The Functional Neuroanatomy of Decision-Making
Abstract
Decision-making is a complex executive function that draws on past experience, present goals, and anticipation of outcome, and which is influenced by prevailing and predicted emotional tone and cultural context. Functional imaging investigations and focal lesion studies identify the orbitofrontal, anterior cingulate, and dorsolateral prefrontal cortices as critical to decision-making. The authors review the connections of these prefrontal regions with the neocortex, limbic system, basal ganglia, and cerebellum, highlight current ideas regarding the cognitive processes of decision-making that these networks subserve, and present a novel integrated neuroanatomical model for decision-making. Finally, clinical relevance of this circuitry is illustrated through a discussion of frontotemporal dementia, traumatic brain injury, and sociopathy.
The act of making a decision is an exercise in the assertion of the human will. Human lives are propelled forward or pulled backward by decisions that are made on a daily basis in both social and professional settings. The importance of this cognitive process is evidenced by the fact that approximately 40% of deaths result from decision-making deficits at the most basic level of self-regulation.1 Consequently, the human brain has evolved to make decisions on many different levels. The prefrontal cortex consists of three major regions, including the orbitofrontal cortex (OFC), anterior cingulate cortex (ACC), and dorsolateral prefrontal cortex (DLPFC), which interact with each other as well as with subcortical structures, such as the basal ganglia, thalamus, and cerebellum, to influence the decision-making process. The OFC has rich limbic connections and is critical in reward-based and emotional decision-making. The DLPFC and ACC facilitate intellectually effortful decisions dependent on working memory and reasoning. Whereas most of the literature has focused primarily on the role of supratentorial structures in decision-making, we offer a new, expanded synthesis, integrating the function of both cortical and subcortical brain regions. In this context, we will then focus on disease processes that target key anatomic systems, resulting in decision-making impairments, and we discuss the secondary impacts on society.
Human Decision-Making: Functional Neuroanatomy
Decision-making is a complex human behavior, dependent on the integrity of frontal networks. As noted, three frontal circuits have been associated with decision-making: 1) the OFC and limbic pathways, directed toward reward and affective-based decisions; 2) the DLPFC, specialized for integrating multiple sources of information; and 3) the ACC, important in sorting among conflicting options, as well as outcome-processing.2 The prefrontal cortex also has connections with striatal and other subcortical areas that influence the function of these cortical regions.
The Orbitofrontal Cortex and Related Structures
The OFC is critical for decisions dependent on incentive gain as well as the emotional experience associated with outcomes.2 The OFC is composed of four cyto-architectonic areas: Brodmann area (BA)11, anteriorly; BA13, posteriorly; BA14, medially; and BA47/12, laterally.3,4 The lateral OFC (area 47/12) receives and integrates visual information from the inferior temporal cortex, auditory information from secondary and tertiary auditory areas, somatosensory information from the secondary somatosensory and parietal cortex, and heteromodal inputs from the superior temporal cortex.5 The orbitofrontal cortex is functionally organized such that the medial portion monitors and decodes rewards, whereas the lateral portion evaluates punishment.6 Reward value for concrete, primary reinforcing factors, such as touch and taste, are encoded in the posterior OFC, whereas the value of more-complex, secondary reinforcing factors, such as money, are encoded in the anterior OFC.7
The OFC is located adjacent to the anterior cingulate cortex (ACC) and the frontal polar cortex;8 these three regions are jointly referred to as the ventromedial prefrontal cortex (VMPC) although the structures designated by this region may vary according to the literature. The OFC has cortico–cortical connections with other frontal structures involved in decision-making, including the DLPFC and ACC. Furthermore, the OFC is distinguished by strong bidirectional links with the anterior cingulate, insular, and medial temporal cortices, and with the amygdala and hippocampus.9 Networks between the OFC, temporal association cortex, amygdala, and lateral prefrontal cortex are largely responsible for emotional processing.9
The temporal cortex contains feed-forward projections to the amygdala, which has bidirectional, partially segregated, and highly specific connections with the OFC.10 The OFC has two distinct efferent projections to the amygdala, resulting in separate outcomes: 1) projections to the intercalated mass of the amygdala lead to disinhibition of autonomic structures within the hypothalamus (autonomic arousal); and 2) projections to the central nucleus of the amygdala lead to inhibition of hypothalamic structures (autonomic homeostasis).10
Patients with OFC lesions suffer from two types of deficits that ultimately affect decision-making. First, individuals fail to shift stimulus–response contingencies and suffer from impaired reward-processing. In other words, patients may fail to alter their decisions to a given stimulus despite a negative associated outcome. Second, patients are deficient in tasks requiring empathy or theory of mind (TOM), failing to process and recognize the emotions of others, and manifest impaired judgment in social contexts. Such limitations in social learning and emotional identification frequently lead to a clinical syndrome consisting of disinhibition, impulsivity, increased risk-taking behavior, and an inability to alter one’s behavior despite negative social consequences.
Evidence for the role of the OFC in emotion-based reward-processing comes from nonhuman primate investigations, human lesion studies, and neuroimaging. On a cellular level, OFC neurons fire during reward contingencies, particularly when monkeys anticipate a large reward as opposed to a small one.11 On the other hand, OFC neurons manifest a decreased firing rate when choices predict larger punishment.12 The firing of OFC neurons can further be influenced by whether or not the stimulus was presented previously, and by the immediacy of the reward.13
Previous investigators have studied the role of the OFC in human decision-making by administering a gambling task in frontal-lesion patients. Studies using the Iowa Gambling Task (IGT), an assessment of decision-making under varying conditions of risk, first established that patients with OFC lesions suffer from impaired decision-making.14 Also, the Cambridge Gambling Task (CGT), another test of decision-making, minimizes the amount of required learning and retrieval that may involve other brain regions, such as the DLPFC.15 Both tests address risk-taking behavior as manifested by an ability to moderate one’s betting strategies on the basis of experience (i.e., normal subjects would avoid placing large bets when the odds of winning are low). Although these studies have provided valuable insights into the role of these brain structures, they are also limited by their inclusion of subjects with diffuse frontal lesions (tumor, infarct, aneurysm, traumatic brain injury, and surgery for intractable epilepsy) that extend beyond the ventromedial prefrontal cortex into the dorsomedial PFC, dorsolateral PFC, temporal poles, and the basal forebrain.
Decision-making in VMPC patients is characterized by increased risk-taking behavior. Compared with normal and brain-damaged control subjects, VMPC patients have an increased predilection for choosing cards from disadvantageous decks during the IGT.16 A study of 11 subjects with chronic frontal-lobe injuries showed that patients with focal left-sided OFC and ventrolateral prefrontal cortex lesions were more likely to exhibit increased risk-taking behavior (rather than impulsivity) on a gambling task, as compared with patients with bilateral frontal and non-frontal injuries.17 VMPC patients also suffer from sympathetic dysfunction when confronted with high-stakes decisions. Over the course of the IGT, normal patients with risky decks develop an anticipatory skin conductance response (SCRs) 5 seconds before making card choices. Such anticipatory SCRs are absent in patients with VMPC lesions.18
The poor decision-making in this population is further characterized by an inability to adjust stimulus–reward contingencies. Patients with OFC lesions fail to modify their gambling strategy on the basis of feedback and continue to make the same gambling mistakes, ending up with greater net losses than control subjects. The mechanism behind recurrent maladaptive decisions in OFC patients may be deficient counterfactual thinking (comparing “what is” with “what could have been”), an important component of regret and subsequent learning. Also, these patients fail to experience negative emotions, despite being informed that they had suffered a gambling loss.19
Patients with VMPC lesions further exhibit deficient emotional processing: they fail to experience and recognize social emotions (compassion, shame, guilt) and possess a low threshold for anger and frustration.20,21 Despite preserved recognition of facial expression, OFC patients rate faces displaying negative emotional expressions as more approachable, compared with subjects with OFC-sparing frontal lesions and normal-control subjects.22
Such impairments in emotional processing may translate to patients’ taking an impersonal approach toward moral decision-making. One study subjected 6 bilateral VMPC patients, 12 brain-damaged controls with lesions sparing the VMPC, and 12 normal controls to high-conflict moral dilemmas that required balancing group welfare with the aversion of harming another individual (e.g., smothering one’s baby to save a group of people).23 Patients with VMPC lesions were more likely than both brain-damaged controls (lesions sparing VMPC) and normal-controls to endorse actions that resulted in harming an individual to save a group. Also, VMPC patients showed lower empathy, embarrassment, and guilt scores, according to the Iowa Rating Scare of Personality Change. The relevance of the VMPC in decision-making has further been confirmed by functional magnetic resonance imaging (fMRI) studies demonstrating activation of the VMPC with tasks requiring moral judgments; passive viewing of morally salient photos; and the elicitation of charity, fairness, or guilt.24–26
The clinical and experimental observations described above have led to the proposition of a VMPC-based neuro-moral network.27 This network may be functionally lateralized to the right hemisphere. Patients with right-sided, as opposed to left-sided, lesions of the VMPC, exhibit impaired life decision-making ability, as reflected by the maintenance of gainful employment, as well as IGT/CGT performance.16 The right VMPC is also activated with performance of the CGT on PET imaging.28
Although the role of the VMPC in emotional and reward processing has been extensively described in the literature, the OFC has connections with both the anterior insula and limbic system (hippocampus, amygdala) that are critical to the decision-making process. The amygdala, a structure activated by decreased perceived trustworthiness, facilitates social judgments about trusting others.29 Recent work has shown that the anterior insular cortex, a multifunctional structure involved in perceptual functions, speech, sensorimotor integration, body awareness, and emotional decision-making,30 serves a similar role in decision-making as the OFC. With connections to the ACC and VMPC, the anterior insula integrates autonomic information with emotional and motivational functions. Patients with insular lesions demonstrate similar impairments on the IGT as OFC patients, but these studies were limited in that subjects’ lesions extended into limbic and ventromedial prefrontal regions.31 Furthermore, patients with insular lesions have been described as demonstrating an “emotional bluntness toward risk.”32
The Dorsolateral Prefrontal Cortex (DLPFC) and Anterior Cingulate Cortex (ACC)
Decisions dependent on emotions and stimulus–response contingencies predominantly activate the OFC, but this structure interacts with other frontal circuits, such as the DLPFC and the ACC, through cortico–cortical connections, to facilitate decision-making on various levels.33
The DLPFC (BA 9 and 46) occupies the superior and lateral regions of the frontal lobes and is organized along a dorsal–ventral axis, with the dorsal DLPFC monitoring working memory and the ventral DLPFC encoding and retrieving information stored in posterior cortical-association regions.2,34 Similar to the OFC, the DLPFC has strong limbic connections as well as cortico–cortical connections throughout the temporal, parietal, and occipital cortices.34,35
Lesions of the DLPFC result in a frontal dysexecutive syndrome, in which patients exhibit impairments in planning, inhibitory control, strategy development, cognitive flexibility, and working memory.36 Goel and Grafman37 describe the case of “PF,” a previously successful architect who underwent surgery to remove a meningioma in the right DLPFC, and subsequently developed impaired problem-solving ability at work. Functional imaging studies show that the DLPFC is activated during impersonal dilemmas, suggesting a more calculated, emotionless approach toward decision-making.38,39 The DLPFC also appears to play an important role in legal decision-making. An fMRI study of 16 normal subjects, given scenarios describing varying degrees of responsibility for an act by a protagonist, showed increased right DLPFC activation when subjects assigned blame, whereas increased amygdala, medial PFC, and posterior cingulate cortex activity was associated with the magnitude of determined punishment.40
The ACC occupies the medial portion of the prefrontal cortex. This region has cortico–cortical connections to the OFC and DLPFC, as well as subcortical projections to the nucleus accumbens. The most anterior portion of the ACC, BA 25, has been implicated in depression,41 and may participate in linking decision-making to emotional tone.42 The ACC’s role in decision-making may be related to the modulation of other select prefrontal regions, such as the OFC and DLPFC. Studies have shown that particularly complex decisions involve the ACC, along with multiple brain structures, such as the OFC, DLPFC, and insular cortex.43,44 Furthermore, patients with bilateral OFC lesions with involvement of the ACC performed worse on tasks requiring evaluation and recognition of social cues than OFC subjects without ACC involvement.45
The ACC is recruited for highly ambiguous choices, such as in situations in which there are conflicting options and a high likelihood of error. Single-unit recording studies of cell populations in the macaque dorsal ACC during the performance of a reward-based decision task (choosing whether to push versus turn a handle for reward), have shown that these cells are five times more active during the interval between reward-reduction and the initiation of the newly-corrected movement.46 Also, the ACC participates in performance-optimization and evaluation by using previous reward experiences to guide choices. A case study of a patient with cortical dysplasia affecting the mediofrontal cortex demonstrated selective impairments in ambiguous decision-making pre- and post-surgically.47 fMRI studies have revealed ACC activation during periods of reduced reward or upon receiving negative feedback for incorrect answers.48
White Matter and Subcortical Projections
The function of the prefrontal regions is dependent not only on the cortical structures themselves, but also on white-matter pathways and subcortical structures, including the thalamus, basal ganglia, and cerebellum, with which the PFC is interconnected. Thus, a lesion involving any part of the network, whether it be neocortex, white-matter tracts, or deep subcortical structures, may lead to clinically relevant deficits in decision-making.
White-Matter Projections
Neurons within the OFC, DLPFC, and ACC conform to the general principle of organization of cerebral connections in that they give rise to five types of efferent projections: 1) association fibers; 2) striatal fibers; 3) commissural fibers and subcortical projections fibers to the 4) thalamus; and 5) the pontocerebellar system.49,50
Key decision-making structures, such as the OFC and temporal pole, are connected to their contralateral counterparts through the anterior commissure.51,52 The uncinate fasciculus, located within the anterior temporal region, is responsible for enabling emotional associations through the relaying of sensory information to the OFC.10 As mentioned previously, the anterior temporal cortex and amygdala both have feed-forward and feed-back projections to the OFC.
In addition to association fibers linking prefrontal regions and commissural fibers that enable projections to the contralateral hemisphere, prefrontal cortices have subcortical projections to the striatum, thalamus, cerebellum, and pons. The anterior limb of the internal capsule conveys fibers from the prefrontal cortex, rostral cingulate region, and supplementary motor area to the thalamus, hypothalamus, and basis pontis.51,52 Consequently, complex cognitive and behavioral syndromes affecting decision-making may result from lesions involving the anterior limb and genu of the internal capsule.53,54
Subcortical Structures
All prefrontal regions involved in decision-making send projections to the caudate nucleus/putamen through the subcallosal fasciculus of Muratoff or the external capsule.50,51 In turn, the basal ganglia relay this information to the thalamus, which sends feedback projections to the original cortical region.55 Lesions involving fronto-striatal connections may interfere with goal-oriented behaviors and potentially result in a “subcortical dementia,” consisting of bradyphrenia, forgetfulness, apathy, and depression.56,57 The OFC and ACC have projections to the ventral striatum,58 and, consequently, damage to this structure may lead to clinical presentations resembling those of VMPC patients, with symptoms of disinhibition, loss of reward-contingencies, and labile behaviors.59 Furthermore, bilateral pallidotomy performed to treat motor symptoms in Parkinson’s disease may lead to a clinical syndrome of disinhibition, apathy, poor judgment, and lack of insight.60
From the basal ganglia, information is relayed to the thalamus, which projects back to the neocortex. The mediodorsal nucleus is reciprocally interconnected with the OFC, DLPFC, amygdala, basal forebrain, olfactory, and entorrhinal cortex.61 Lesions involving this thalamic nucleus have resulted in apathy, abulia, and disinhibition.62
Finally, selected areas of the posterior lobe of the cerebellum (crus I and II of lobule VIIA, in particular) are engaged in high-level functions, such as working memory, linguistic processing, visual spatial analysis, and emotion regulation,63 and are reciprocally interconnected with neocortical association areas, including the prefrontal cortex in monkey64 and human.65 The cerebellar engagement in these higher-order functions is facilitated by topographically-organized feed-forward pro-jections from the cerebral cortex by way of the nuclei in the basis pontis66,67 and by feedback projections from topographically precise regions of the cerebellar deep nuclei (the dentate nucleus, in particular) to the cerebral-cortical association areas.68 The cerebellum may therefore be regarded as an integral node in the cortical–subcortical circuits linked to the prefrontal cortex, and likely plays a role in the modulation of prefrontal circuits and function.49
The cerebellar cognitive affective syndrome that results from lesions of the cognitive cerebellum in the posterior lobe is characterized by deficits in executive function and linguistic processing, in addition to visuospatial impairments and affective dysregulation.69 The affective component is particularly evident in patients with lesions involving the vermis and fastigial nucleus; these individuals exhibit disinhibition, loss of social boundaries, and impulsivity.69,70
A Model for Decision-Making
The decision-making process depends on interactions between the three prefrontal regions described above with subcortical structures that include the limbic system, basal ganglia, thalamus, cerebellum, and pons. In contrast to memory71 and language, a formal decision-making network has yet to be defined. Wallis has assigned the OFC, DLPFC, and ACC specific roles in the process of decision-making.13 According to this model, the OFC, through connections with the amygdala and limbic system, encodes the value of a reward outcome in relationship to a particular decision. The DLPFC then processes and utilizes this information in a top-down fashion to construct a specific plan for that reward outcome based on the OFC’s appraisal of reward value. Finally, the ACC serves to evaluate the likelihood of success for the plans generated by the DLPFC before the execution of the behavioral response. This model provides a rationale for previous observations that patients with extensive prefrontal lesions extending beyond the OFC perform worse on decision-making tasks such as the IGT.
Graybiel has proposed that components of the neocortical–basal ganglia loops are essential for learned actions to become habitual, and that abnormal activity within these loops is implicated in a range of clinical disorders related to action-compulsion, as occurs in obsessive-compulsive disorders and addiction behaviors.72 Inhibitory prefrontal projections to the basal ganglia may serve a self-regulating purpose, providing signals that direct behaviors away from immediate and toward distant rewards.13 In general, PFC activity is associated with long-term outcomes, whereas subcortical activity is associated with more immediate outcomes.
Heatherton and colleagues describe the prefrontal cortex as providing top-down control affecting the striatum and limbic cortices. The nucleus accumbens (NA) is a dopamine-activated region located within the striatum responsible for mediation of immediate reward, and it has been implicated in addiction. Rats with excitotoxic lesions involving this region demonstrate an increased predilection for immediate rewards and show difficulty learning instrumental responses using delayed reinforcement.73 The NA receives dopamine inputs from the ventral tegmental area (VTA) within the brainstem, and disruptions to this neurotransmitter’s activity may contribute to impulsive decision-making, as evidenced by disease states that include schizophrenia, amphetamine abuse, and Parkinson’s disease, particularly in the setting of dopamine agonists.74 The NA receives inhibitory inputs from the medial prefrontal cortex; cocaine users or smokers asked to inhibit their cravings to environmental cues manifest increased prefrontal activity on functional imaging.75 The prefrontal cortex also has inhibitory connections with the amygdala and limbic system (i.e., OFC, with inhibitory connection to the central nucleus of the amygdala), and compromise of this pathway has been implicated in mood disorders without obvious structural abnormalities. This model is supported by an FDG-PET study showing frontal hypoperfusion and limbic hyperperfusion in subjects classified as “affective murderers.”76 Ultimately, inhibitory projections between the prefrontal cortex and subcortical regions (striatum and limbic system) provide a balance between self-control and reward incentives, emotions, or attitudes.
The decision-making process depends on cortico–cortical and cortico–subcortical interactions such that all three prefrontal regions (OFC, ACC, and DLPFC) may be defined by the pattern of their connections. In other words, decision-making impairment may result from lesions not only involving the cortical regions, but also the limbic system, subcortical nuclei and white matter. The cortex is distinguished in that it maintains direct connections through associational fibers with other neocortical regions as well as the subcortical gray matter and limbic cortex.
On the basis of the prefrontal circuits and models described above, we conceptualize a novel decision-making network depicting the projections between neocortical and subcortical regions (Figure 1).
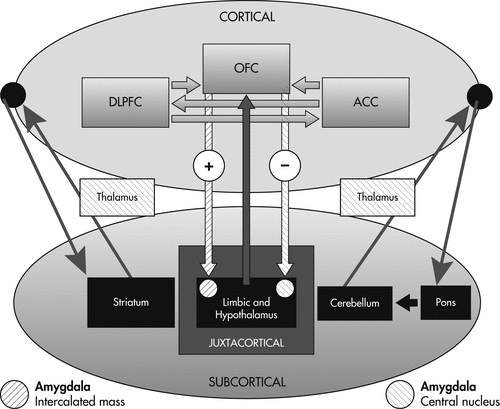
Clinical Correlations
Multiple disease processes result in selective lesions to the decision-making network and have provided useful insights into the functional anatomy of decision-making. Common lesions affecting decision-making structures include neurodegenerative disorders (behavioral-variant frontotemporal dementia), trauma (traumatic and surgical brain injury), and antisocial behavior (including sociopathy).
Behavioral-Variant Frontotemporal Dementia (bv-FTD)
Frontotemporal dementia is a chronic neurodegenerative condition resulting in early behavioral disinhibition, apathy, loss of sympathy, perseverative behavior, and/or hyperorality.34 Consequently, patients may engage in unsolicited sexual behavior, traffic violations, physical assaults, and paraphilias.77 Cognitive testing reveals prominent deficits in executive functioning, with relative sparing of memory and visuospatial ability. Neuroanatomically, bv-FTD results in selective degeneration of a network consisting of the frontopolar, insular, and anterior cingulate cortices, and striatum, with involvement of the DLPFC to a lesser extent.78,79 On the basis of the preclinical and cognitive-neuroscience studies described above, each of these structures has been shown to play a prominent role in decision-making. Furthermore, predominant atrophy of the right frontal network is most commonly associated with inappropriate social behaviors, loss of empathy, and loss of insight.80 Such observations have also been made in FTD patients with atrophy involving the right temporal pole.81
Mendez and colleagues79 studied the effect of impaired empathy on moral decision-making in bv-FTD. Twenty-six FTD and AD patients were given an inventory of moral knowledge and presented with two moral dilemmas. The first dilemma was impersonal, requiring the subject to choose whether to allow a runaway trolley to kill one person versus five, whereas the second dilemma was more personal, assessing whether the participant was willing to push a person from a footbridge to save five others. For this latter scenario, 57.7% of FTD subjects responded that they would indeed push a stranger onto the tracks to save the five workmen, versus 23.1% of AD subjects, and 19.2% of normal-controls. Despite intact moral knowledge, the bv-FTD patients lacked the ability for emotional identification with others and solved the moral dilemmas in an impersonal fashion. This observation may result from preservation of an intact, rational DLPFC in the setting of a dysfunctional OFC incapable of emotional processing. Such studies suggest that bv-FTD patients are unable to appreciate the social consequences of their actions because of an ability to emotionally identify with others.
The behavioral variant of frontotemporal dementia provides insight into both cognitive and behavioral components underlying the process of decision-making. Characterized by prominent behavioral disinhibition, this condition suggests that intact emotional processing is absolutely necessary for sound decision-making. Furthermore, these patients are deficient in frontally-based tasks such as multitasking, planning, and problem-solving, with relative preservation of verbal memory, thus making the argument that, from a cognitive standpoint, executive functioning, rather than memory, most significantly affects decision-making.
Traumatic and Surgical Brain Injury
There are 1.4 million new cases of traumatic brain injury (TBI) per year in the United States.82 Leading causes include motor vehicle accidents, falls, assaults, recreational accidents, and warfare.83 Functional impairments affecting comportment and decision-making have long been described in patients suffering from traumatic brain injury (TBI).
The OFC and temporal poles are particularly vulnerable to coup–contrecoup injury resulting from TBI. In addition to cortical contusions, TBI may further result in white-matter lesions through diffuse axonal and shearing injury. Consequently, intracranial lesions resulting from trauma are rarely circumscribed, often extending beyond prefrontal and temporal regions. Nevertheless, this condition does affect key frontal structures described in the model above and results in frontally-based deficits including attention, executive functioning, comportment, and memory retrieval.20,84
The case of EVR, described by Damasio and colleagues,85 is an illustrative description of the cognitive-behavioral consequences of surgical trauma to the prefrontal cortex. Before the resection of a bilateral orbitofrontal meningioma at the age of 35, EVR was described as a “role model and natural leader,” finishing college, rising through the ranks of a building firm, and maintaining a happy marriage. A life that had previously been characterized by sound decision-making suddenly underwent a catastrophic change first noticed after resection of the tumor. Simple decisions, such as choosing where to dine, became labored exercises in deliberation, lasting hours, as he considered the menu, the seating arrangement, and the atmosphere. He divorced his wife, remarrying 1 month after the separation, only to divorce his second wife within 2 years. He lost his job as his employers complained about his tardiness and disorganization. He eventually had to return home to live with his parents.
Decision-making in TBI patients is characterized by impulsivity and deficient planning. The Vietnam Head Injury Study (VHIS) was one of the earliest investigations to demonstrate that individuals with frontal lobe lesions exhibited increased impulsive, violent behavior, as compared with patients with nonfrontal injury as well as normal-controls.86 Violent behavior (fights or damaged property) occurred in 14% of patients with frontal lesions, versus 4% of normal-controls. Furthermore, the study found that aggression was associated with focal mediofrontal and orbitofrontal injury on CT. Patients with frontal lesions demonstrate impaired performance on tasks of executive functioning and decision-making. A study comparing chronic focal frontal lesions (N=42) with controls (N=38) showed that left ventrolateral lesions resulted in an increased number of incorrect responses to distracters, whereas right superior medial lesions (anterior cingulate cortex, supplementary motor cortex), and dorsolateral PFC resulted in fewer correct responses and delayed reaction time on the Modified Stroop task.84
TBI patients may show impairments in decision-making even in the absence of obvious structural lesions on MRI. More sensitive imaging techniques still indicate dysfunction of prefrontal and associated subcortical structures. Diffusion tensor imaging (DTI) is a technique that measures microstructural gray- and white-matter integrity, based on the diffusion of water molecules within brain tissue. One study of TBI subjects without obvious MRI structural abnormalities subjected both patients and controls to the CGT and correlated impaired performance with associated anatomical structures on DTI.87 TBI patients demonstrated greater impulsivity than control subjects on the CGT. Also, increased impulsivity on the CGT correlated with DTI abnormalities within the OFC, insula, and caudate; impaired risk-adjustment (i.e., learning from mistakes) correlated with DTI abnormalities in the thalamus, dorsal striatum, and left caudate; and problems with rational choice were associated with DTI abnormalities of the bilateral dorsolateral prefrontal cortices, superior frontal gyrus, right ventrolateral prefrontal cortex, dorsal/ventral striatum, and left caudate.
Studies of preadolescent patients with focal damage to the prefrontal cortex have demonstrated a more severe tendency for behavior to be guided by immediate consequences and demonstrate inadequate emotional responses with little recovery of function over time.88 Price et al.89 described two patients with early bilateral frontal lobe pathology. The first patient was a 31-year-old man who had suffered a subdural hematoma when he was 7 days old that required surgical evacuation, leading to serious behavioral problems recognized by the age of 8. He failed to respond to parental discipline and later was imprisoned eight times on charges of assault, forgery, grand larceny, drug involvement, and lewd behavior. The other patient was a 26-year-old woman who had suffered a frontal hematoma secondary to a car accident sustained at the age of 4. The patient later became notorious for her sexual promiscuity and bravado, frequently engaging in drug use. She displayed inappropriate and negligent care of her infant. She would also make poor decisions, such as wandering alone through a local cemetery where she was raped on two different occasions by the same man.
Sociopathy and Criminality
Although it is difficult for the neurologist to determine whether a criminal act stems from cognitive, behavioral, psychiatric, or environmental factors, the individual guilty of the crime nevertheless has to make a risk-versus-benefit decision upon performance of the illicit act. In contrast to TBI and bv-FTD, individuals with sociopathy do not uniformly demonstrate structural frontal lesions with conventional neuroimaging.
Decision-making in this population appears to be characterized by impulsivity. A study of 81 criminals that included violent, drug, and sex offenders showed that individuals charged with assault or murder focused on immediate outcomes on the Iowa Gambling Task, similar to patients with OFC lesions.91 Like OFC patients, patients with sociopathy experience difficulty with reversal-learning and exhibit decision-making characterized by high risk-taking and impulsivity.92 They further fail to appreciate suffering in other individuals, with reduced recognition of and reaction to distress in others, as manifested by sad or fearful expressions, and show reduced autonomic responses in heart rate, skin conductance, or respirations to the distress of others.93
Studies have shown a high incidence of frontal network disorders within the criminal population.94 Of 31 patients awaiting trial or sentenced for murder, undergoing neurological examination, neuropsychological testing, EEG, and neuroimaging,95 64.5% showed frontal dysfunction, whereas 29% were found to have a temporal lobe abnormality.95 Studies on adult and juvenile death-row inmates show a high rate of head injury in this population, and one hypothesis is that these individuals have suffered previous injuries to the ventromedial cortex akin to the acquired sociopathy associated with TBI.96
Neuroimaging of subjects with either a history of violence or antisocial personality disorder (ASPD) have further implicated the prefrontal cortex. A meta-analysis across 43 independent structural and functional imaging studies in antisocial individuals showed the right OFC, right ACC, and the left DLPFC to be the most affected regions in this population.97 A PET study involving 41 subjects charged with murder or manslaughter showed statistically significant bilateral prefrontal metabolic hypometabolism during a frontal lobe activation task, as compared with controls.98 A subsequent PET study from the same group showed frontal hypometabolism and limbic hypermetabolism in 9 “affective” murderers, as compared with 41 controls, suggesting a component of limbic disinhibition due to frontal dysfunction in this population.98 PET and SPECT imaging of patients with ASPD have shown decreased glucose metabolism in the orbitofrontal, left anterior frontal, anterior medial frontal, left angular gyrus, and temporal cortex, as compared with controls.99,100,101
Conclusion
We present a novel, comprehensive model for decision-making, integrating the roles of the prefrontal cortex, limbic system, striatum, thalamus, and cerebellum in decision-making. Based on our paradigm, a cortical area is defined by its connections: any lesion affecting any part of the decision-making network, whether it be cortical or subcortical, may compromise the process of decision-making.
Similar to complex cognitive processes such as memory and language, the execution of a decision is a human behavior necessary for the maintenance of mental health, physical health, and the achievement of personal goals. Depending on context and complexity, decisions derive from a dynamic, reciprocal, multidimensional process based on specific interactions between prefrontal and subcortical structures.
Disruption to the decision-making process has profound implications both for the affected individual as well as for society. Individuals who are better able to self-regulate demonstrate improved relationships, increased job success, and better mental health, with decreased likelihood of developing substance-abuse problems. The cognitive process of decision-making is arguably no less important than memory, yet understanding the neurobiology of decision-making has been limited by the absence of investigational tools, complexity of the process, and the broad extent of brain regions involved. Fortunately, anatomical tract-tracing in nonhuman primates, comprehensive lesion studies in afflicted patients, and imaging techniques have yielded complementary and converging evidence for a functional neuroanatomy of decision-making. Increasing collaboration between the neuroscience, neurological, and psychiatric communities should yield further discoveries relevant to the diagnosis and treatment of disorders involving impaired decision-making.
1 : Shattuck Lecture: We can do better: improving the health of the American people. N Engl J Med 2007; 357:1221–1228 Crossref, Medline, Google Scholar
2 : Contributions of the prefrontal cortex to the neural basis of human decision-making. Neurosci Biobehav Rev 2002; 26:631–664 Crossref, Medline, Google Scholar
3 : Architectonic subdivision of the orbital and medial prefrontal cortex in the macaque monkey. J Comp Neurol 1994; 346:366–402 Crossref, Medline, Google Scholar
4 : Comparative cytoarchitectonic analysis of the human and the macaque ventrolateral prefrontal cortex and corticocortical connection patterns in the monkey. Eur J Neurosci 2002; 16:291–310 Crossref, Medline, Google Scholar
5 : Sensory and premotor connections of the orbital and medial prefrontal cortex of macaque monkeys. J Comp Neurol 1995; 363:642–664 Crossref, Medline, Google Scholar
6 : Abstract reward and punishment representations in the human orbitofrontal cortex. Nat Neurosci 2001; 4:95–102 Crossref, Medline, Google Scholar
7 : The functions of the orbitofrontal cortex. Brain Cogn 2004; 55:11–29 Crossref, Medline, Google Scholar
8 : Architectonic subdivision of the human orbital and medial prefrontal cortex. J Comp Neurol 2003; 460:425–449 Crossref, Medline, Google Scholar
9 : Flow of information for emotions through temporal and orbitofrontal pathways. J Anat 2007; 211:237–249 Crossref, Medline, Google Scholar
10 : Pathways for emotion: interactions of prefrontal and anterior temporal pathways in the amygdala of the rhesus monkey. Neuroscience 2002; 115:1261–1279 Crossref, Medline, Google Scholar
11 : Neuronal activity in primate orbitofrontal cortex reflects the value of time. J Neurophysiol 2005; 94:2457–2471 Crossref, Medline, Google Scholar
12 : Neuronal activity related to reward value and motivation in primate frontal cortex. Science 2004; 304:307–310 Crossref, Medline, Google Scholar
13 : Orbitofrontal cortex and its contribution to decision-making. Annu Rev Neurosci 2007; 30:31–56 Crossref, Medline, Google Scholar
14 : Insensitivity to future consequences following damage to human prefrontal cortex. Cognition 1994; 50:7–15 Crossref, Medline, Google Scholar
15 : Decision-making processes following damage to the prefrontal cortex. Brain 2002; 125:624–639 Crossref, Medline, Google Scholar
16 : The role of emotion in decision-making: evidence from neurological patients with orbitofrontal damage. Brain Cogn 2004; 55:30–40 Crossref, Medline, Google Scholar
17 : Impulsivity and risk-taking behavior in focal frontal lobe lesions. Neuropsychologia 2008; 46:213–223 Crossref, Medline, Google Scholar
18 : Failure to respond autonomically to anticipated future outcomes following damage to prefrontal cortex. Cereb Cortex 1996; 6:215–225 Crossref, Medline, Google Scholar
19 : The involvement of the orbitofrontal cortex in the experience of regret. Science 2004; 304:1167–1170 Crossref, Medline, Google Scholar
20 : Individuals with sociopathic behavior caused by frontal damage fail to respond autonomically to social stimuli. Behav Brain Res 1990; 41:81–94 Crossref, Medline, Google Scholar
21 : The regulatory function of self-conscious emotion: insights from patients with orbitofrontal damage. J Pers Soc Psychol 2003; 85:594–604 Crossref, Medline, Google Scholar
22 : Orbitofrontal cortex lesions result in abnormal social judgements to emotional faces. Neuropsychologia 2010; 48:2182–2187 Crossref, Medline, Google Scholar
23 : Damage to the prefrontal cortex increases utilitarian moral judgements. Nature 2007; 446:908–911 Crossref, Medline, Google Scholar
24 : Moral judgments, emotions and the utilitarian brain. Trends Cogn Sci 2007; 11:319–321 Crossref, Medline, Google Scholar
25 : Neural correlates of regulating negative emotions related to moral violations. Neuroimage 2006; 30:313–324 Crossref, Medline, Google Scholar
26 : The functional architecture of human empathy. Behav Cogn Neurosci Rev 2004; 3:71–100 Crossref, Medline, Google Scholar
27 : The neurobiology of moral behavior: review and neuropsychiatric implications. CNS Spectr 2009; 14:608–620 Crossref, Medline, Google Scholar
28 : Choosing between small, likely rewards and large, unlikely rewards activates inferior and orbital prefrontal cortex. J Neurosci 1999; 19:9029–9038 Crossref, Medline, Google Scholar
29 : The human amygdala in social judgment. Nature 1998; 393:470–474 Crossref, Medline, Google Scholar
30 Jones CL, Ward J, Critchley HD: The neuropsychological impact of insular cortex lesions. J Neurol Neurosurg Psychiatry 81:611–618Google Scholar
31 : Differential effects of insular and ventromedial prefrontal cortex lesions on risky decision-making. Brain 2008; 131:1311–1322 Crossref, Medline, Google Scholar
32 : The effects of insula damage on decision-making for risky gains and losses. Soc Neurosci 2009; 4:347–358 Crossref, Medline, Google Scholar
33 : Complementary roles of prefrontal cortical regions in cognition, memory, and emotion in primates. Adv Neurol 2000; 84:87–110 Medline, Google Scholar
34 : The Human Frontal Lobes, 2nd Edition. New York, Guilford, 2007 Google Scholar
35 : Comparison of prefrontal architecture and connections. Philos Trans R Soc Lond B Biol Sci 1996; 351:1423–1432 Crossref, Medline, Google Scholar
36 : Social and emotional decision-making following frontal lobe injury. Neurocase 2004; 10:398–403 Crossref, Medline, Google Scholar
37 : Role of the right prefrontal cortex in ill-structured planning. Cogn Neuropsychol 2000; 17:415–436 Crossref, Medline, Google Scholar
38 : Why are VMPFC patients more utilitarian? a dual-process theory of moral judgment explains. Trends Cogn Sci 2007; 11:322–323, author reply 323–324 Crossref, Medline, Google Scholar
39 : Individual differences in moral judgment competence influence neural correlates of socio-normative judgments. Soc Cogn Affect Neurosci 2008; 3:33–46 Crossref, Medline, Google Scholar
40 : The neural correlates of third-party punishment. Neuron 2008; 60:930–940 Crossref, Medline, Google Scholar
41 : Deep brain stimulation for treatment-resistant depression. Neuron 2005; 45:651–660 Crossref, Medline, Google Scholar
42 : Impaired flexible decision-making in Major Depressive Disorder. J Affect Disord 2010; 124:207–210 Crossref, Medline, Google Scholar
43 : Neural systems responding to degrees of uncertainty in human decision-making. Science 2005; 310:1680–1683 Crossref, Medline, Google Scholar
44 : The neural basis of financial risk-taking. Neuron 2005; 47:763–770 Crossref, Medline, Google Scholar
45 : Changes in emotion after circumscribed surgical lesions of the orbitofrontal and cingulate cortices. Brain 2003; 126:1691–1712 Crossref, Medline, Google Scholar
46 : Role for cingulate motor area cells in voluntary movement selection based on reward. Science 1998; 282:1335–1338 Crossref, Medline, Google Scholar
47 Labudda K, Brand M, Mertens M, et al: Alterations of decision-making and underlying neural correlates after resection of a mediofrontal cortical dysplasia: a single case study. Neurocase 2010; 16:59–73Google Scholar
48 : Error monitoring using external feedback: specific roles of the habenular complex, the reward system, and the cingulate motor area revealed by functional magnetic resonance imaging. J Neurosci 2003; 23:4308–4314 Crossref, Medline, Google Scholar
49 : Disconnection syndromes of basal ganglia, thalamus, and cerebrocerebellar systems. Cortex 2008; 44:1037–1066 Crossref, Medline, Google Scholar
50 Schmahmann JDPandya D (ed): Fiber Pathways of the Brain. New York, Oxford University Press, 2006 Crossref, Google Scholar
51 : Cerebral white matter: neuroanatomy, clinical neurology, and neurobehavioral correlates. Ann N Y Acad Sci 2008; 1142:266–309 Crossref, Medline, Google Scholar
52 : The cerebrocerebellar system. Int Rev Neurobiol 1997; 41:31–60 Crossref, Medline, Google Scholar
53 : Confusion and memory loss from capsular genu infarction: a thalamocortical disconnection syndrome? Neurology 1992; 42:1966–1979 Crossref, Medline, Google Scholar
54 : Extensive metabolic and neuropsychological abnormalities associated with discrete infarction of the genu of the internal capsule. J Neurol Neurosurg Psychiatry 2001; 71:658–662 Crossref, Medline, Google Scholar
55 : Limbic connections of the orbital and medial prefrontal cortex in macaque monkeys. J Comp Neurol 1995; 363:615–641 Crossref, Medline, Google Scholar
56 : The “subcortical dementia” of progressive supranuclear palsy. J Neurol Neurosurg Psychiatry 1974; 37:121–130 Crossref, Medline, Google Scholar
57 : Reward-related responses in the human striatum. Ann N Y Acad Sci 2007; 1104:70–88 Crossref, Medline, Google Scholar
58 : Corticostriatal connections of the superior temporal region in rhesus monkeys. J Comp Neurol 1998; 399:384–402 Crossref, Medline, Google Scholar
59 : Specialized elements of orbitofrontal cortex in primates. Ann N Y Acad Sci 2007; 1121:10–32 Crossref, Medline, Google Scholar
60 : Bilateral contemporaneous posteroventral pallidotomy for the treatment of Parkinson’s disease: neuropsychological and neurological side effects: report of four cases and review of the literature. J Neurosurg 1999; 91:313–321 Crossref, Medline, Google Scholar
61 : The primate mediodorsal (MD) nucleus and its projection to the frontal lobe. J Comp Neurol 1985; 242:535–560 Crossref, Medline, Google Scholar
62 : Thalamic infarcts: clinical syndromes, etiology, and prognosis. Neurology 1988; 38:837–848 Crossref, Medline, Google Scholar
63 : Functional topography in the human cerebellum: a meta-analysis of neuroimaging studies. Neuroimage 2009; 44:489–501 Crossref, Medline, Google Scholar
64 : Cerebellar loops with motor cortex and prefrontal cortex of a nonhuman primate. J Neurosci 2003; 23:8432–8444 Crossref, Medline, Google Scholar
65 : Distinct and overlapping functional zones in the cerebellum defined by resting state functional connectivity. Cereb Cortex 2010; 20:953–965 Crossref, Medline, Google Scholar
66 : Anatomic organization of the basilar pontine projections from prefrontal cortices in rhesus monkey. J Neurosci 1997; 17:438–458 Crossref, Medline, Google Scholar
67 : Prefrontal cortex projections to the basilar pons in rhesus monkey: implications for the cerebellar contribution to higher function. Neurosci Lett 1995; 199:175–178 Crossref, Medline, Google Scholar
68 : Cerebellar projections to the prefrontal cortex of the primate. J Neurosci 2001; 21:700–712 Crossref, Medline, Google Scholar
69 : The cerebellar cognitive affective syndrome. Brain 1998; 121:561–579 Crossref, Medline, Google Scholar
70 : Neuropsychological consequences of cerebellar tumour resection in children: cerebellar cognitive affective syndrome in a paediatric population. Brain 2000; 123:1041–1050 Crossref, Medline, Google Scholar
71 : Molecular, structural, and functional characterization of Alzheimer’s disease: evidence for a relationship between default activity, amyloid, and memory. J Neurosci 2005; 25:7709–7717 Crossref, Medline, Google Scholar
72 : Habits, rituals, and the evaluative brain. Annu Rev Neurosci 2008; 31:359–387 Crossref, Medline, Google Scholar
73 : Limbic corticostriatal systems and delayed reinforcement. Ann N Y Acad Sci 2004; 1021:33–50 Crossref, Medline, Google Scholar
74 : Cortico-limbic-striatal circuits subserving different forms of cost-benefit decision making. Cogn Affect Behav Neurosci 2008; 8:375–389 Crossref, Medline, Google Scholar
75 : Cognitive control of drug craving inhibits brain reward regions in cocaine abusers. Neuroimage 2010; 49:2536–2543 Crossref, Medline, Google Scholar
76 : Prefrontal glucose deficits in murderers lacking psychosocial deprivation. Neuropsychiatry Neuropsychol Behav Neurol 1998; 11:1–7 Medline, Google Scholar
77 : Acquired sociopathy and frontotemporal dementia. Dement Geriatr Cogn Disord 2005; 20:99–104 Crossref, Medline, Google Scholar
78 : Neurodegenerative diseases target large-scale human brain networks. Neuron 2009; 62:42–52 Crossref, Medline, Google Scholar
79 : An investigation of moral judgement in frontotemporal dementia. Cogn Behav Neurol 2005; 18:193–197 Crossref, Medline, Google Scholar
80 : Progressive right frontotemporal degeneration: clinical, neuropsychological, and SPECT characteristics. Dementia 1993; 4:204–213 Medline, Google Scholar
81 : Social conceptual impairments in frontotemporal lobar degeneration with right anterior temporal hypometabolism. Brain 2009; 132:604–616 Crossref, Medline, Google Scholar
82 2010; http://www.cdc.gov/ncipc/pub-res/TBI_in_US_04/TBI_ED.htm; accessed June 2010Google Scholar
83 : Is a picture worth a thousand words? neuroimaging in the courtroom. Am J Law Med 2007; 33:239–269 Crossref, Medline, Google Scholar
84 : Regional frontal injuries cause distinct impairments in cognitive control. Neurology 2007; 68:1515–1523 Crossref, Medline, Google Scholar
85 : Severe disturbance of higher cognition after bilateral frontal lobe ablation: patient EVR. Neurology 1985; 35:1731–1741 Crossref, Medline, Google Scholar
86 : Frontal lobe injuries, violence, and aggression: a report of the Vietnam Head Injury Study. Neurology 1996; 46:1231–1238 Crossref, Medline, Google Scholar
87 : Parcellating the neuroanatomical basis of impaired decision-making in traumatic brain injury. Brain 2011; 134:759–768 Crossref, Medline, Google Scholar
88 : Disturbances of emotion regulation after focal brain lesions. Int Rev Neurobiol 2004; 62:159–193 Crossref, Medline, Google Scholar
89 : The comportmental learning disabilities of early frontal lobe damage. Brain 1990; 113:1383–1393 Crossref, Medline, Google Scholar
90 : Neurocognitive deficits related to poor decision making in people behind bars. Psychon Bull Rev 2008; 15:44–51 Crossref, Medline, Google Scholar
91 : Prefrontal and medial temporal correlates of repetitive violence to self and others. Biol Psychiatry 2000; 47:928–934 Crossref, Medline, Google Scholar
92 : The psychopath as observer: emotion and attention in picture processing. J Abnorm Psychol 2000; 109:373–385 Crossref, Medline, Google Scholar
93 : Neuropsychiatry of frontal lobe dysfunction in violent and criminal behaviour: a critical review. J Neurol Neurosurg Psychiatry 2001; 71:720–726 Crossref, Medline, Google Scholar
94 : Neurologic abnormalities in murderers. Neurology 1995; 45:1641–1647 Crossref, Medline, Google Scholar
95 : Neuropsychiatric, psychoeducational, and family characteristics of 14 juveniles condemned to death in the United States. Am J Psychiatry 1988; 145:584–589 Crossref, Medline, Google Scholar
96 : Prefrontal structural and functional brain imaging findings in antisocial, violent, and psychopathic individuals: a meta-analysis. Psychiatry Res 2009; 174:81–88 Crossref, Medline, Google Scholar
97 : Brain abnormalities in murderers indicated by positron emission tomography. Biol Psychiatry 1997; 42:495–508 Crossref, Medline, Google Scholar
98 : Reduced prefrontal and increased subcortical brain functioning assessed using positron emission tomography in predatory and affective murderers. Behav Sci Law 1998; 16:319–332 Crossref, Medline, Google Scholar
99 : Single photon emission computerised tomography in chronic alcoholism: antisocial personality disorder may be associated with decreased frontal perfusion. Br J Psychiatry 1996; 169:348–354 Crossref, Medline, Google Scholar
100 : Reduced regional cerebral blood flow in non-psychotic violent offenders. Psychiatry Res 2000; 98:29–41 Crossref, Medline, Google Scholar
101 : Risky decision-making and the anterior cingulate cortex in abstinent drug abusers and nonusers. Brain Res Cogn Brain Res 2005; 23:119–136 Crossref, Medline, Google Scholar