Central Pontine Myelinolysis: A Metabolic Disorder of Myelin
Abstract
Central pontine myelinolysis (CPM) is a clinically heterogeneous, demyelinating condition originally thought to occur only in the central pons.7,8 When demyelination is found in areas outside of the pons, the disorder is referred to as either extrapontine myelinolysis (EPM) or central and extrapontine myelinolysis (CPEPM).1–6 Identification of the importance of severe osmotic stress in the etiology of CPM led to the alternative name of osmotic demyelination syndrome (ODS). Disease severity varies from an incidental asymptomatic finding on imaging or autopsy to coma or death. Frequently, the symptoms of CPM are a combination of neuropsychiatric (e.g., emotional lability, disinhibition, and other bizarre behaviors) and neurologic (e.g., confusion, impaired cognition, dysarthria, dysphasia, gait instability, weakness or paralysis, generalized seizures).9–11 The most common cause of CPM is an overly-rapid correction of hyponatremia in patients with conditions leading to nutritional or electrolyte stress, such as alcoholism, liver disease, immunosuppression after transplantation, malnourishment with underlying medical disease, gastrointestinal disease with acute electrolyte abnormalities, the syndrome of inappropriate secretion of ADH (SIADH), renal disease, cancer, pregnancy, and in high-endurance exercise (in triathletes, marathon runners, etc.).1,4,12,13 Acute hyponatremia (≤48-hour development), as compared with a slowly progressive chronic hyponatremia (>48 hours in development), generally causes a more critical patient presentation, but more chronically developing hyponatremia can portend a higher risk for CPM.4,12,14
The central pons is anatomically unusual in that gray matter and white matter are intermixed (Figure 1), and this feature is presumed to account for the vulnerability of this area to osmotic injury. In most areas of the brain, oligodendrocytes are embedded within white matter and physically isolated from the capillary-rich gray matter.15 It has been proposed that, in CPM, oligodendrocytes in close proximity to gray matter are exposed to a myelinotoxic substance as a result of osmotic stress. Although the mechanism of injury has not been definitely determined, both clinical and animal studies support the central role of osmotic stress, most commonly due to rapid correction of chronic hyponatremia.1,2,4 During acute onset of hyponatremia, water moves from the blood into the brain, causing brain cells (primarily astrocytes) to swell (Figure 2).2,5,6 Brain cells quickly adapt to hyponatremia by losing inorganic osmolytes (electrolytes) in order to reestablish normal cell volume. If the hyponatremic state is corrected during this acute phase, the brain can quickly accumulate electrolytes so as to restore normal osmotic equilibrium. Brain injury is likely to occur only in the most vulnerable patients (e.g., menstruating women, prepubescent children, patients with hypoxemia).2 If the hyponatremic state continues, brain cells must also expel organic osmolytes (e.g., myo-inositol, taurine, glutamine) in addition to electrolytes in order to fully restore normal cell volume. Movement of organic osmolytes is much slower than movement of electrolytes. In the chronic hyponatremic state, brain-cell volume is normal, but the intracellular osmolality is low, in order for the brain to be in osmotic equilibrium with the blood (Figure 2). At this point, correction of hyponatremia must be undertaken slowly in order to allow sufficient time for the brain-cells to reacquire both inorganic (electrolytes) and organic osmolytes. Osmotic stress due to rapid correction of chronic hyponatremia sometimes impairs blood–brain barrier (BBB) function, suggesting a loosening of the tight junctions between vascular endothelial cells (Figure 2). This process may allow an injurious substance (e.g., complement) access to myelin. It has also been proposed that osmotically-stressed vascular endothelial cells release other substances (e.g., plasminogen activator, cytokines) that can injure myelin. An alternative possibility, suggested by the presence of structures similar to Rosenthal fibers within perivascular astrocytes (see below), is that myelin destruction is secondary to impairment in potassium siphoning, as has been recently proposed for several other demyelinating diseases.2,16,17
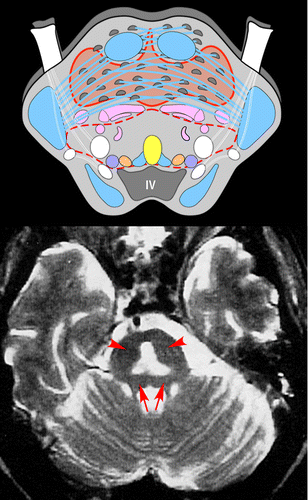
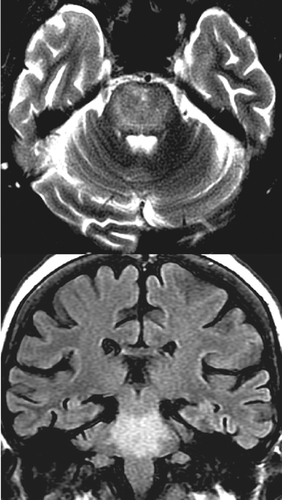
COVER AND FIGURE 1. [Left Top] An axial schematic diagram of the pons (radiographic orientation) illustrates the major nuclei (locus coeruleus, purple; paramedian raphe nuclei, yellow; lateral dorsal tegmental nucleus, light gold; cranial nerve V, white), major tracts (motor, blue; sensory, pink; cranial nerve, white), and the reticular formation (red dashed line). An unusual aspect of the central pons that is believed to underlie its vulnerability to osmotic injury is the intermingling of gray matter (pontine nuclei, dark gray) and white matter (transverse fibers to cerebellum, blue). Osmotic stress often spares the surrounding compact motor and sensory tracts, resulting in the characteristic “trident” or “bat-wing”-shaped area of injury (light orange area). [Left Bottom] Axial T2-weighted MRI illustrates the classic area of injury in the central pons, with sparing of the tegmentum (arrows) and corticospinal tracts (arrowheads). [Right] Axial T2-weighted (top) and coronal FLAIR (bottom) MRIs from a case in which the area of injury was more widespread (image contributed by Dr. Robert Kotloski, Wake Forest University School of Medicine).
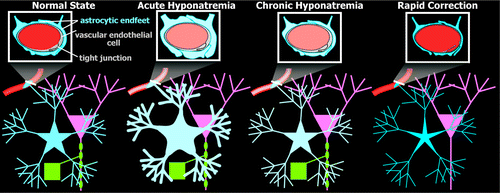
FIGURE 2. Schematics of major changes that occur in the brain during osmotic regulation (astrocytes, blue; neurons, pink; oligodendrocytes and myelin, green; blood vessels, red; changes in osmolality are indicated by changes in color intensity).1–6 [Normal State] The brain and blood are in osmotic balance. Astrocytes extend processes to enclose vessels (single endfoot illustrated), forming part of the blood–brain barrier (BBB). [Acute Hyponatremia] As plasma osmolality decreases, blood becomes dilute, as compared with brain, and water moves from blood into brain, causing brain cells to swell (cerebral edema). Cellular swelling occurs primarily in astroglia, rather than neurons. The brain adapts to hyponatremia by losing solutes in order to reestablish normal cell volume. Initially (in hours), cells expel inorganic osmolytes (electrolytes), and water follows, decreasing cell volume back toward normal size. [Chronic Hyponatremia] If hyponatremia is sustained, cells must also expel organic osmolytes in order to lose more water and restore normal cell volume. This process is much slower (days). In the chronic hyponatremic state, brain cell volume is normal, but the intracellular osmolality is low, to be in balance with the blood. [Rapid Correction] If blood osmolality is raised quickly to normal, the blood becomes much more concentrated than brain, and water moves from brain to blood, causing cells to become dehydrated and shrink. In order to restore normal volume, brain cells must reacquire solutes. Electrolytes are regained relatively quickly; organic osmolytes, very slowly. Osmotic stress caused by rapid correction results in focal loss of oligodendrocytes and myelin, with sparing of neurons and axons, and can cause transient BBB disruption.
Several psychiatric illnesses (in addition to alcoholism) and many neuropsychiatric medications have hyponatremia as a potential complication. Over the past two decades, multiple case reports and case series have been published documenting hyponatremia and resulting CPM in patients with schizophrenia, anorexia, primary psychogenic polydipsia, and “ecstasy” abuse.13,18–21 Among patients with chronic mental illness, 10%–25% may have primary polydipsia, increasing the risk of hyponatremia and premature death.13,21 Primary medications or classes of medications used daily in neuropsychiatry in which hyponatremia is a side effect or reported to occur after use include carbamazepine, oxcarbazepine, the serotonin reuptake inhibitors (SSRIs), lithium, tricyclic antidepressants, opioids, and polypharmacy with multiple antipsychotic drugs.20,22–24 Other classes of commonly used medications that increase risk of hyponatremia include salt-losing diuretics, nicotine, nonsteroidal anti-inflammatory drugs, and acetaminophen.20
CLINICAL COURSE
Historically, the prognosis in CPM or CPEPM has been considered very poor. More recent studies (primarily retrospective chart reviews), however, indicate a considerable range in both clinical course and prognosis in patients with clinically symptomatic CPM and/or CPEPM.14,25–27 The first of these studies examined 44 cases, most (42/44) with chronic alcoholism.25 Of the 32 patients with follow-up data who survived (2 died in the acute phase), 34% (11/32) had a “good outcome” (no significant functional deficits); 34% (11/32) had an “adequate outcome” (minor neurological deficits); and 31% (10/32) had a “poor outcome” (dependent). Another study in patients with alcoholism reported somewhat more favorable outcomes, with 44% (4/9) free of neurological deficits by 6 months after onset.26 The authors noted that most of their cohort (8/9) did not have an episode of acute correction of hyponatremia. A more recent study examined 12 individuals with CPEPM related to a variety of medical causes.14 In this more-diverse population, four patients died in the acute phase, and two were lost to follow-up. The remaining six were reported to have “good motor and cerebellar recovery.” However, all five of the patients who received neuropsychological testing had evidence of subcortical/frontal dysfunction, and most of these (4/5) were unable to return to work. The most recent study is also the only one to include prospective collection of objective follow-up measures of disability and functional status.27 As in the previous study, this cohort was quite diverse in terms of causative conditions. Almost half (12/25) died either during the acute phase (2) or after hospital discharge (10). One was lost to follow-up. At final follow-up (mean 2.2 years; median: 1 year; range: 0–8 years), 29% (7/24) were normal; 17% (4/24) had mild cognitive or extrapyramidal deficits; and 54% (13/24) had a poor outcome (died or were dependent). Univariate analysis indicated that lower initial Glasgow Coma Scores, lower disability rating scales, lower sodium levels, and the presence of hypokalemia all predicted poorer outcomes. Although these studies differ in many respects, some consistent themes are emerging. A common pattern is an initial presentation with delirium, seizures, or encephalopathy, followed by a brief period (i.e., a few days) of lucidity or clarity before the onset of symptoms indicating CPEPM. These studies indicate that a “favorable” prognosis is possible, although few return to previous levels of functioning. The authors of one study made an interesting proposal, based on their results combined with data from previous publications, delineating three groups of patients with distinguishable prognoses.14 Group One included patients who present with seizures and have a “good” prognosis. Group Two included those with cerebellar presentations (i.e., commonly, with severe alcoholism). These patients generally have good physical recovery, with residual cognitive impairment. Group Three comprised those with immunosuppression and liver transplantation. These patients generally present with more severe clinical features and do very poorly. This three-part grouping is in reasonable agreement with the prognostic factors noted above.27
The literature contains only a few reports of neuropsychological test results in cases of CPM and/or EPM. Two case reports included test results within 2 weeks of symptom onset.28,29 A patient with only EPM (lesions in the basal ganglia) had severely impaired attention, verbal and visual memory, visuospatial function, frontal executive function, recognition memory, free recall memory, and naming, with preservation of other language-related functions.29 All these deficits are consistent with previous reports in patients with basal ganglia lesions. In the other case, the patient had CPEPM (lesions in the pons, caudate, lentiform nucleus, thalamus, and internal capsules).28 At 1 week, the patient had prominent deficits in attention and concentration (e.g., high distractibility, slow visual scanning), memory (immediate verbal recall and memory for daily events), visuomotor functioning, and fine motor speed. On reexamination at 4 months, the patient had improved somewhat in some domains, but deficits were still clearly present, and new problems included learning of new verbal information. Although acute neurological and behavioral symptoms had resolved, a new vocal tremor was present. Two other studies included neuropsychological test results obtained 1 month or more after symptom onset. A case report of CPM after liver transplantation noted lower-than-expected intellectual abilities, impairments of attention (auditory, divided, and selective), learning, problem-solving, and ability to use feedback to alter action, as well as pathological crying and laughter at 6 months after symptom onset, all consistent with a brainstem process.30 Finally, in a case series described above, follow-up of five patients included neuropsychological testing (range: 1–19 months after onset of symptoms).14 Although details of measures and scores are not provided, all five were noted to have subcortical/frontal dysfunction, and memory was impaired in two. Some of the patients in these studies had histories of alcoholism before CPM, possibly affecting the test results. Overall, although limited neuropsychological information is available in patients with CPM or CPEPM, many patients have lasting and significant cognitive impairments consistent with other pathological insults to the brainstem or subcortical structures.
PATHOLOGY
CPM was first documented in 1959, in patients with alcoholism.7,8 The association with hyponatremia and rapid correction of the low sodium did not occur until many years later.1–4 The defining neuropathological characteristics of CPM are symmetric, sharply demarcated lesions of the central pons, with selective destruction of myelin sheaths and loss of oligodendrocytes, presence of fat-laden macrophages, and absence of inflammatory infiltrate.7,8 Blood vessels are patent, and both axons and neuronal cell bodies are generally spared. Swollen myelin sheaths and fragmented myelin are present in border regions. Centrally-located hypertrophic astrocytes are only lightly labeled for glial fibrillary acidic protein (GFAP), and GFAP+ structures similar to Rosenthal fibers may be present within astrocytic processes around blood vessels.16 In older lesions, there may be a central area of cavitation in which all elements have degenerated.7,8,16 Evidence of BBB disruption (e.g., green discoloration in the pons in patient with jaundice) may also be present.4 Pathological findings in EPM are similar to those of CPM.1–4
NEUROIMAGING
The appearance of CPM on computed tomography (CT) and MRI has been well characterized.31–36 Standard clinical imaging can be normal for a considerable time (several days to 2 weeks) after onset of the clinical symptoms. After this period, the classic imaging findings of CPM generally emerge, and MRI is more likely to be positive than CT. Lesions are bright on T2-weighted (including fluid-attenuated inversion recovery, FLAIR) MRI sequences. Sparing of the ventrolateral pons, tegmentum, and corticospinal tracts results in the characteristic “trident-shaped” or “bat-winged” appearance (Figure 1). The lesion is hypointense on T1-weighted MRI, and there is no mass effect. Contrast enhancement is not usually seen. When present, enhancement will be noted in border regions.37 If extrapontine lesions are present, they are often bilateral, and the most common locations are the cerebellar peduncles, globus pallidus, thalamus, lateral geniculate body, and putamen.31,38 In many cases, the severity on imaging does not correlate with clinical manifestations.37 However, as the clinical symptoms resolve, the T2-weighted or FLAIR images may demonstrate a decrease in lesion size and intensity.36,37,39 The differential diagnosis of pontine lesion on imaging includes pontine glioma, acute disseminated encephalomyelitis, vasculitis, hypertensive encephalopathy, multiple sclerosis, pontine infarct, and secondary radiation changes.33,40,41
Diffusion-weighted (DW) MRI is a newer pulse sequence that is very sensitive to changes in water motion associated with cellular dysfunction and so may hold promise for early diagnosis of CPM.36 Multiple case reports and case series have recently described the presence of hyperintense pontine lesions with DW MRI in the first few days after symptom onset, when conventional MRI is usually negative.31,37,41–44 Calculation of the apparent diffusion constant (ADC) at various time-points after symptom onset indicates that it is usually low early after symptom onset, then gradually rises to either a normal or somewhat elevated value. Lower-than-normal ADC values are associated with cytotoxic (intracellular) edema, and higher-than-normal ADC values are associated with vasogenic (extracellular) edema.42 The recent demonstration that ADC values are modulated in-vivo by the level of aquaporin expression in astrocytes may also be relevant to CPM.45 Some authors assert that hyperintense lesions on DW MRI associated with low ADC values help to differentiate CPM from the other pontine disorders described above (in the differential diagnosis).37,41,42,44 It has also been proposed that if the ADC values are either normal in the presence of increased signal on T2-weighted MRI, or clear to normal rapidly, the prognosis for recovery is better.37,46 These preliminary results are quite promising, but larger studies are needed to fully understand the clinical benefits in using DW and ADC values in evaluation of the CPM patient.
Only a few studies have used advanced neuroimaging such as positron-emission tomography (PET), single photon-emission computed tomography (SPECT), and MR spectroscopy (MRS) in patients with CPM or CPEPM. One case report of PET examination in a comatose patient with CPEPM reported hypermetabolism in the acute phase (1 week after symptom onset) in the right temporal cortex, thalami, and pons.38 By 1 month, the pontine and thalamic areas were hypometabolic, with the right temporal cortex remaining hypermetabolic. The authors speculated that hypermetabolism might be secondary to pathological microglial and/or astroglial activity. Two case reports of striatal EPM have reported decreased dopamine transporter binding (presynaptic) as measured by SPECT, suggesting osmotic injury to the nigrostriatal dopaminergic system.47,48 In one of these cases, postsynaptic dopamine D2 receptor binding was also assessed and found to be decreased.48 Both cases responded well to carbidopa/l-dopa treatment. Post-treatment resolution of both binding abnormalities was confirmed in one case.48 The authors noted that the initial decreased ligand uptake and subsequent lesion resolution in the basal ganglia clarified the pathology of EPM and correlated with clinical recovery. Changes in proton MRS have been reported in both hyponatremia and CPM. An early study comparing patients with chronic (>1 week) hyponatremia with healthy individuals reported reductions in cortical levels of multiple metabolites, including choline (Cho), creatine/phosphocreatine (Cr), and N-acetylaspartate (NAA), which returned to normal after clinical recovery.49 The authors noted that their results are consistent with the proposal that many of these compounds are osmolytes. A recent study combined proton MRS and MR perfusion to examine the pons in a case of CPM after liver transplantation.44 In the acute stage (8 days after onset), decreased NAA/Cr ratio, increased Cho/Cr ratio, and increased perfusion (as indicated by cerebral blood-volume mapping) were present. In the chronic stage (Day 48), there was further decrease in NAA/Cr and increase in Cho/Cr, and perfusion was decreased. The authors speculated that the acute spectroscopic findings were indicative of neuronal loss and gliosis, with the later findings related to further neuronal loss, but spared gliosis. More studies are required to better understand the functional significance of proton MRS changes, as multiple roles have been proposed for many of these substances.50,51
TREATMENT
Hyponatremia occurs when the serum sodium drops below 135 mmol/liter. Acute hyponatremia is not uncommon in several populations, including hospitalized patients and nursing home residents.12,52 Chronic hyponatremia is more common in outpatient settings.53 Treatment algorithms include considerations of current symptoms and risk for acute decline (to coma, respiratory arrest, or death), acuity of the hyponatremia, presence of chronic malnourishment, underlying medical illnesses or complications, volume status of the patient, and other accompanying electrolyte abnormalities. The choice between correcting hyponatremia with hypertonic 3% saline for brief periods versus much slower initial correction with normal saline requires a thorough clinical assessment and expertise in fluid and electrolyte balance. The overall goal is to achieve correction of serum sodium at a rate not exceeding 0.5 mmol/hour, or 0.5 meq/hour.12,52,53 Frequent checks of serum sodium level are necessary during the stabilization phase. Conivaptan, a vasopressin V1A/V2 receptor antagonist, may be of assistance in treating acute euvolemic hyponatremia, as vasopressin is intimately involved in salt/water balance.12 New interventions may be on the horizon for patients after CPM has occurred. Plasmapheresis and administration of intravenous immune globulins have been reported to improve outcomes.54–56 Other potential treatments include steroids, symptomatic treatment for specific neurologic or neuropsychiatric symptoms (e.g., antiparkinsonian agents for movement disorders or psychiatric medications for agitation), and psychotherapies or behavioral interventions, if psychogenic polydipsia is present.
CONCLUSION
In summary, CPM or CPEPM are devastating and often preventable conditions, with considerable morbidity and/or mortality. Prevention is certainly the key, as current treatment only rarely leads to full recovery. Although imaging advances and a broader neuropathological understanding have improved diagnosis in the last few decades, CPM remains a condition for neuropsychiatrists to be aware of, particularly in view of the multiple neuropsychiatric medication classes in use and the psychiatric illnesses in which hyponatremia is a potential risk.
1. : Central and extrapontine myelinolysis: then and now. J Neuropathol Exp Neurol 2006; 65:1–11Crossref, Medline, Google Scholar
2. : Brain cell volume regulation in hyponatremia: role of sex, age, vasopressin, and hypoxia. Am J Physiol Renal Physiol 2008; 295:F619–F624Crossref, Medline, Google Scholar
3. : Osmotic demyelination syndrome. Am J Med Sci 2010; 339:561–567Crossref, Medline, Google Scholar
4. : Central pontine myelinolysis: historical and mechanistic considerations. Metab Brain Dis 2010; 25:97–106Crossref, Medline, Google Scholar
5. : Brain volume regulation: osmolytes and aquaporin perspectives. Neuroscience 2010; 168:871–884Crossref, Medline, Google Scholar
6. : Brain volume regulation in response to changes in osmolality. Neuroscience 2010; 168:862–870Crossref, Medline, Google Scholar
7. : Central pontine myelinolysis: a hitherto-undescribed disease occurring in alcoholic and malnourished patients. American Medical Association Arch Neurol Psychiatry 1959; 81:154–172Crossref, Medline, Google Scholar
8. : Living legends: central pontine myelinolysis. Adv Clin Neurosci Rehabil 2006; 6:22–22Google Scholar
9. : Behavioral manifestations of central pontine myelinolysis. Arch Neurol 1987; 44:671–673Crossref, Medline, Google Scholar
10. : Psychotic disorder in a patient with central and extrapontine myelinolysis. Psychiatry Clin Neurosci 2007; 61:320–322Crossref, Medline, Google Scholar
11.
12. : Hyponatremia: clinical diagnosis and management. Am J Med 2007; 120:653–658Crossref, Medline, Google Scholar
13. : The assessment and treatment of water imbalance in patients with psychosis. Clin Schizophr Relat Psychoses 2010; 4:115–123Crossref, Medline, Google Scholar
14. : Central pontine and extrapontine myelinolysis: from epileptic and other manifestations to cognitive prognosis. J Neurol 2010; 257:1176–1180Crossref, Medline, Google Scholar
15. : Osmotic stress, osmotic myelinolysis, and oligodendrocyte topography. Arch Pathol Lab Med 1989; 113:1386–1388Medline, Google Scholar
16. : Changes in glial cell markers in recent and old demyelinated lesions in central pontine myelinolysis. Acta Neuropathol 1990; 80:46–58Crossref, Medline, Google Scholar
17. : Molecular disruptions of the panglial syncytium block potassium siphoning and axonal saltatory conduction: pertinence to neuromyelitis optica and other demyelinating diseases of the central nervous system. Neuroscience 2010; 168:982–1008Crossref, Medline, Google Scholar
18. : Psychogenic polydipsia review: etiology, differential, and treatment. Curr Psychiatry Rep 2007; 9:236–241Crossref, Medline, Google Scholar
19. : Central pontine myelinolysis as a complication of refeeding syndrome in a patient with anorexia nervosa. J Neuropsychiatry Clin Neurosci 2008; 20:371–373Link, Google Scholar
20. : Adverse endocrine and metabolic effects of psychotropic drugs: selective clinical review. CNS Drugs 2009; 23:1003–1021Crossref, Medline, Google Scholar
21. : Central pontine demyelinolysis following water intoxication in schizophrenia. Schizophr Res 2011; 125:300–301Crossref, Medline, Google Scholar
22. : Pontine and extrapontine osmotic myelinolysis after the syndrome of inappropriate secretion of antidiuretic hormone (SIADH) associated with fluoxetine: case report. Arq Neuropsiquiatr 2007; 65:858–864Crossref, Medline, Google Scholar
23. : Central pontine and extra-pontine myelinolysis: a complication of lithium toxicity in a pregnant woman. Clin Neurol Neurosurg 2008; 110:852–854Crossref, Medline, Google Scholar
24. : Antidepressants, thiazide diuretics, and alcoholism: central pontine myelinolysis waiting to happen? Br J Hosp Med 2009; 70:296–297Crossref, Google Scholar
25. : Outcome of central pontine and extrapontine myelinolysis (n=44). J Neurol 1999; 246:700–705Crossref, Medline, Google Scholar
26. : Benign type of central pontine myelinolysis in alcoholism: clinical, neuroradiological, and electrophysiological findings. J Neurol 2003; 250:1077–1083Crossref, Medline, Google Scholar
27. : Clinical and functional outcome and factors predicting prognosis in osmotic demyelination syndrome (central pontine and/or extrapontine myelinolysis) in 25 patients. J Neurol Neurosurg Psychiatry 2011; 82:326–331Crossref, Medline, Google Scholar
28. : Neuropsychiatric and neuropsychological manifestations of central pontine myelinolysis. Gen Hosp Psychiatry 1999; 21:296–302Crossref, Medline, Google Scholar
29. : Neuropsychological findings of extrapontine myelinolysis without central pontine myelinolysis. Behav Neurol 2007; 18:131–134Crossref, Medline, Google Scholar
30. : Cognitive and emotional dysfunction after central pontine myelinolysis. Behav Neurol 2003; 14:103–107Crossref, Medline, Google Scholar
31. : MRI findings in osmotic myelinolysis. Clin Radiol 2002; 57:800–806Crossref, Medline, Google Scholar
32. : Central pontine myelinolysis, an update. Neurol Res 2006; 28:360–366Crossref, Medline, Google Scholar
33. : AJR teaching file: symmetric demyelination. AJR Am J Roentgenol 2008; 191:S34–S36Crossref, Medline, Google Scholar
34. : Best cases from the AFIP: osmotic demyelination syndrome. Radiographics 2009; 29:933–938Crossref, Medline, Google Scholar
35. : Imaging evaluation of demyelinating processes of the central nervous system. Postgrad Med J 2010; 86:218–229Crossref, Medline, Google Scholar
36. : Differential diagnosis of T2 hyperintense brainstem lesions, part 2: Diffuse lesions. Semin Ultrasound CT MR 2010; 31:260–274Crossref, Medline, Google Scholar
37. : Diffusion magnetic resonance imaging may provide prognostic information in osmotic demyelination syndrome: report of a case. Acta Radiol 2006; 47:208–212Crossref, Medline, Google Scholar
38. : A case of central pontine and extrapontine myelinolysis with early hypermetabolism on 18FDG-PET scan. J Korean Med Sci 1998; 13:99–102Crossref, Medline, Google Scholar
39. : Magnetic resonance imaging of sequelae of central pontine myelinolysis in chronic alcohol abusers. Neuroradiology 2003; 45:877–880Crossref, Medline, Google Scholar
40. : Hypertension encephalopathy: isolated pons involvement mimicking central pontine myelinolysis. Korean J Radiol 2006; 7:218–219Crossref, Medline, Google Scholar
41. : Decreased diffusion in central pontine myelinolysis. AJNR Am J Neuroradiol 2001; 22:1476–1479Medline, Google Scholar
42. : Diffusion-weighted MR findings of central pontine and extrapontine myelinolysis. Acta Neurol Scand 2001; 104:385–388Crossref, Medline, Google Scholar
43. : Early diagnosis of central pontine myelinolysis with diffusion-weighted imaging. AJNR Am J Neuroradiol 2004; 25:210–213Medline, Google Scholar
44. : Central pontine myelinolysis after liver transplantation: MR diffusion spectroscopy and perfusion finding. Magn Reson Imaging 2006; 24:1395–1398Crossref, Medline, Google Scholar
45. : Brain water mobility decreases after astrocytic aquaporin-4 inhibition using RNA interference. J Cereb Blood Flow Metab 2011; 31:819–831Crossref, Medline, Google Scholar
46. : Normal diffusion-weighted MR imaging predicts a good prognosis in extrapontine myelinolysis-induced parkinsonism. Mov Disord 2009; 24:1701–1703Crossref, Medline, Google Scholar
47. : Decreased striatal dopamine transporter binding in a patient with extrapontine myelinolysis. Mov Disord 2003; 18:342–345Crossref, Medline, Google Scholar
48. : (99m) Tc-TRODAT-1 and (123)I-IBZM SPECT studies in a patient with extrapontine myelinolysis with parkinsonian features. Ann Nucl Med 2009; 23:409–412Crossref, Medline, Google Scholar
49. : Human cerebral osmolytes during chronic hyponatremia: a proton magnetic resonance spectroscopy study. J Clin Invest 1995; 95:788–793Crossref, Medline, Google Scholar
50. : N-acetylaspartate in the CNS: from neurodiagnostics to neurobiology. Prog Neurobiol 2007; 81:89–131Crossref, Medline, Google Scholar
51. : Magnetic resonance spectroscopy of the brain: review of metabolites and clinical applications. Clin Radiol 2009; 64:12–21Crossref, Medline, Google Scholar
52. : Management of hyponatraemia. Br J Hosp Med (London) 2011; 72:M22–M25Crossref, Medline, Google Scholar
53. : Puzzles in practice: central pontine myelinolysis (osmotic demyelination syndrome). Postgrad Med 2011; 123:191–194Crossref, Medline, Google Scholar
54. : Plasmapheresis improves the outcome of central pontine myelinolysis. Exp Neurol 2005; 252:734–735Crossref, Google Scholar
55. : Treatment of central pontine myelinolysis with plasmapheresis and immunoglobulins in liver transplant. Transpl Int 2008; 21:390–391Crossref, Medline, Google Scholar
56. : Plasmapheresis and intravenous immune globulin improve neurologic outcome of central pontine myelinolysis occurring post-orthotropic liver transplant. Ann Pharmacother 2011; (E-pub ahead of print)Crossref, Google Scholar