Internal Capsule Size in Good-Outcome and Poor-Outcome Schizophrenia
A problem with several studies of white matter in schizophrenia is that they have examined gross regional areas without measurement of specific pathways that are implicated in schizophrenia-associated circuitry abnormalities. This limitation is likely a result of the inherent difficulty in the quantification of white matter, given its diffuse representation throughout the entire brain. Although few white matter pathways have discrete anatomical borders, the anterior limb of the internal capsule is uniquely delimited by subcortical nuclei that can be used for reliable anatomical landmarks in manual tracing or region-of-interest (ROI) approaches. The anterior limb of the internal capsule contains descending motor and ascending sensory fibers interconnecting brain regions implicated in the pathophysiology of schizophrenia, including neocortex, striatum, thalamus, and pons. Disruption of this fiber bundle can result in cognitive deficit with a “subcortical” profile that is similar to the cognitive deficits seen in schizophrenia. 10 Furthermore, the internal capsule contains a heterogeneous set of neurons, including cholinergic and noncholinergic projections, which may mediate sensory and behavioral functioning often affected in schizophrenia. 11 The anterior limb of the internal capsule contains frontothalamic and thalamofrontal pathways, the corticopontine pathways, and a lesser number of caudate/pallidum fibers. 12 , 13 Thalamocortical fibers appear most concentrated in the anterior tip, while the posterior section of the anterior limb of the internal capsule has primarily corticopontine fibers. 13 Reduction in the size of the internal capsules in patients with schizophrenia would be consistent with diminished corticothalamic and corticostriatal connectivity and with previous reports of schizophrenia-associated abnormalities in the striatum 14 , 15 and thalamus. 16 – 21 Indeed, a recent study from our laboratory 22 demonstrated a significant relationship between frontal lobe size and thalamus size in schizophrenia patients with poor functional outcome, suggesting faulty interconnections between the two structures.
Previous studies examining the morphology of the internal capsules in schizophrenia suggest a reduction in volume. Manual tracings of the anterior limb of the internal capsules on coronal magnetic resonance imaging (MRI) slices in 53 patients with schizophrenia and 48 healthy comparison subjects revealed bilateral decrease in volume in the patient group. 23 A similar study of patients with schizotypal personality disorder, thought to be on the schizophrenia “spectrum,” reported volume reduction in the right internal capsule. 24 Schizophrenia-associated volume decreases in the internal capsules were also observed with voxel-based morph(ometry, 23 – 25 although one study using voxel-based morphometry did not find anterior limb volume differences between patients with schizophrenia and healthy comparison subjects. 5
A study of 39 schizophrenia patients and estimates from voxel-based morphometry showed significant correlations between right posterior internal capsule volume and illness duration, but contrasts with normal volunteers were not available. 26 Multiplanar manual tracing of the internal capsule can provide better regional specificity than approaches that consider voxel-wide or full volume comparisons, which require spatial normalization that might obscure the shape of smaller regions (see Giuliani et al. 27 for discussion). By tracing the internal capsules at various slices in the dorsal-to-ventral plane, comparisons with other brain regions at various slice levels can be made to examine more comprehensively the interrelationships among brain regions. Furthermore, multiplanar tracing allows for examination of the shape of the internal capsules as well as overall size. For example, the lateral ventricles appear at more dorsal levels of the internal capsules, but not at ventral levels. Expansion of the lateral ventricles could put pressure on adjacent internal capsule fibers, thus narrowing the fiber tract at dorsal levels or pushing them in the ventral direction without affecting the overall size or volume of the structure.
Given previous findings of ventriculomegaly in poor-outcome patients, 28 , 29 it is important to explore the relationships between the size of the internal capsule at different levels and the size of the lateral ventricles. Further, examination of the intercorrelation among the volumes of brain regions may shed light on potential trophic relationships or circuitry abnormalities implicated in schizophrenia (see Mitelman et al. 30 for discussion). Thus, another goal of the current study was to explore the relationship between internal capsule size and volumes of other regions implicated in good and poor-outcome schizophrenia. 16 , 31 – 33 For the current study, a novel neuromorphometric region-of-interest protocol for examining the size of the anterior limb of the internal capsule was developed. The primary purpose of the study was to examine the size of the anterior limb of the internal capsule in healthy comparison subjects compared to good- and poor-outcome schizophrenia patients (“non-Kraepelinian” and “Kraepelinian” patients, respectively). Distinguishing patients by functional status has been one strategy of reducing clinical heterogeneity in schizophrenia. Poor-outcome patients are defined as being dependent on others for food, clothing, and shelter for a continuous period of 5 years or longer, whereas good-outcome patients are more independent in these functional domains. 34 Recent MRI studies have shown reliable differences between the two groups in cortical gray matter distribution, 32 cingulate cortex volume, 33 striatal size, 31 and thalamus size. 16 , 22 We hypothesized that there would be a reduction in internal capsule size in the two schizophrenia groups, which would be more pronounced in the poor-outcome patients. Further, we expected that the size of the internal capsules would be related to the severity of psychopathology and to volumes of surrounding cortical and subcortical regions.
METHOD
Subjects
The schizophrenia group comprised 106 patients, and there were 42 demographically similar healthy comparison subjects who were recruited through flyers placed around the hospital community, advertisements in local area newspapers, and word-of-mouth. Patients in the current study were referred from clinicians in the outpatient departments at the Mount Sinai School of Medicine and the Bronx VA Medical Center and from inpatient departments at Pilgrim State Psychiatric Institute. Referring clinicians were informed of the inclusion and exclusion criteria of the study and referred patients that they felt were appropriate for participation. Referred patients were screened by study personnel to confirm that study criteria were met. All schizophrenia patients met DSM-IV diagnostic criteria for schizophrenia (N=95) or schizoaffective disorder (N=11). Diagnosis was determined by a structured clinical interview using the Comprehensive Assessment of Symptoms and History (CASH). 35 Schizophrenia patients were divided into good-outcome (N=52, including five schizoaffective bipolar type and three schizoaffective depressed type) and poor-outcome (N=54, including two schizoaffective bipolar type and one schizoaffective depressed type) subgroups based on objective criteria put forth by Keefe et al. 34 Briefly, poor-outcome, or “Kraepelinian,” patients met the following criteria for at least 5 years prior to study contact: 1) continuous hospitalization, or, if living outside the hospital, complete dependence on others for food, clothing, and shelter; 2) no useful work or employment; and 3) no evidence of symptom remission. All other schizophrenia patients were considered good-outcome, or “non-Kraepelinian.”
Demographic and clinical data were collected at the time of MR image acquisition; these are displayed in Table 1 . The three groups were similar in age ( F (2, 145)=1.52, p=0.221) and sex distribution (χ 2 (2)=3.06, p=0.216). Schizophrenia patients were assessed with the Positive and Negative Syndrome Scales (PANSS), 36 and poor-outcome patients had more severe positive symptom, negative symptom, and general subscale scores (all F-values>15.00, p<0.0001), consistent with their more severe psychopathology. For the schizophrenia patients, interview and clinical chart reviews were conducted to determine age of first neuroleptic exposure and medication history over the 3-year period prior to scan acquisition. To examine the effects of neuroleptic exposure, we classified patient treatment at the time of MRI scan and for the predominant pattern over the 3-year period prior to the scan as off-medication, typical neuroleptics, atypical neuroleptics, or both typical and atypical neuroleptics. Poor-outcome patients began neuroleptic treatment significantly earlier than good-outcome patients t (82)=2.02, p=0.006). The distribution of type of neuroleptic exposure was similar between the two groups at the time of scan (χ 2 (3)=4.36, p=0.225) and for the majority of time over the 3-year period prior to the scan (χ 2 (3)=4.14, p=0.247). MRI data from participants in the current study have been reported previously in studies of the thalamus, striatum, cingulate, and Brodmann areas of the cortex 16 , 22 , 31 – 33 .
![]() |
MR Image Acquisition
The 1.5 T Signa 5x system (GE Medical Systems, Milwaukee) with a three-dimensional SPGR sequence (TR=24 msec, TE=5 msec, flip angle=40° and slice thickness=1.22 mm, matrix size 256×256, total slices=128) was used for T 1 -weighted image acquisition. MR images were resliced using SPM 99 (Wellcome Department of Imaging Neuroscience, London) to adjust along the anterior commissure-posterior commissure (AC-PC) axis with a 6-parameter rigid body transformation. AC-PC adjustments were made blind to subject diagnosis.
Manual Tracing of the Anterior Limb of the Internal Capsules
The left and right anterior internal capsules were manually traced in the axial plane on five dorsal-to-ventral equidistant levels. The five slices were determined based on anatomy of the striatum, following Buchsbaum et al. 31 Briefly, the most ventral and most dorsal slices containing the putamen were selected. The most ventral aspect was defined as the slice in which the caudate and putamen were entirely merged. The most dorsal slice was defined as the first slice in which the putamen was no longer visible. The number of slices between the most dorsal and most ventral slices was divided by six to yield an increment accurate to two decimal places. This increment was added to the bottom slice number five times and the result rounded to yield five equally and proportionately spaced slices for tracing. The internal capsule was traced on the five slices corresponding to the five equidistant spaced slices of putamen. The tracing protocol was developed after consultation with a neuroanatomist, expert in cerebral white matter, with the intent of maximizing consistent measurements across subjects while capturing fibers restricted to the anterior limb of the internal capsule region. Four manually inserted landmarks were used to create the “corners” of a polygon containing the fibers of the internal capsule ( Figure 1 ). For each hemisphere, a landmark was placed on the most lateral anterior part of the caudate nucleus to define the anterior medial corner of the internal capsules (point 1 in Figure 1 ). A landmark was placed on the most medial anterior part of the putamen to define the anterior lateral corner of the internal capsule (point 2 in Figure 1 ). For the more posterior medial and lateral corners, a landmark was placed on the most posterior part of the caudate nucleus, and one was placed on the most medial aspect of the putamen, respectively (points 3 and 4 in Figure 1 ). An automatic boundary-finding method, based on the Sobel-gradient filter, allowed for maximization of gray/white matter contrast for accurate placement of landmarks on the borders between the striatum and internal capsules. Figure 1 displays an example of placement of landmarks on the five equidistant slices. To determine interrater reliability for the manual tracing protocol, 10 subjects were chosen randomly and traced by two independent operators, and total size, across hemisphere, were compared between the two. The intraclass correlation (ICC) was 0.74, which is consistent with other studies that have examined the tracer reliability of small structures (for discussion, see Spinks et al.). 37 An automatic computer program developed by M.S. Buchsbaum was used to compute the area of the internal capsule at each slice, which was contained in a polygon formed by the four landmarks. A three-dimensional reconstruction of the internal capsule is displayed in Figure 2 . Following established protocols, the size of the striatum (i.e., caudate and putamen, 31 thalamus), 16 lateral ventricles (anterior horn, temporal horn, lateral), 38 and frontal and temporal gray and white matter volume 32 were determined.

Left images are with the Sobel-gradient filter applied, and right images are raw. Landmarks are shown in yellow and are labeled on the most ventral slice. Point #1 is the anterior, medial landmark. Point #2 is the anterior, lateral landmark. Point #3 is the posterior, medial landmark. Point #4 is the posterior, lateral landmark.

The 3-dimensional reconstruction is illustrated in red as it bisects a mid-cerebral axial slice. Orthogonal coronal and sagittal slices are displayed for reference. MRI intensity values are color coded, with red and yellow corresponding to white matter, green corresponding to gray matter, and blue to CSF.
Data Analysis
Data were entered into a repeated-measures nested-design analysis of variance (ANOVA) with the diagnostic group (2 levels: healthy comparison subject, schizophrenia) as a between-subjects factor and outcome group (2 levels: good-outcome, poor-outcome) nested within the schizophrenia group. Slice Level (5 levels: ventral to dorsal) and Hemisphere (2 levels: left, right) were within-subjects factors. The analyses were run first on relative values, calculated as the ratio of the internal capsule size at each level in each hemisphere to total brain volume, and then on absolute values. Follow-up simple interactions and pairwise post hoc tests were used to identify the greatest source of variance for significant interactions involving diagnosis. Pearson's product-moment correlation coefficients were calculated to examine the relationship between dorsal and ventral internal capsule size and the size of the lateral ventricles, caudate, putamen, and thalamus. For these analyses, we computed dorsal internal capsule size by summing the volume of the two most dorsal slices in both hemispheres, and by summing the ventral internal capsule size of the three most ventral slices across hemisphere. We conducted exploratory analyses between dorsal and ventral internal capsule size, sex, and age at which the patient was first treated, duration of neuroleptic treatment, and positive, negative, and general PANSS scores in all schizophrenia patients grouped together.
RESULTS
Relative Internal Capsule Size
The nested design analysis on relative internal capsule size revealed a trend-level Diagnostic Group (good- and poor-outcome nested within) by slice level interaction ( F (4, 580)=2.1917, p=0.0688) ( Figure 3 ). Although follow-up analyses did not reach statistical significance, the pattern of the interaction suggests that compared to both the good-outcome group and healthy comparison subjects, the poor-outcome group has relatively larger internal capsule volume at the most ventral levels and relatively reduced volume at the most dorsal levels. On the other hand, good-outcome patients and healthy comparison subjects had relatively similar internal capsule volume across the five levels. No other effects involving Diagnostic Group approached statistical significance.
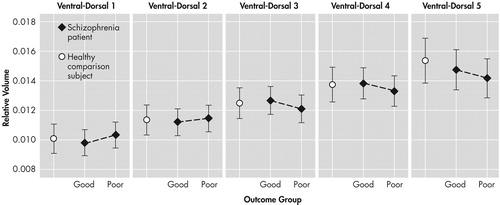
Vertical bars denote 0.95 confidence interval.
Absolute Internal Capsule Size
As described above, we conducted a similar nested-design ANOVA on absolute internal capsule size. Poor-outcome patients had significantly smaller internal capsule size than good-outcome patients and comparison subjects (significant main effect of Diagnostic Group [good and poor-outcome patients nested within], F (1, 145)=10.159, p=0.0176) ( Figure 4 ). This effect was increasingly larger at more dorsal slices (significant Diagnostic Group [good and poor-outcome nested within] by slice level interaction, F (4, 580)=3.2438, p=0.001201) ( Figure 5 ). Follow-up analyses revealed that the good-outcome group and the healthy comparison subjects did not significantly differ from each other at any of the slice levels (p>0.05). Follow-up tests with the poor-outcome patients revealed significant differences from comparison subjects at the most dorsal slice (p=0.02) and significant differences from good-outcome patients at the fourth slice (p=0.04) and most dorsal slice (p<0.01). No other effects involving the diagnostic group approached statistical significance.

Vertical bars denote 0.95 confidence interval.

Vertical bars denote 0.95 confidence interval.
Clinical Correlates of Internal Capsule Size
Relative (to whole brain volume) internal capsule size did not significantly correlate (p>0.05) with age, age at first neuroleptic exposure, type of neuroleptic at time of scan or for the 3-year period prior to scan acquisition, PANSS scores, or sex when all of the schizophrenia patients were considered together. When the two schizophrenia patient groups were considered separately, there was a significant negative association between age at scan and internal capsule size at the most dorsal level (r (24)=−0.43, p<0.05) in the poor-outcome group. In the good-outcome group, men tended to have larger relative internal capsules in the middle slice (r (35)=0.47, p<0.05) and there was a significant positive association between the size of the most ventral slice of the internal capsule and age of commencement of neuroleptic treatment, a correlate of age of onset (r (35)=0.49, p<0.05). As differences between groups tended to vary as a function of ventral-to-dorsal slice level, ratio scores of the three most ventral slices to the two most dorsal slices were calculated for comparisons with the clinical variables. When the two schizophrenia patient groups were combined, the only significant association to emerge was a positive one between the ratio score and age of commencement of neuroleptic treatment (r (84)=0.28, p<0.05).
Correlations With Other Brain Regions
We examined correlation coefficients between relative size of the ventral and dorsal levels of the internal capsules and average size of subcortical nuclei, lateral ventricles, and cortical regions. Subcortical nuclei included thalamus, caudate and putamen; average values (collapsed across hemisphere) were calculated for each of the five ventral to dorsal slices for each nucleus. We conducted correlations, controlling for whole brain volume, and examined relationships for healthy comparison subjects, combined schizophrenia groups, and each of the schizophrenia subtypes separately. For the thalamus ( Table 2 ), significant positive associations emerged between dorsal internal capsule and the most ventral and the most dorsal thalamus size in healthy comparison subjects. In the entire schizophrenia sample, ventral internal capsule size was significantly positively associated with the second most dorsal level (slice 4) of the thalamus. In good-outcome patients, this pattern emerged for the two most dorsal levels of thalamus. However, in the poor-outcome patient group, there were no significant associations between internal capsule size and thalamus size; qualitative examination of the effect sizes in this group revealed coefficients close to zero. For the caudate nucleus ( Table 3 ), we observed significant positive associations between the ventral internal capsule and the second most ventral level (slice 2), between the ventral internal capsule and second most dorsal level (slice 4), and between the dorsal internal capsule and second most ventral level (slice 2) in the healthy comparison group. In the combined schizophrenia group, there was a significant positive association between the ventral internal capsule and the middle caudate slice, a pattern that was similar in the poor-outcome group, but not in the good-outcome group. Of note, however, the two correlation coefficients for that association did not significantly differ (p=0.59) between the two schizophrenia patient groups with Fisher's z test. For the putamen ( Table 4 ), several significant associations emerged. First, ventral internal capsule size was positively correlated with the second most ventral level of putamen (slice 2) in healthy comparison subjects. A negative association emerged between the dorsal internal capsule and the most ventral level of putamen, but the associations with the next two levels (slices 2 and 3) were positive. In the schizophrenia group, both the ventral and dorsal internal capsule were positively associated with the middle putamen level (slice 3). For the good-outcome group, there was a significant positive association between the dorsal internal capsule and the second most ventral level (slice 2), whereas in the poor-outcome group, there was a positive association between ventral internal capsule and the middle putamen slice. Several significant negative associations emerged for the ventricles ( Table 5 ). In healthy comparison subjects, there were significant correlations between both dorsal and ventral internal capsules and anterior horn, temporal horn, and lateral aspects of the ventricles. In the combined schizophrenia group, only the association between dorsal internal capsule and the size of the anterior horn was statistically significant. In the good-outcome group, the dorsal internal capsule was associated with anterior horn and lateral ventricular size. No significant associations emerged in the poor-outcome group. There were no significant correlations between ventral or dorsal internal capsule and frontal or temporal gray and white matter size in any of the study groups.
![]() |
![]() |
![]() |
![]() |
DISCUSSION
The findings from this study provide tentative support for internal capsule size reduction in schizophrenia. These abnormalities may be restricted to dorsal levels of the internal capsule in poor functioning patients and are associated with age. Significant correlations emerged, particularly between internal capsule size in healthy comparison subjects and the surrounding size of subcortical brain regions. However, these associations tended to become weaker with progressive decline in functional outcome. Taken together, these findings are suggestive of internal capsule abnormalities in poor-outcome patients, which may reflect a neurodegenerative process and disrupted cortical-subcortical circuitry. Analyses of relative and absolute internal capsule size revealed several important findings. First, schizophrenia patients, as a single group, did not significantly differ from healthy comparison subjects. A trend level interaction on relative values suggested that poor-outcome patients had smaller internal capsules at more dorsal levels and larger internal capsules at more ventral levels than both good-outcome patients and healthy comparison subjects. Analyses on absolute values confirmed that poor-outcome patients had significantly smaller internal capsules, particularly at dorsal levels. Taken together, the findings suggest abnormal fiber distribution in poor-outcome patients. Only one published study, to our knowledge, has taken an a priori, region-of-interest approach to examining the internal capsules in schizophrenia. 23 In that study, the authors used manual tracing and confirmatory analysis with voxel-based morphometry to demonstrate that total bilateral anterior limb volume was decreased in schizophrenia patients compared to matched healthy subjects. Findings from the current study are somewhat consistent with this report; reduced internal capsule size was found, but restricted to poor-outcome patients. Discrepancies between the two studies could be due to subject characteristics. That is, functional status (e.g., inpatient, outpatient, or institutionalized) and disease severity of the patient sample in the Zhou et al. 23 paper were not reported. Thus, their sample could have been composed of particularly poor functioning patients. Unlike Zhou et al., the current study did not find greater right- than left-sided asymmetry in the patient group relative to healthy comparison subjects.
Two other studies using voxel-based morphometry have reported significant anterior limb internal capsule reduction in schizophrenia patients compared to healthy subjects. 24 , 25 These voxel-based morphometry studies are somewhat exploratory because they did not test a priori hypotheses about specific anatomical regions. Instead, they provided important information on a whole brain, voxel-by-voxel basis. Thus, the two positive findings of internal capsule decreases in schizophrenia with this method should be interpreted cautiously given that most studies using voxel-based morphometry have failed to report similar differences. Because the internal capsules comprise a portion of the larger corona radiata, which has widespread cerebral distribution, their fibers are at least indirectly involved with projections to and from most cortical regions. 39 In terms of the specific pathways comprising the region, the anterior thalamic peduncle forms a large portion of the anterior limb, connecting anterior and medial nuclei of the thalamus with the cingulate gyrus and with dorsolateral and medial aspects of the frontal lobe, as has been demonstrated with polarized light and confocal scanning laser microscopy. 13 Fibers extending between caudate nucleus and globus pallidus are also found in the anterior limb, 12 , 13 particularly at dorsal levels of the pallidum. 40 Finally, frontopontine fibers, which were well described in classic post-mortem studies of individuals with leucotomy, 41 have been shown to occupy approximately 37% of anterior limb volume. 12 Consistent with the systematic distribution of the corona radiata throughout the brain, fibers coursing through the anterior limb of the internal capsule are topographically organized, 39 particularly in the anterior-posterior direction. 12 However, several of the fiber bundles intertwine with one another and are distinguishable by their slope of projection, rather than their discrete boundaries. 13 Consideration of the pathways contained within the anterior limb of the internal capsules suggests particular frontothalamic, thalamofrontal, and intrastriatal abnormalities in poor-outcome patients. Findings from our recent examination of the thalamus, 16 indeed, demonstrated thalamic volume reduction in the same cohort of poor-outcome patients compared to good-outcome patients and healthy comparison subjects. Furthermore, the results are consistent with a study on a subsample of the included participants, 31 which suggested striatal abnormalities in poor-outcome patients. Correlation coefficients examining the relationship between internal capsule size and the size of surrounding cortical and subcortical regions provide some further insight into the nature of the internal capsule size reduction seen in the poor-outcome patients. By far, the most consistent associations were between ventral and dorsal internal capsule size and all aspects of the lateral ventricles; larger ventricles were associated with smaller internal capsules in healthy comparison subjects.
This normal relationship suggests that enlargement of the ventricles contributes to reduction in size of the internal capsule, potentially as a secondary effect of atrophy or increased ventricular pressure. However, this association was not as consistent in the good-outcome patients and nonexistent in the poor-outcome patients. The markedly enlarged ventricles 28 , 42 and reduced internal capsule size in the poor-outcome patients may be, therefore, the result of independent pathophysiological processes. This finding lends support to the idea that ventricular enlargement in poor-outcome patients is endophenotypic and does not cause secondary surrounding structural reduction.
Consistent with the report by Zhou et al., 23 we found significant positive associations between ventral and dorsal internal capsule size and some levels of the caudate in the healthy comparison subjects, but these associations were less consistent in either of the patient groups. Dorsal internal capsule sizes were not related at all to caudate size at any level in either of the schizophrenia groups when considered separately or alone. Given that the caudate nucleus forms the medial border of the internal capsules, the finding of a normal association between the two structures is not surprising. However, the lack of association in the schizophrenia patients, particularly the poor-outcome group, suggests that internal capsule size reduction is independent of variability in the caudate. Our findings are not consistent with those of Hulshoff Pol et al., 25 who reported negative associations between internal capsule and caudate density, attributing the finding to normal brain maturation.
A somewhat similar pattern emerged for associations with the putamen. Significant correlations were strongest in healthy comparison subjects, but still present in both schizophrenia groups. Given that slices for analysis of the internal capsule were chosen based on putamen morphology and that the internal capsule borders the lenticular nucleus, these associations are not unexpected. Although some significant positive associations were found between ventral and dorsal aspects of the internal capsule and levels of the thalamus in all three groups, they were less consistent than expected. Dorsal internal capsule was associated with dorsal and ventral thalamic size in healthy comparison subjects, whereas ventral aspects of the internal capsule were associated with dorsal slices of the thalamus in good-outcome patients. Poor-outcome patients evidenced no significant relationships. These findings tentatively suggest a greater disruption in circuitry as a function of worsening functional outcome, but do not support the idea that one structure affects the other. Similarly, there was a lack of significant associations between internal capsule size and cortical gray or white matter volume in any of the groups.
The current study found a significant negative association between age at the time of scan and dorsal internal capsule size in the poor-outcome group, but not in the good-outcome patients. The finding speaks to the possibility that poor-outcome patients, who exhibited dorsal internal capsule size reduction, have a progressive, neurodegenerative course. It is also somewhat consistent with a report by Velakoulis et al., 26 who found a negative association between right internal capsule volume and illness duration in a group of schizophrenia patients. Similar to Hulshoff Pol et al., 25 the current study found little relationship between internal capsule size and exposure to neuroleptic treatment and only a modest effect of sex on internal capsule size.
There are some limitations to the current study. The anterior limb of the internal capsules contains fibers that are part of the corona radiata, a much larger, diffusely distributed cortical-subcortical white matter system. A benefit to studying morphometry of the internal capsules is that it is largely contained within surrounding subcortical structures, facilitating reliable boundary demarcation. However, positive findings in the internal capsule are likely to be representative of much more diffuse white matter changes; given the intertwining fiber bundles and the anterior-posterior topography contained within the structure, 13 it is difficult to draw conclusions about which of the specific pathways contained within the internal capsules are implicated, particularly when considering the internal capsules in the dorsal-ventral direction. Studies using diffusion tensor, an MRI sequence that provides information about the coherence and directionality of white matter fibers, would complement morphometric efforts and help better characterize white matter abnormalities in good- and poor-outcome patients. Indeed, in the first study using diffusion tensor in schizophrenia patients, Buchsbaum et al. 43 demonstrated anisotropic reductions in areas of the anterior limb of the internal capsule. Further, preliminary diffusion tensor analyses with a subset of patients from the current cohort 44 showed schizophrenia-associated anterior anisotropic reduction, but greater reduction in posterior regions in poor-outcome patients. A second limitation is the restriction of the area to definition by four points to enhance reliability, although the geometry of the parallelogram shape is generally well matched by the internal capsule appearance.
In conclusion, internal capsule size reduction in this study was restricted to poor-outcome patients at dorsal levels and appeared to be somewhat related to age. These findings suggest neurodegeneration in this poor-outcome group. Internal capsule size appeared to be most related to ventricular size in healthy comparison subjects, but this relationship was less strong in good-outcome patients and nonexistent in poor-outcome patients. Disruption of fibers coursing through the internal capsule in poor-outcome patients may be reflective of myelin-related or oligodendroglial abnormalities or neurodevelopmental maturational problems. Findings from this study are less supportive of trophic effects.
1. Davis KL, Stewart DG, Friedman JI, et al: White matter changes in schizophrenia: evidence for myelin-related dysfunction. Arch Gen Psychiatry 2003; 60:443–456Google Scholar
2. Wright IC, Rabe-Hesketh S, Woodruff PW, et al: Meta-analysis of regional brain volumes in schizophrenia. Am J Psychiatry 2000; 157:16–25Google Scholar
3. Breier A, Buchanan RW, Elkashef A, et al: Brain morphology and schizophrenia: a magnetic resonance imaging study of limbic, prefrontal cortex, and caudate structures. Arch Gen Psychiatry 1992; 49:921–926Google Scholar
4. Buchanan RW, Vladar K, Barta PE, et al: Structural evaluation of the prefrontal cortex in schizophrenia. Am J Psychiatry 1998; 155:1049–1055Google Scholar
5. Paillere-Martinot M, Caclin A, Artiges E, et al: Cerebral gray and white matter reductions and clinical correlates in patients with early onset schizophrenia. Schizophr Res 2001; 50(1–2):19–26Google Scholar
6. Sanfilipo M, Lafargue T, Rusinek H, et al: Volumetric measure of the frontal and temporal lobe regions in schizophrenia: relationship to negative symptoms. Arch Gen Psychiatry 2000; 57:471–480Google Scholar
7. Sigmundsson T, Suckling J, Maier M, et al: Structural abnormalities in frontal, temporal, and limbic regions and interconnecting white matter tracts in schizophrenic patients with prominent negative symptoms. Am J Psychiatry 2001; 158:234–243Google Scholar
8. Cannon TD, van Erp TG, Huttunen M, et al: Regional gray matter, white matter, and cerebrospinal fluid distributions in schizophrenic patients, their siblings, and controls. Arch Gen Psychiatry 1998; 55:1084–1091Google Scholar
9. Foong J, Symms MR, Barker GJ, et al: Neuropathological abnormalities in schizophrenia: evidence from magnetization transfer imaging. Brain 2001; 124(pt 5):882–892Google Scholar
10. Chukwudelunzu FE, Meschia JF, Graff-Radford NR, et al: Extensive metabolic and neuropsychological abnormalities associated with discrete infarction of the genu of the internal capsule. J Neurol Neurosurg Psychiatry 2001; 71:658–662Google Scholar
11. Zaborszky L, Pang K, Somogyi J, et al: The basal forebrain corticopetal system revisited. Ann N Y Acad Sci 1999; 877:339–367Google Scholar
12. Axer H, Lippitz BE, von Keyserlingk DG: Morphological asymmetry in anterior limb of human internal capsule revealed by confocal laser and polarized light microscopy. Psychiatry Res 1999; 91:141–154Google Scholar
13. Axer H, Keyserlingk DG: Mapping of fiber orientation in human internal capsule by means of polarized light and confocal scanning laser microscopy. J Neurosci Methods 2000; 94:165–175Google Scholar
14. Shihabuddin L, Buchsbaum MS, Hazlett EA, et al: Dorsal striatal size, shape, and metabolic rate in never-medicated and previously medicated schizophrenics performing a verbal learning task. Arch Gen Psychiatry 1998; 55:235–243Google Scholar
15. Shihabuddin L, Buchsbaum MS, Hazlett EA, et al: Striatal size and relative glucose metabolic rate in schizotypal personality disorder and schizophrenia. Arch Gen Psychiatry 2001; 58:877–884Google Scholar
16. Brickman AM, Buchsbaum MS, Shihabuddin L, et al: Thalamus size and outcome in schizophrenia. Schizophr Res 2004; 71(2–3):473–484Google Scholar
17. Buchsbaum MS, Someya T, Teng CY, et al: PET and MRI of the thalamus in never-medicated patients with schizophrenia. Am J Psychiatry 1996; 153(2):191–199Google Scholar
18. Byne W, Buchsbaum MS, Kemether E, et al: Magnetic resonance imaging of the thalamic mediodorsal nucleus and pulvinar in schizophrenia and schizotypal personality disorder. Arch Gen Psychiatry 2001; 58:133–140Google Scholar
19. Hazlett EA, Buchsbaum MS, Byne W, et al: Three-dimensional analysis with MRI and PET of the size, shape, and function of the thalamus in the schizophrenia spectrum. Am J Psychiatry 1999; 156:1190–1199Google Scholar
20. Hazlett EA, Buchsbaum MS, Kemether E, et al: Abnormal glucose metabolism in the mediodorsal nucleus of the thalamus in schizophrenia. Am J Psychiatry 2004; 161:305–314Google Scholar
21. Kemether EM, Buchsbaum MS, Byne W, et al: Magnetic resonance imaging of mediodorsal, pulvinar, and centromedian nuclei of the thalamus in patients with schizophrenia. Arch Gen Psychiatry 2003; 60:983–991Google Scholar
22. Mitelman SA, Brickman AM, Shihabuddin L, et al: Correlations between MRI-assessed volumes of the thalamus and cortical Brodmann's areas in schizophrenia. Schizophr Res 2005; 75:265–281Google Scholar
23. Zhou SY, Suzuki M, Hagino H, et al: Decreased volume and increased asymmetry of the anterior limb of the internal capsule in patients with schizophrenia. Biol Psychiatry 2003; 54:427–436Google Scholar
24. Suzuki M, Nohara S, Hagino H, et al: Regional changes in brain gray and white matter in patients with schizophrenia demonstrated with voxel-based analysis of MRI. Schizophr Res 2002; 55:41–54Google Scholar
25. Hulshoff Pol HE, Schnack HG, Mandl RC, et al: Focal white matter density changes in schizophrenia: reduced inter-hemispheric connectivity. Neuroimage 2004; 21:27–35Google Scholar
26. Velakoulis D, Wood SJ, Smith DJ, et al: Increased duration of illness is associated with reduced volume in right medial temporal/anterior cingulate grey matter in patients with chronic schizophrenia. Schizophr Res 2002; 57:43–49Google Scholar
27. Giuliani NR, Calhoun VD, Pearlson GD, et al: Voxel-based morphometry versus region of interest: a comparison of two methods for analyzing gray matter differences in schizophrenia. Schizophr Res 2005; 74(2–3):135–147Google Scholar
28. Davis KL, Buchsbaum MS, Shihabuddin L, et al: Ventricular enlargement in poor-outcome schizophrenia. Biol Psychiatry 1998; 43:783–793Google Scholar
29. Shihabuddin L, Buchsbaum MS, Brickman AM, et al: Structural brain differences between poor-outcome and good-outcome patients with schizophrenia [Abstract]. Schizophr Res 2003; 60(suppl 1):206Google Scholar
30. Mitelman SA, Shihabuddin L, Brickman AM, et al: Cortical intercorrelations of temporal area volumes in schizophrenia. Schizophr Res 2005; 76:207–229Google Scholar
31. Buchsbaum MS, Shihabuddin L, Brickman AM, et al: Caudate and putamen volumes in good and poor outcome patients with schizophrenia. Schizophr Res 2003; 64:53–62Google Scholar
32. Mitelman SA, Shihabuddin L, Brickman AM, et al: MRI assessment of gray and white matter distribution in Brodmann's areas of the cortex in patients with schizophrenia with good and poor outcomes. Am J Psychiatry 2003; 160:2154–2168Google Scholar
33. Mitelman SA, Shihabuddin L, Brickman AM, et al: Volume of the cingulate and outcome in schizophrenia. Schizophr Res 2005; 72(2–3):91–108Google Scholar
34. Keefe RS, Mohs RC, Losonczy MF, et al: Characteristics of very poor outcome schizophrenia. Am J Psychiatry 1987; 144:889–895Google Scholar
35. Andreasen NC, Flaum M, Arndt S: The comprehensive assessment of symptoms and history (CASH): an instrument for assessing diagnosis and psychopathology. Arch Gen Psychiatry 1992; 49:615–623Google Scholar
36. Kay SR, Fiszbein A, Opler LA: The positive and negative syndrome scale (PANSS) for schizophrenia. Schizophr Bull 1987; 13:261–276Google Scholar
37. Spinks R, Magnotta VA, Andreasen NC, et al: Manual and automated measurement of the whole thalamus and mediodorsal nucleus using magnetic resonance imaging. Neuroimage 2002; 17:631–642Google Scholar
38. Shihabuddin L, Silverman JM, Buchsbaum MS, et al: Ventricular enlargement associated with linkage marker for schizophrenia-related disorders in one pedigree. Mol Psychiatry 1996; 1:215–222Google Scholar
39. Parent A: Carpenter's Human Neuroanatomy. Baltimore, Williams & Wilkins, 1996Google Scholar
40. Hazrati LN, Parent A: The striatopallidal projection displays a high degree of anatomical specificity in the primate. Brain Res 1992; 592(1–2):213–227Google Scholar
41. Meyer M: A study of efferent connections of the frontal lobe in the human brain after leucotomy. Brain 1949; 72:265–296Google Scholar
42. Shihabuddin L, Buchsbaum MS, Brickman AM, et al: Volume of the ventricles and cortical volume correlates in poor and good outcome schizophrenia, in reviewGoogle Scholar
43. Buchsbaum MS, Tang CY, Peled S, et al: MRI white matter diffusion anisotropy and PET metabolic rate in schizophrenia. Neuroreport 1998; 9:425–430Google Scholar
44. Shihabuddin L, Buchsbaum MS, Brickman AM, et al: Diffusion tensor imaging in Kraepelinian and non-Kraepelinian schizophrenia [abstract]. Biol Psychiatry 2001; 49:19SGoogle Scholar