates how closely tied these functions are and how integral one has been to the other throughout evolution. Hypocretin may play a role in other neuropsychiatric disorders
Prader-Willi Syndrome
There are a number of neurological disorders that exhibit both sleep and appetite disturbances in which Hcrt function is potentially an important factor. Prader-Willi syndrome is a genetic disease characterized by hypotonia, developmental delay, failure to thrive, increased appetite, and childhood obesity. Sleep disturbances are also common in Prader-Willi syndrome cases. Excessive daytime sleepiness is a common symptom of Prader-Willi syndrome 33 and sleep disordered breathing, sleep-onset REM periods, and cataplexy have also been reported 34 , 35 suggesting possible common pathways between narcolepsy and Prader-Willi syndrome. Though there have been no large studies examining the Hcrt system in Prader-Willi syndrome, a number of smaller studies exist which make the case that disruption of the Hcrt system can explain some of the symptoms observed in this disorder. A recent study by Nevsimalova et al. 36 looked at CSF Hcrt-1 levels in four Prader-Willi syndrome patients. All four patients were extremely obese, two of the four had excessive daytime sleepiness and none had cataplexy. The two cases with excessive daytime sleepiness were found to have the lowest levels of Hcrt (approximately half of normal) while the other two had intermediate or normal levels compared to healthy comparison subjects. Arii et al. 37 reported a 2-week-old baby with severe Prader-Willi syndrome who had intermediate levels of Hcrt. Traditionally, the sleep disturbances seen in Prader-Willi syndrome were thought to be due to weight, but these studies indicate that Hcrt could also play an important role. Similarly, Hcrt could explain the sleepiness experienced by obese women who do not have obstructive sleep apnea. 38 The mechanism for disruption of the Hcrt system appears to be different in Prader-Willi syndrome patients versus narcoleptics however. While narcoleptics appear to have slow degeneration of Hcrt neurons over time, possibly from an auto-immune reaction, Prader-Willi syndrome patients have no change in the number of Hcrt neurons, as recently reported by Fronczek et al. 39 The differing mechanisms for the disruption of the Hcrt system could also explain why the narcolepsy-like symptoms in Prader-Willi syndrome patients differ slightly from those seen in narcoleptics. For example, while both groups have a tendency toward obesity, narcoleptics tend to eat less than healthy subjects while Prader-Willi syndrome patients tend to eat voraciously.
Kleine-Levin Syndrome
Kleine-Levin syndrome is a rare disorder characterized by recurring periods of excessive drowsiness and sleep, at times up to 20 hours per day. Other symptoms include excessive eating, periodic hypersexuality, irritability, disorientation, low energy, and hypersensitivity to noise. These symptoms are transient, lasting days to weeks, and affected individuals are normal in between attacks. Though the cause remains unknown, hypothalamic dysfunction is thought to play a role. Two patients who were investigated for CSF Hcrt levels during an attack were found to have intermediate 40 and normal 41 levels but a study by Dauvilliers et al. 42 was the first to examine CSF Hcrt levels during attacks and normal periods. Four cases investigated during an asymptomatic interval had CSF Hcrt within the normal range, but one patient had a twofold decrease during an attack. This patient also had Prader-Willi syndrome. Clearly, more patients with Kleine-Levin syndrome need to be examined but the findings in this one patient suggest that Kleine-Levin syndrome could possibly be a suitable model for studying the regulation of the Hcrt peptide system.
Summary
The discovery of hypocretin and subsequent linking to narcolepsy and other neurological disorders represents a significant leap forward in sleep research. Not only did it shed light on the molecular etiology of sleep disorders, it demonstrated how closely sleep, feeding, and reward-seeking are related as this single neuropeptide is implicated in all of these behaviors. Elucidating these molecular pathways will help in the understanding of the etiology of neuropsychiatric disorders and offer explanations as to why certain clusters of symptoms are seen in certain disorders or brain lesions. Ultimately, the understanding of the molecular pathogenesis, regulation and natural progression of these disorders will offer new treatment strategies and novel approaches that would otherwise be overlooked. Future studies should examine the prevalence of disrupted eating patterns and impulsivity in narcoleptic patients; inquiring into these behavioral changes in the clinical history may be worthwhile.
1 . de Lecea L, Kilduff TS, Peyron C, et al: The hypocretins: hypothalamus-specific peptides with neuroexcitatory activity. Proc Natl Acad Sci U S A 1998; 95:322–327Google Scholar
2 . Sakurai T, Amemiya A, Ishii M, et al: Orexins and orexin receptors: a family of hypothalamic neuropeptides and G protein-coupled receptors that regulate feeding behavior. Cell 1998; 92:573–585Google Scholar
3 . Lin L, Faraco J, Li R, et al: The sleep disorder canine narcolepsy is caused by a mutation in the hypocretin (orexin) receptor 2 gene. Cell 1999; 98:365–376Google Scholar
4 . Ripley B, Fujiki N, Okura M, et al: Hypocretin levels in sporadic and familial cases of canine narcolepsy. Neurobiol Dis 2001; 8:525–534Google Scholar
5 . Chemelli RM, Willie JT, Sinton CM, et al: Narcolepsy in orexin knockout mice: molecular genetics of sleep regulation. Cell 1999; 98:437–451Google Scholar
6 . Peyron C, Faraco J, Rogers W, et al: A mutation in a case of early onset narcolepsy and a generalized absence of hypocretin peptides in human narcoleptic brains. Nat Med 2000; 6:991–997Google Scholar
7 . Nishino S, Ripley B, Overeem S, et al: Hypocretin (orexin) deficiency in human narcolepsy. Lancet 2000; 355:39–40Google Scholar
8 . Thannickal TC, Moore RY, Nienhuis R, et al: Reduced number of hypocretin neurons in human narcolepsy. Neuron 2000; 27:469–474Google Scholar
9 . Smith AJ, Jackson MW, Neufing P, et al: A functional autoantibody in narcolepsy. Lancet 2004; 364:2122–214Google Scholar
10 . Baumann CR, Bassetti CL: Hypocretins (orexins): clinical impact of the discovery of a neurotransmitter. Sleep Med Rev 2005; 9:253–268Google Scholar
11 . Samson WK, Taylor MM, Ferguson AV: Non-sleep effects of hypocretin/orexin. Sleep Med Rev 2005; 9:243–252Google Scholar
12 . Ripley B, Overeem S, Fujiki N, et al: CSF hypocretin/orexin levels in narcolepsy and other neurological conditions. Neurol 2001; 57:2253–228Google Scholar
13 . Samson WK, Gosnell B, Chang JK, et al: Cardiovascular regulatory actions of the hypocretins in brain. Brain Res 1999; 831:248–253Google Scholar
14 . Chen CT, Hwang LL, Chang JK, et al: Pressor effects of orexins injected intracisternally and to rostral ventrolateral medulla of anesthetized rats. Am J Physiol Regul Integr Comp Physiol 2000; 278:R692–7Google Scholar
15 . Marcus JN, Aschkenasi CJ, Lee CE, et al: Differential expression of orexin receptors 1 and 2 in the rat brain. J Comp Neurol 2001; 435:6–25Google Scholar
16 . Davis SF, Williams KW, Xu W, et al: Selective enhancement of synaptic inhibition by hypocretin (orexin) in rat vagal motor neurons: implications for autonomic regulation. J Neurosci 2003; 23:3844–3854Google Scholar
17 . de Oliveira CV, Rosas-Arellano MP, Solano-Flores LP, et al: Cardiovascular effects of hypocretin-1 in nucleus of the solitary tract. Am J Physiol Heart Circ Physiol 2003; 284:H1369–77Google Scholar
18 . Ciriello J, de Oliveira CV: Cardiac effects of hypocretin-1 in nucleus ambiguus. Am J Physiol Regul Integr Comp Physiol 2003; 284:R1611–20Google Scholar
19 . Kayaba Y, Nakamura A, Kasuya Y, et al: Attenuated defense response and low basal blood pressure in orexin knockout mice. Am J Physiol Regul Integr Comp Physiol 2003; 285:R581–93Google Scholar
20 . Sachs C, Kaijser L: Autonomic regulation of cardiopulmonary functions in sleep apnea syndrome and narcolepsy. Sleep 1982; 5:227–238Google Scholar
21 . Date Y, Mondal MS, Matsukura S, et al: Distribution of orexin/hypocretin in the rat median eminence and pituitary. Brain Res Mol Brain Res 2000; 76:1–6Google Scholar
22 . Campbell RE, Grove KL, Smith MS: Gonadotropin-releasing hormone neurons coexpress orexin 1 receptor immunoreactivity and receive direct contacts by orexin fibers. Endocrinology 2003; 144:1542–158Google Scholar
23 . Small CJ, Goubillon ML, Murray JF, et al: Central orexin A has site-specific effects on luteinizing hormone release in female rats. Endocrinology 2003; 144:3225–3236Google Scholar
24 . Samson WK, Taylor MM, Follwell M, et al: Orexin actions in hypothalamic paraventricular nucleus: physiological consequences and cellular correlates. Regul Pept 2002; 104:97–103Google Scholar
25 . Jaszberenyi M, Bujdoso E, Pataki I, et al: Effects of orexins on the hypothalamic-pituitary-adrenal system. J Neuroendocrinol 2000; 12:1174–118Google Scholar
26 . Hara J, Beuckmann CT, Nambu T, et al: Genetic ablation of orexin neurons in mice results in narcolepsy, hypophagia, and obesity. Neuron 2001; 30:345–354Google Scholar
27 . Rauch M, Riediger T, Schmid HA, et al: Orexin A activates leptin-responsive neurons in the arcuate nucleus. Pflugers Arch 2000; 440:699–703Google Scholar
28 . Lopez M, Seoane LM, Garcia Mdel C, et al: Neuropeptide Y, but not agouti-related peptide or melanin-concentrating hormone, is a target peptide for orexin-A feeding actions in the rat hypothalamus. Neuroendocrinology 2002; 75:34–44Google Scholar
29 . Kok SW, Meinders AE, Overeem S, et al: Reduction of plasma leptin levels and loss of its circadian rhythmicity in hypocretin (orexin)-deficient narcoleptic humans. J Clin Endocrinol Metab 2002; 87:805–809Google Scholar
30 . Harris GC, Wimmer M, Jones GA: A role for lateral hypothalamic orexin neurons in reward seeking. Nature 2005; 437:556–559Google Scholar
31 . Rosen R, Brown C, Heiman J, et al: The female sexual function index (FSFI): a multidimensional self-report instrument for the assessment of female sexual function. J Sex Marital Ther 2000; 26:191–208Google Scholar
32 . Binks M, Adolfsson B: Duke study reports sex, self esteem diminish for morbidly obese. CDS Rev 2005; 98:28–29Google Scholar
33 . Vgontzas AN, Bixler EO, Kales A, et al: Daytime sleepiness and REM abnormalities in Prader-Willi syndrome: evidence of generalized hypoarousal. Int J Neurosci 1996; 87:127–139Google Scholar
34 . Manni R, Politini L, Nobili L, et al: Hypersomnia in the Prader Willi syndrome: clinical-electrophysiological features and underlying factors. Clin Neurophysiol 2001; 112:800–805Google Scholar
35 . Tobias ES, Tolmie JL, Stephenson JB: Cataplexy in the Prader-Willi syndrome. Arch Dis Child 2002; 87:170Google Scholar
36 . Nevsimalova S, Vankova J, Stepanova I, et al: Hypocretin deficiency in Prader-Willi syndrome. Eur J Neurol 2005; 12:70–72Google Scholar
37 . Arii J, Kanbayashi T, Tanabe Y, et al: CSF hypocretin-1 (orexin-A) levels in childhood narcolepsy and neurologic disorders. Neurology 2004; 63:2440–242Google Scholar
38 . Sloan EP, Shapiro CM: Obstructive sleep apnea in a consecutive series of obese women. Int J Eat Disord 1995; 17:167–173Google Scholar
39 . Fronczek R, Lammers GJ, Balesar R, et al: The number of hypothalamic hypocretin (orexin) neurons is not affected in Prader-Willi syndrome. J Clin Endocrinol Metab 2005; 90:5466–5470Google Scholar
40 . Mignot E, Lammers GJ, Ripley B, et al: The role of cerebrospinal fluid hypocretin measurement in the diagnosis of narcolepsy and other hypersomnias. Arch Neurol 2002; 59:1553–1562Google Scholar
41 . Katz JD, Ropper AH: Familial Kleine-Levin syndrome: two siblings with unusually long hypersomnic spells. Arch Neurol 2002; 59:1959–1961Google Scholar
42 . Dauvilliers Y, Baumann CR, Carlander B, et al: CSF hypocretin-1 levels in narcolepsy, Kleine-Levin syndrome, and other hypersomnias and neurological conditions J Neurol Neurosurg Psychiatry 2003; 74:1667–1673Google Scholar