Dissociation, Hemispheric Asymmetry, and Dysfunction of Hemispheric Interaction: A Transcranial Magnetic Stimulation Approach
Abstract
The authors investigated the hypothesis that dissociation may represent a functional dysconnectivity syndrome using a transcranial magnetic stimulation (TMS) approach. Transcranial magnetic stimulation investigations that included motor thresholds and the transcallosal conduction time (TCT) reflecting the interhemispheric transfer were performed in 74 right-handed students. All subjects completed the Dissociative Experience Scale. The high dissociators had a significantly lower left hemispheric excitability than right hemispheric excitability. They also had a significantly shorter TCT from the left to the right hemisphere than did the low dissociators. These results suggest that the neural basis of dissociation may involve a cortical asymmetry with a left hemispheric superiority or, alternatively, a lack of right hemispheric integration.
Dissociation is characterized by a “disruption in the usually integrated functions of consciousness, memory, identity, or perception of the environment.”1 Its clinical manifestations include amnesia for autobiographical information, depersonalization and derealization, and identity disturbances, which are core features of the dissociative disorders. Moreover, dissociative symptoms also play a prominent role as response to traumatic stress and in posttraumatic stress disorder (PTSD).2–4 Despite the broad clinical and theoretical interest in dissociation, its neurobiological basis is far from understood.5 Particularly, neurophysiological models have been given little attention,6 although it has been assumed that dissociation may represent a functional dysconnectivity syndrome.5 More specifically, it has been suggested that a dysfunction of hemispheric interaction might be a predisposition to dissociative psychopathology.6,7 Empirical evidence for this hypothesis has originated from various sources. A neurophysiological study of hypnotic and dissociative states indicated lateralized shifts in electroencephalogram (EEG) frequency and evoked potential amplitudes.8 A shift in hemispheric dominance was assumed as a possible explanation for the changes in handedness in dissociative identity disorders.9 In epileptic patients, dissociative-like phenomena were considered to reflect ictally dependent shifts in relative hemispheric dominance.10 Additionally, it was argued that a hemispheric imbalance may be relevant to the pathophysiology of PTSD.11 This is consistent with EEG and evoked potential studies in PTSD patients12,13 as well as with functional imaging investigations.14 Finally, developmental traumatology research15 indicated a negative correlation between dissociative psychopathology and the size of the corpus callosum in maltreated children. In sum, several lines of evidence support the hypothesis that a deviance in cerebral lateral organization and an altered interhemispheric processing of sensorimotor and cognitive information, which has been referred to as functional commissurotomy,5 may play a crucial role in dissociation.
Transcranial magnetic stimulation (TMS), a noninvasive, painless, and safe method of neuropsychiatry,16 allows the investigator to assess the cortical excitability and its interhemispheric asymmetry by means of the motor threshold required to induce a motor-evoked potential (MEP).17 Transcranial magnetic stimulation can also be applied to study the function of the corpus callosum (CC).18,19 The CC represents the largest connection between the cerebral hemispheres and plays a major role in interhemispheric transfer and integration of sensorimotor and cognitive information.20 The TMS approach to investigate transcallosal pathways is based on the observation that focal magnetic stimulation over the motor cortex of one hemisphere suppresses ongoing voluntary electromyographic (EMG) activity originating from the contralateral motor cortex, and this inhibition is mediated via the CC.18,19 The transcallosal inhibition (TI) and the transcallosal conduction time (TCT) reflect the interhemispheric transfer. In patients with abnormalities or cerebrovascular lesions of the CC, the TI was found to be abolished or delayed.19,21 In schizophrenia patients, the TCT and the duration of the TI were significantly prolonged as compared with healthy subjects.22
Taking the above into consideration, our explorative study, in which TMS was utilized, aimed to test the following hypotheses: 1) dissociative psychopathology is related to an interhemispheric cortical asymmetry as revealed by differences in MEP thresholds; 2) dissociation is linked to a dysfunction of hemispheric interaction as revealed by an altered TI. Because several TMS investigations23,24 indicated a functional hemispheric asymmetry between right- and left-handed individuals, we included only right-handed participants. Another reason for this procedure is the observation that handedness might be a predisposing factor for dissociative experiences.7
METHOD
Participants and Procedure
A random sample of students from our university was screened for the selection criteria of this study. Exclusion criteria were younger than 18 years of age, current or past psychiatric treatment (either inpatient or outpatient), current medication with psychopharmacological drugs, history of seizures or any other disorder of the central nervous system, and left handedness. A total of 74 students met the inclusion criteria. After complete description of the study, which was approved by the local ethical committee, all subjects gave written, informed consent to participate. The mean age of the participants was 22.4 years (SD=3.0, range=18–34), and there was an almost equal gender distribution, with 36 (49%) female and 38 (51%) male students. All participants underwent the electrophysiological investigation, as described below, and then completed the self-report measures.
TMS Procedure
We used a KEYPOINT commercial amplifier (Medtronic Dantec, Skovlunde, Denmark) with a band pass of 10–10000 Hz. Electromyographic recordings were made bilaterally with surface electrodes in tendon-belly arrangement from the first dorsal interosseus muscle (FDI). Transcranial magnetic stimulation was performed using a Maglite Stimulator (2-T version; Medtronic Dantec, Skovlunde, Denmark).
Motor thresholds of the FDI were evaluated using a standard round coil (outer diameter 13 cm) centered over the vertex, with the current flowing anticlockwise (as viewed from above) for stimulation of the left hemisphere and clockwise for the right hemisphere, as described elsewhere.23–25 Motor-evoked potential thresholds were determined in relaxed muscles on either side according to the International Federation of Clinical Neurophysiology recommendations26 by changing the stimulator intensity at 1% steps until there was a minimal motor response (>50 μV) in five out of 10 trials. This intensity was defined as the motor threshold and is expressed as percent of the maximum stimulator output.
Focal TMS of the motor cortex of each hemisphere was performed consecutively with a figure of eight-shaped coil (outside diameter of half-coil 9 cm). The coil center was placed over the hand area of the motor cortex, with the handle of the coil pointing backwards (current flow in posterior-anterior direction). The optimal stimulation point for producing maximal hand motor responses was determined individually for each subject.
Cortical stimulation was performed with a stimulus intensity of 75% of the maximum stimulator output during maximal voluntary contraction of the FDI. Stimuli were applied at a frequency of 0.2 Hz. Subjects were asked to maintain a maximal activation of their ipsilateral FDI before and during the stimulation and to relax their hand muscles afterwards in order to prevent fatigue. Twenty responses in each FDI ipsilateral and contralateral to focal TMS were recorded and averaged. In muscles contralateral to the hemisphere, stimulated corticomotor conduction time (CMCT) was determined. In FDI ipsilateral to stimulation, we measured the onset latency (LTI) and the duration (DTI) of transcallosal inhibition. The CMCT is the interval between the transcranial magnetic stimulus and the beginning of the EMG response in the contralateral FDI. The LTI was defined as the interval between the stimulation and the suppression of the tonic EMG activity in the ipsilateral FDI. The DTI was measured from the onset of EMG activity suppression to its recurrence. The TCT was calculated by subtracting the CMCT of one FDI from the LTI of the same FDI (e.g., LTI right FDI—CMCT right FDI=TCT right to left hemisphere). The principle of TMS and the method of determining the different response parameters are illustrated in Fig. 1.
Psychometric Instruments
The Dissociative Experiences Scale (DES) is a 28-item, self-administered inventory to measure the frequency of dissociative experiences.27 A cutoff score of 30 or above was recommended to differentiate between high and low dissociators.28 The DES is an internationally, well-established, highly valid and reliable questionnaire.29 The psychometric properties of the German version were almost identical to the original version.30 Handedness was determined by the Edinburgh Inventory.31
Statistical Analysis
Data analyses were computed using SPSS (version 10.0). The results are presented as absolute numbers and corresponding percentages or as group means and standard deviations. Motor-evoked potential thresholds are expressed as mean percentages of the stimulator output. The chi-square test was used to compare the male-to-female ratio. We applied nonparametric procedures because we did not assume a normal distribution of the data due to the relatively small number of participants. Within group differences (i.e., MEP thresholds of both sides) were analyzed by means of the Wilcoxon matched-pairs test. For between group differences (i.e., high and low dissociators) we used the Mann-Whitney U test. This was followed by a linear regression with the DES score as dependent variable and those neurophysiological parameters as independent variables, which significantly differed between the high and low dissociators. Significance level was set at P<0.05.
RESULTS
Dissociative Experiences Scale scores ranged from 1.1 to 58.9, with a median of 11.8 and mean of 14.8 (SD=11.4). Of the 74 participants, eight (11%) scored 30 or above. Among these high dissociators, four (50%) were female. There was no significant difference in the gender distribution between the high and low dissociators (χ2=0.01, df=1, P=0.935). High and low scoring subjects did not significantly differ with respect to age (high dissociators mean age=22.3 years, SD=2.0; low dissociators mean=22.4 years, SD=3.1) (U=242.0, P=0.699).
For the entire sample, there was no difference in cortex excitability as measured by the motor thresholds, which was 40.9% (SD=6.8%) for the right FDI and 41.1% (SD=6.8%) for the left FDI (z=−0.52, P=0.604). However, in the group of high dissociating students, the motor threshold for the right FDI was significantly higher (44.3%, SD=9.3%) than that for the left FDI (41.4%, SD=8.5%) (z=−2.32, P=0.020) indicating a lower left hemispheric excitability. In the low dissociators, the right motor threshold (mean=40.5%, SD= 6.5%) did not significantly differ from the left side (mean=41.1%, SD=6.7%) (z=−1.22, P=0.223).
For both the high and the low dissociators, the CMCT, LTI, and DTI of the transcallosal inhibition and the calculated TCT are presented in Table 1. The high dissociators had a significantly earlier LTI of the left FDI than did the low dissociators. For the right FDI, this comparison was not significant (P=0.226). Correspondingly, the TCT from the left to the right hemisphere was significantly shorter in high dissociating subjects than in low dissociating subjects. There was no significant difference in the TCT from the right to the left hemisphere between the two groups. The DTI did not significantly differ between high and low dissociators, for neither the left nor the right FDI. Entering the DES score as dependent variable into a linear regression did not reveal any significant relationship (F=0.175, df=2.71, P=0.840) with the LTI of the left FDI (standardized β=−0.05, T=−0.21, P=0.835) and the left-to-right TCT (standardized β=−0.02, T=−0.07, P=0.948).
DISCUSSION
Using a TMS approach, this is the first explorative study investigating the hypotheses that dissociative experiences are associated with an interhemispheric cortical asymmetry and a dysfunction of hemispheric interaction. We found that high dissociating students (i.e., DES scores of 30 or above) had a significantly lower left hemispheric excitability, as compared to the right hemisphere. In contrast, this difference in cortical excitability was absent in low dissociators. Furthermore, the TCT from the left to the right hemisphere of high dissociators was significantly shorter than that of low dissociators. This shortened TCT was caused by an LTI of the transcallosal inhibition and not by a shortened CMCT in the high dissociators. Although we found significant differences between the high and low dissociators, there was no linear relationship between the DES score, the TCT, and the LTI across all subjects. Presumably, these variables are strongly interrelated because the TCT is calculated on the basis of the LTI, and therefore a linear regression might be distorted for reasons of co-linearity. Alternatively, there may be a nonlinear relationship between dissociation and parameters of interhemispheric transfer. This consideration corresponds with findings indicating that dissociation does not follow a continuum (or linear) model, but can better be described by distinct types.28
The motor thresholds found in our study are comparable to values reported for other nonclinical, healthy samples, and we did not find any differences in motor thresholds between right and left FDI, which is consistent with other studies.23,24 However, high dissociators had a lower excitability of the left hemisphere, as compared to the right hemisphere. This difference was absent in the low dissociating group. It was proposed that threshold asymmetry may arise from functional asymmetry,32 and it was speculated that a lower excitability might indicate a dominance for a broad range of functions that are independent of language dominance.23 Following these considerations, our results suggest that dissociation may involve a functional superiority of the left hemisphere over the right hemisphere or, alternatively, a lack of an integration in the right hemisphere. This corresponds with the idea that the right hemisphere has a distinct role in establishing, maintaining, and processing personally relevant aspects of an individual's world.33 Thus, a right hemispheric dysfunction might result in an altered sense of personally relevant familiarity,33 which resembles phenomenologically the dissociative symptoms of depersonalization and derealization. Additionally, there is converging evidence suggesting that traumatic stress disturbs hemispheric balance,34 and trauma-related conditions, which themselves are closely associated with dissociative psychopathology,2,3 lack right hemispheric integration.13
However, one must be mindful that motor threshold asymmetries were also found in patients with treatment-refractory major depression.35 Therefore, it remains unclear whether a lower left hemispheric excitability is specific to dissociation, especially since other psychiatric disorders have been considered to involve alterations in hemispheric balance as well.36
High dissociators had a significantly earlier onset latency of the TI than low dissociators, resulting in a shorter TCT from the left to the right hemisphere. This finding seems counter-intuitive and is difficult to interpret because all investigations on TI reported that TCT is longer in the conditions of interest such as schizophrenia22 or neurological diseases.19,21 One interpretation could be that a shortened TCT in high dissociators may entail a “hyperconnectivity” between the hemispheres, contradicting the notion that dissociation is possibly due to a functional commissurotomy.5,6 However, this hypothesized “hyperconnectivity” relates to inhibitory influences, and therefore may disturb functional balance between the hemispheres. The finding that activation of the left hemisphere in high dissociators results in faster inhibition of right hemispheric regions, then vice versa, whereas low dissociators have a balanced interhemispheric inhibition, corresponds with the consideration that dissociation may involve a functional superiority of the left hemisphere over the right hemisphere.
Relating this assumption to models of hemispheric specialization, particularly to those of emotional lateralization,37,38 may provide a framework for a better understanding of the neurophysiology of trauma-related conditions and dissociation.2,3 One assertion is that the right hemisphere is responsible for the perception and processing of negative emotions, while the left hemisphere deals with positive emotions.37 A number of experimental studies, including functional imaging in healthy subjects38,39 and brain-lesioned patients,40 have confirmed this valence hypothesis. Given that dissociation involves an interhemispheric imbalance, with the left hemisphere being superior, one might speculate that in dissociation-prone individuals, a trauma that is perceived and processed by the right hemisphere will lead to a “disruption in the usually integrated functions of consciousness.”1
Our results should be interpreted cautiously, pending replication and extension to patient samples. In particular, it would be necessary to investigate patients with confirmed dissociative disorders. However, such patients may suffer from comorbid PTSD and other disorders, which in turn could prove confounding.2–5 Furthermore, the generalizability of our findings is limited by the relatively low number of high dissociators. Another critical issue might be the measurement of excitability using 75% of maximum stimulator output. If the excitability of one hemisphere is markedly different from the other, then a fixed stimulus would be relatively stronger on the one side than the other. This could result in different parameters of TI. However, using 75% of the maximum stimulator output seems reasonable because this stimulus intensity always evokes TI in normal subjects, and the parameters of TI do not markedly change with increasing stimulus intensity.19 Finally, because TMS merely allows conclusions on the transfer and processing of motor information, we can only hypothesize that our considerations also apply to sensory and cognitive information. This question is to be addressed by future studies, which should investigate whether our findings are specific to dissociation. Notwithstanding these caveats and the preliminary nature of our results, neuropsychiatric approaches to dissociation and trauma-related conditions seem to provide heuristically valuable contributions to understanding them, particularly when integrating the issue of cerebral laterality.
![]() |
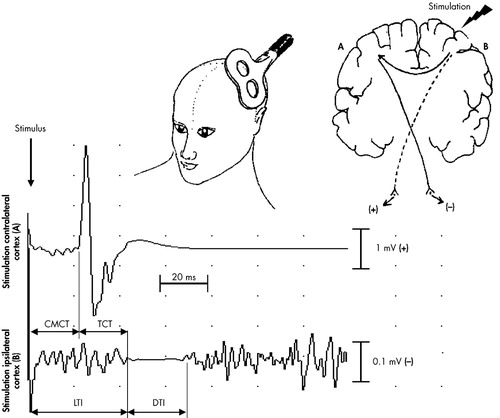
FIGURE 1. Stimulation Performed With Posterior-anterior Coil Currents and 75% of the Maximum Stimulator Output and With a Focal Coil Placed Over the Hand Area of the Motor Cortex (Upper Left).
Stimulation of the motor cortex of one hemisphere (A) activates the corticospinal tract, which results in contralateral hand motor responses, and activates callosal fibres (B), which leads to suppression of tonic EMG activity of maximally contracted ipsilateral hand muscles.
1 American Psychiatric Association: Diagnostic and Statistical Manual of Mental Disorders, 4th ed (DSM-IV). Washington, DC, APA, 1994Google Scholar
2 Putnam FW: Traumatic stress and pathological dissociation. Ann NY Acad Sci 1995; 771:708–715Crossref, Medline, Google Scholar
3 Spiegel D: Trauma, dissociation, and memory. Ann NY Acad Sci 1997; 821:225–237Crossref, Medline, Google Scholar
4 Bremner JD, Marmar CR (eds): Trauma, Memory, and Dissociation. Washington, DC, American Psychiatric Press, 1998Google Scholar
5 Krystal JH, Bremner JD, Southwick SM, et al: The emerging neurobiology of dissociation: implications for treatment of posttraumatic stress disorder, in Trauma, Memory, and Dissociation. Edited by Bremner JD, Marmar CR. Washington, DC, American Psychiatric Press, 1998, pp 321–363Google Scholar
6 Putnam FW: Dissociative phenomena, in Dissociative Disorders. A Clinical Review. Edited by Spiegel D. Lutherville, Md, Sidran Press, 1993, pp 1–16Google Scholar
7 Lipsanen T, Lauerma H, Peltola P, et al: Associations among dissociative experiences, handedness, and demographic variables in a nonclinical population. J Nerv Ment Dis 2000; 188:422–427Crossref, Medline, Google Scholar
8 Spiegel D: Neurophysiological correlates of hypnosis and dissociation. J Neuropsychiatry Clin Neurosci 1991; 3:440–445Link, Google Scholar
9 Henninger P: Conditional handedness: handedness changes in multiple personality disordered subject reflect shift in hemispheric dominance. Conscious Cogn 1992; 1:265–287Crossref, Google Scholar
10 Ahern GL, Herring AM, Tackenberg J, et al: The association of multiple personality and temporolimbic epilepsy: intracarotid amobarbital test observations. Arch Neurol 1993; 50:1020–1025Crossref, Medline, Google Scholar
11 Spivak B, Segal M, Mester R, et al: Lateral preference in post-traumatic stress disorder. Psychol Med 1998; 28:229–232Crossref, Medline, Google Scholar
12 Teicher MH, Ito Y, Glod CA, et al: Preliminary evidence for abnormal cortical development in physically and sexually abused children using EEG coherence and MRI. Ann NY Acad Sci 1997; 821:160–175Crossref, Medline, Google Scholar
13 Schiffer F, Teicher MH, Papanicolaou AC: Evoked potential evidence for right brain activity during the recall of traumatic memories. J Neuropsychiatry Clin Neurosci 1995; 7:169–175Link, Google Scholar
14 Shin LM, McNally RJ, Kosslyn SM, et al: Regional cerebral blood flow during script-driven imagery in childhood sexual abuse-related PTSD: a PET investigation. Am J Psychiatry 1999; 156:575–584Medline, Google Scholar
15 De Bellis MD, Keshavan MS, Clark DB, et al: AE Bennett Research Award: developmental traumatology, part II: brain development. Biol Psychiatry 1999; 45:1271–1284Crossref, Medline, Google Scholar
16 George MS, Wassermann EM, Post RM: Transcranial magnetic stimulation: a neuropsychiatric tool for the 21st century. J Neuropsychiatry Clin Neurosci 1996; 8:373–382Link, Google Scholar
17 Pascual-Leone A, Tormos JM, Keenan J, et al: Study and modulation of human cortical excitability with transcranial magnetic stimulation. J Clin Neurophysiol 1998; 15:333–343Crossref, Medline, Google Scholar
18 Ferbert A, Priori A, Rothwell JC, et al: Interhemispheric inhibition of the human motor cortex. J Physiol 1992; 453:525–546Crossref, Medline, Google Scholar
19 Meyer BU, Roricht S, Grafin von Einsiedel H, et al: Inhibitory and excitatory interhemispheric transfers between motor cortical areas in normal humans and patients with abnormalities of the corpus callosum. Brain 1995; 118:429–440Crossref, Medline, Google Scholar
20 Gazzaniga MS: Cerebral specialization and interhemispheric communication: does the corpus callosum enable the human condition? Brain 2000; 123:1293–1326Crossref, Medline, Google Scholar
21 Boroojerdi B, Diefenbach K, Ferbert A: Transcallosal inhibition in cortical and subcortical cerebral vascular lesions. J Neurol Sci 1996; 144:160–170Crossref, Medline, Google Scholar
22 Boroojerdi B, Topper R, Foltys H, et al: Transcallosal inhibition and motor conduction studies in patients with schizophrenia using transcranial magnetic stimulation. Br J Psychiatry 1999; 175:375–379Crossref, Medline, Google Scholar
23 Triggs WJ, Calvanio R, Macdonell RA, et al: Physiological motor asymmetry in human handedness: evidence from transcranial magnetic stimulation. Brain Res 1994; 636:270–276Crossref, Medline, Google Scholar
24 Civardi C, Cavalli A, Naldi P, et al: Hemispheric asymmetries of cortico-cortical connections in human hand motor areas. Clin Neurophysiol 2000; 111:624–629Crossref, Medline, Google Scholar
25 Priori A, Oliviero A, Donati E, et al: Human handedness and asymmetry of the motor cortical silent period. Exp Brain Res 1999; 128:390–396Crossref, Medline, Google Scholar
26 Rossini PM, Barker AT, Berardelli A, et al: Non-invasive electrical and magnetic stimulation of the brain, spinal cord and roots: basic principles and procedures for routine clinical application: report of an IFCN committee. Electroencephalogr Clin Neurophysiol 1994; 91:79–92Crossref, Medline, Google Scholar
27 Bernstein EM, Putnam FW: Development, reliability, and validity of a dissociation scale. J Nerv Ment Dis 1986; 174:727–735Crossref, Medline, Google Scholar
28 Putnam FW, Carlson EB, Ross CA, et al: Patterns of dissociation in clinical and nonclinical samples. J Nerv Ment Dis 1996; 184:673–679Crossref, Medline, Google Scholar
29 Van Ijzendoorn MH, Schuengel C: The measurement of dissociation in normal and clinical populations: meta-analytic validation of the Dissociative Experience Scale. Clin Psychol Rev 1996; 16:365–382Crossref, Google Scholar
30 Spitzer C, Freyberger HJ, Stieglitz RD, et al: Adaptation and psychometric properties of the German version of the Dissociative Experience Scale. J Trauma Stress 1998; 11:799–809Crossref, Medline, Google Scholar
31 Oldfield RC: The assessment and analysis of handedness: the Edinburgh Inventory. Neuropsychologia 1971; 9:97–113Crossref, Medline, Google Scholar
32 Matsunaga K, Uozumi T, Tsuji S, et al: Age-dependent changes in physiological threshold asymmetries for the motor evoked potential and silent period following transcranial magnetic stimulation. Electroencephalogr Clin Neurophysiol 1998; 109: 502–507Google Scholar
33 Van Lancker D: Personal relevance and the human right hemisphere. Brain Cogn 1991; 17:64–92Crossref, Medline, Google Scholar
34 Bremner JD: Does stress damage the brain? Biol Psychiatry 1999; 45:797–805Crossref, Medline, Google Scholar
35 Maeda F, Keenan JP, Pascual-Leone A: Interhemispheric asymmetry of motor cortical excitability in major depression as measured by transcranial magnetic stimulation. Br J Psychiatry 2000; 177:169–173Crossref, Medline, Google Scholar
36 Cutting J: The Right Cerebral Hemisphere and Psychiatric Disorders. Oxford, UK, Oxford University Press, 1990Google Scholar
37 Silberman EK, Weingartner H: Hemispheric lateralization of functions related to emotion. Brain Cogn 1986; 5:322–353Crossref, Medline, Google Scholar
38 Spence S, Shapiro D, Zaidel E: The role of the right hemisphere in the physiological and cognitive components of emotional processing. Psychophysiology 1996; 33:112–122Crossref, Medline, Google Scholar
39 Canli T, Desmond JE, Zhao Z, et al: Hemispheric asymmetry for emotional stimuli detected with fMRI. Neuroreport 1998; 9:3233–3239Google Scholar
40 Borod JC, Cicero BA, Obler LK, et al: Right hemisphere emotional perception: evidence across multiple channels. Neuropsychology 1998; 12:446–458Crossref, Medline, Google Scholar