What Does Epilepsy Tell Us About the Neural Correlates of Consciousness?
Abstract
Epilepsy and consciousness are intimately related. Epileptic seizures can cause impairment of consciousness, and the study of how this occurs has informed us about the neural mechanisms that underlie normal consciousness. Moreover, loss of consciousness during seizures increases injury risk and worsens health-related quality of life. The present review describes the current understanding of consciousness and its alterations during ictal events, with implications for the clinical management of patients with epilepsy and relevant neuro-philosophical issues.
DEFINING CONSCIOUSNESS
Defining consciousness poses an exciting intellectual challenge.1 Several definitions have been proposed, according to the following two aspects of consciousness: 1) a state of awareness, with capacity to respond and interact to external stimuli; or 2) a personal, internal, and qualitative experience of existence; but both are to be considered with certain caveats.2 Considering the assessment of consciousness in epilepsy using the “Ictal Consciousness Inventory (ICI),”3 1) would refer to the “level,” and 2) to the “content” of consciousness; these terms will be used throughout to describe each of the definitions.
Defining the level of consciousness as awareness of the environment lends itself to a continuum. The highest level would be a fully conscious state, with normal ability to respond. With decreasing level, a patient would pass through drowsiness and sleep and, ultimately, to unconsciousness, without any ability to respond. When a patient is reported to have had “loss of consciousness” during a seizure, it is usually this definition that the physician is referring to. The Glasgow Coma Scale4 is a widely-used method of assessing the level of consciousness; however, it is probably too simplistic for assessment of seizures.3 The ICI-Level Scale assesses the level of general awareness during epileptic seizures, querying general awareness of time, place, and the presence of others; comprehension of others' spoken words; verbal and nonverbal responsiveness; gaze control; forced attention; and voluntary initiative.
Defining the content of consciousness as an experience is much harder to complete and objectively analyze. When conscious, each person has an individual experience of the world and themselves. Thomas Nagel described the intrinsic subjectivity of conscious experiences by asking, by analogy, “What is it like to be a bat?”.5 This famous formulation reveals much about how we conceptualize consciousness. In considering what it is like to be a bat, we assume that there is something that it must be like to be a bat (including scanning the environment via ultrasound); therefore, it has a form of conscious personal experience that is accessible only through first-person perspective. By means of this analogy, Nagel argues that consciousness has essential to it a subjective character, which is accessible to first-person introspection, but cannot be objectified/made accessible to external observers. The ICI content scale assesses the subjective contents of consciousness during epileptic seizures, including dreamy states; derealization with regard to time and space; illusions and hallucinations; déjà vu, and unpleasant and pleasant ictal emotions.
Impairment of Consciousness in Epilepsy
Epilepsy is a chronic neurological condition characterized by a tendency toward unprovoked, stereotyped seizures.6 Seizures arise due to abnormal synchronous depolarization of cortical neurons, which may propagate and result in either activation or deactivation of other areas of neurons. Seizures can be divided into generalized (unable to identify the origin of activity to one hemisphere) or focal (localized origin of seizure onset). Focal seizures are subdivided into simple (without impairment of consciousness) or complex (with impairment). Conventional methods to differentiate between simple and complex may be limited by the patient's ability to respond (e.g., ictal aphasia, retrograde amnesia), which makes assessment difficult.7 The ICI is an important tool in allowing differentiation and better characterization of simple and complex focal seizures by identifying complex seizures that involve reduced content with near-normal level of consciousness.2,3
From the patient's perspective, the impairment of consciousness in epilepsy is central to the effect on quality of life, mostly because of the unpredictable nature of seizures.8–10 In some countries, if a patient only experiences seizures that do not affect consciousness, they are permitted to drive (e.g., some states in the U.S.). Impairment of consciousness during absence seizures may be the only symptom and will significantly affect employment, education, and daily activities.
For the researcher, epilepsy poses a unique opportunity to study consciousness because localized dysregulated neuronal activity results in varying degrees of consciousness.11 Furthermore, the information on consciousness that can be gleaned from epilepsy may benefit multiple neurological conditions associated with impaired consciousness. The seizure types that provide the best paradigms for studying consciousness in epilepsy are generalized tonic–clonic seizures (GTCS), absence seizures, and complex focal seizures (often part of temporal lobe epilepsy). Subtle differences in the neural correlates of consciousness impairment for each seizure have been used to aid research.12
Clinical Phenomenology
Idiopathic generalized epilepsy (IGE; also known as primary generalized epilepsy) encompasses absence seizures and GTCS. IGE seizures have diffuse involvement of the cortex, with rapid onset, such that the region of onset cannot be localized.12 These typically cause immediate loss of consciousness with greatly reduced level and little-or-no content (Figure 1).2
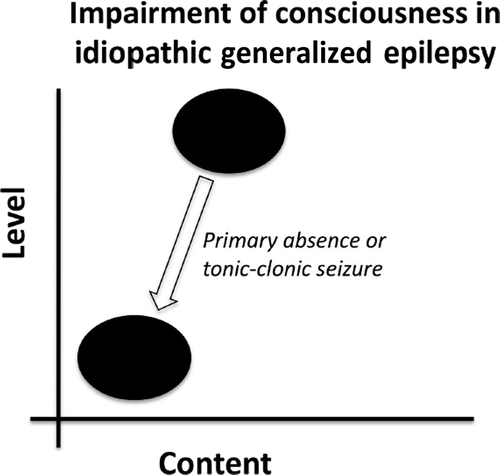
Primary absence seizures and generalized tonic–clonic seizures are characterized by low level and content of consciousness from a previously normal state.
GTCS have motor symptoms from increased activity in frontal lobe motor areas. They begin with a tonic phase, with flexion of the upper limbs and extension of the lower limbs and back, lasting 10–20 seconds. This is followed by a phase of clonic muscle activity, that consists of rapidly-alternating muscle relaxation and violent contraction lasting about 60–90 seconds. After the seizure, patients enter a period of post-ictal confusion, with reduced level and content of consciousness, usually manifested as confusion and drowsiness.
Absence seizures typically have minimal motor symptoms; for example, eye movement toward one side, with eyelid automatisms.13,14 In children, they may be mistaken for staring spells, as patients simply appear to be inattentive for 2–10 seconds. However, during a staring spell, it would be possible to get a child's attention, whereas during an absence seizure, a child becomes unresponsive.
Temporal-lobe epilepsy (TLE) seizures cause impairment of consciousness different from IGE. TLE seizures characteristically begin with a simple focal seizure known as an epileptic “aura.”15 Auras can take any form, but are often auditory, olfactory, or a “rising” epigastric sensation. These subjective experiences are usually stereotyped, such that each ictal event will begin in the same manner.16 Sometimes, patients find it difficult to describe their auras, as they can be either pleasant or, more frequently, profoundly unpleasant experiences. Auras usually do not affect the level of consciousness; however, they may result in increased conscious content from the intense subjective experience (Figure 2).3 Patients can become familiar with their aura, and, if level of consciousness is not impaired, they may be able to take precautions in case their seizure becomes generalized.
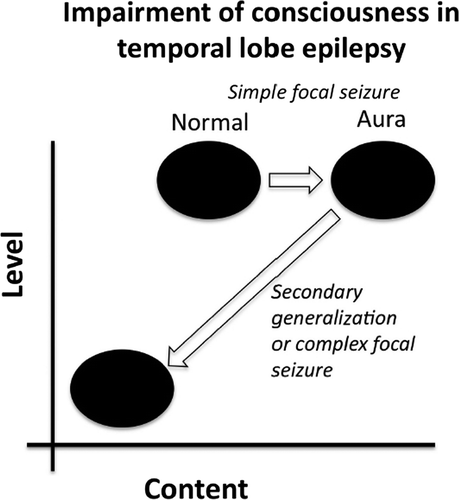
During a simple focal seizure, the general level of consciousness is maintained, but the epileptic aura causes increased content. If the seizure progresses to secondary generalization or to complex focal seizure, then both the level and the content of consciousness are greatly impaired.
Most TLE seizures are classified as complex focal seizures, which means that although the seizure focus is localized to one hemisphere, consciousness is impaired. This is due to the fact that some TLE seizures may show spread of the epileptiform activity to involve the cortex more diffusely, a phenomenon known as secondary generalization.17,18 Generalized seizures (primary or secondary) are characterized by a significantly reduced level of consciousness.12 Thus, TLE seizures may progress from increased content, with normal consciousness (during the aura), to a state of very low level and content of consciousness (when secondary generalization occurs; Figure 2). It has been shown that spread of activity to the frontal lobe can cause epileptic automatisms—repetitive, purposeless movements, such as lip-smacking.16,19
The clinical alterations of consciousness in the different seizure types are variable.3 For instance, patients with TLE seizures may have automatisms while experiencing ictal alterations in the content of consciousness, ranging from distorted perceptions to “déjà vu” experiences; however, because of anterograde amnesia from involvement of the hippocampus they may be unable to remember the event. History from the patient and an eyewitness is useful in determining the seizure type.
RESEARCH METHODS
The development of investigative techniques and imaging methods has determined the pace of the scientific study of the neural correlates of consciousness.13 Back in 1929, Berger first demonstrated the presence of spontaneous electrical activity by use of a crude electroencephalogram (EEG).20 Visualization of anatomy has progressed through computerized tomography (CT) and low-resolution MRI to 7-tesla MRI. Of particular importance is the use of functional imaging that allows the measurement of neuronal activity: functional MRI (fMRI), positron emission tomography (PET), and single-photon emission tomography (SPECT). They are based on the principle that brain metabolism is characterized by coupling of cerebral blood flow and oxygen-extraction/consumption.21 This can be measured using radioactive fluorine-labeled glucose, as in the case of FDG-PET, or hemoglobin-oxygenation (BOLD [blood oxygen level-dependent] signal), as in the case of fMRI.22
Neural Correlates of Consciousness
Overview
Specific areas of the CNS are involved in maintaining consciousness. The brainstem is the location for the reticular activating system, which maintains our level of general awareness. The reticular activating system's most important connection is with the thalamus, which, in turn, has widespread projections to the cortex. This pathway is responsible for regulation of cortical activity, which is necessary for the generation of conscious experiences. Several neurobiological theories attempt to combine these processes with definitions of consciousness into one explanation of our conscious experience.23,24 In the following sections, we discuss the main components of the neural correlates of consciousness in relation to epilepsy.
Ascending Reticular Activating System
The ascending reticular activating system (ARAS) is an archaic component of the CNS that is important for level of consciousness, or arousal. The ARAS is located in the caudal medulla and extends to rostral mesencephalon.25 It receives descending input from higher centers and ascending information from the spinal cord. The ARAS has multiple polysynaptic connections that project to several subcortical structures,26 especially medial thalamic nuclei.27 Activity in the ARAS is required for correct function of the thalamo-cortical relay that is thought to be the main mechanism by which the ARAS maintains arousal.
The role of the upper brainstem in arousal was first suggested around 1930 and confirmed by Bremer and others,28–30 who showed that transection through the midbrain caused a sleep-like state, whereas a cortico-medullary lesion had no effect on arousal. The term ARAS was coined by Moruzzi and Magoun, who found that appropriate activation of the reticular system can cause low-voltage high frequency EEG mimicking the awake state (Figure 3).25
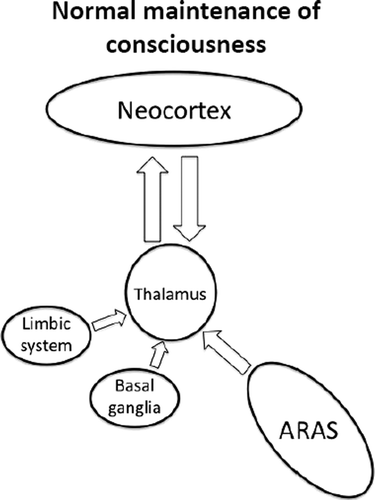
The ascending reticular activating system (ARAS) drives the thalamo-cortical relay, which is responsible for regulating cortical activity. The limbic system and basal ganglia also have a role in determining cortical activity.
Dysregulation of the ARAS function is thought to cause impairment of consciousness during epileptic seizures. Jasper found that involvement of the brainstem and diencephalon was required for impairment of consciousness in temporal lobe seizures.19 Interestingly, a number of studies have shown increased activity of the upper brainstem and thalamus during GTCS, absence, and complex focal seizures.13,15,31,32
Thalamo-Cortical Connections
There are multiple projections from the thalamus that are important for cortical activity.17,33 Absence seizures are characterized by a widespread 3- to 4-Hz “spike and wave” pattern on EEG that is thought to result from abnormal thalamo-cortical activity (Figure 4).34 This can be elicited by stimulation of the intralaminar thalamic nuclei,35,36 and most (although not all) absence seizures result in impairment of consciousness.13 It can therefore be inferred that regulated activity in these parts of the thalamus is required for maintaining consciousness.
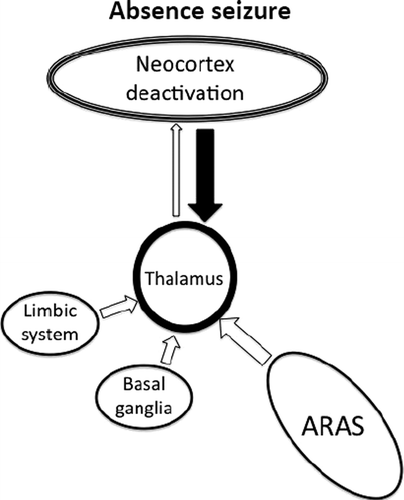
A defect in thalamo-cortical transmission causes alternating hyperactivation and excessive inhibition of the cortex. The net effect is deactivation of the regions most important for maintaining consciousness.
Spread to the thalamus is thought to be partly responsible for loss of consciousness during complex focal seizures of temporal lobe origin. There are established neuronal connections between the mesial temporal lobe and midline subcortical structures.18,37,38 Likewise, a functional correlation has been found between reduced consciousness level during temporal lobe seizures and hyperperfusion of the thalamus.15 Taken together, these findings support the theory that propagation from the temporal lobe is responsible for the impaired level of consciousness.
Cerebral Cortex and the Baseline State
The cerebral cortex is known as the location for higher cognitive processing; thus, it is not surprising that it is also central to consciousness. In generalized seizures, large areas of the cortex are diffusely involved, and consciousness is almost always impaired.39 However, others have noted generalized seizures with localized areas affected,40,41 and there are reports of patients who have suffered GTCS without loss of consciousness.42 Therefore, not all cortical regions appear to be equally involved in consciousness.
Although it has long been known that there is hemispheric dominance for many functions (e.g., speech), it has been traditionally difficult to elucidate consistent data on dominance for consciousness. Studies of patients who have undergone hemispherectomy show that only one hemisphere is needed for consciousness.43 Using amytal hemispheric inactivation, some researchers have suggested that the left side is dominant for consciousness.44 A more recent study, using video-electroencephalography (video-EEG), showed that consciousness is more frequently preserved if the right temporal lobe is affected, rather than the left.45 However, most of these studies used verbal response to assess consciousness, which is known to be biased toward the left side in the majority of patients.7 Of note, in one study, no hemispheric dominance was observed when noxious stimuli were used,46 and other work demonstrated bilateral cortical involvement to be necessary for impaired consciousness.47
Functional neuroimaging studies have consistently found that the cortical areas that are selectively deactivated during seizures are the fronto-parietal association areas, including the medial prefrontal cortex, the posterior cingulate cortex, and the precuneus.48–50 These regions are normally concerned with planning and association of visuospatial imagery with episodic memory.51,52 Raichle and colleagues53 noted that previous investigators had found these same areas to have a reduced cerebral oxygen-extraction fraction (i.e., they show a relative deactivation) when subjects shift from a baseline control state to a cognitively active state where they perform a non–self-directed task. This idea was developed further to suggest that the fronto-parietal association areas form part of the brain's “default state,” such that they are selectively activated in the absence of other activity. The same areas are known to be affected in diseases with impaired sensory awareness (e.g., Alzheimer's disease54) and are required for peripheral awareness. However, it is yet to be established whether these cortical regions are required for self-awareness, being meta-reflection, an integral part of consciousness.
Studies using SPECT imaging of GTCS have demonstrated that increased activity in the fronto-parietal association cortices correlates with loss of consciousness14 (Figure 5). This is in contrast with absence seizures, which are known to have reduced cortical activity in these regions.13 These findings support the importance of the medial prefrontal cortex, posterior cingulate cortex, and precuneus in maintaining the level of awareness, by showing that their abnormal activation or deactivation can impair the normal function of consciousness.
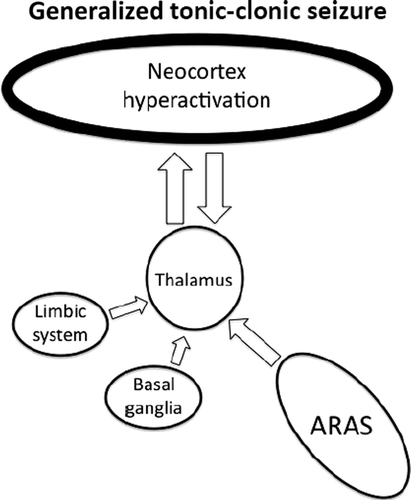
Loss of normal inhibitory mechanisms within the fronto-parietal association cortex results in hyperactivation that prevents normal functioning.
Norden and Blumenfeld55 have summarized the evidence on the brain mechanisms responsible for the alterations of consciousness during complex focal seizures of temporal lobe origin as the “network inhibition hypothesis.” The propagation of temporal lobe seizures to the thalamus interrupts the normal ARAS function, which, in turn, results in reduced activity of the fronto-parietal association cortices (Figure 6).
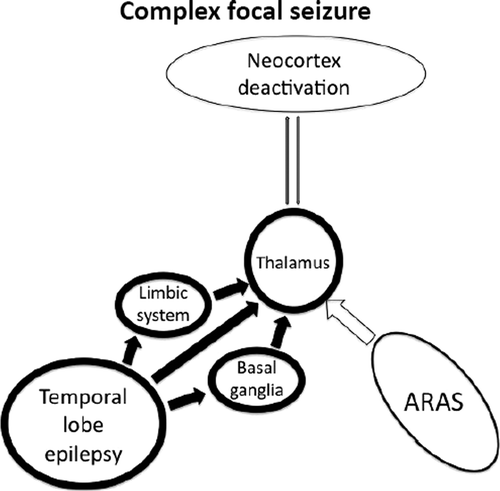
Epileptiform activity propagates from the temporal lobe to midline subcortical structures, disrupting thalamo-cortical relay (“network inhibition hypothesis”).
This idea is supported by the finding of slow-wave EEG activity in these cortical areas during complex focal seizures of the temporal lobe.56 Although the exact mechanism behind this phenomenon is unknown, modulation of inhibitory or excitatory inputs seems to be involved.12 The neural correlates of the alterations in level and content of consciousness in the most common seizure types affecting consciousness are summarized in Table 1.
![]() |
Implications for Clinical Practice
As definitions of consciousness are fine-tuned and our understanding of epilepsy widens, classification of epilepsy is modified.57 Is “generalized” an appropriate name for seizures that are known to have selective cortical effects? Furthermore, within the current setting of translational research, we should aim to use our understanding of the neurobiological mechanisms underlying consciousness to modulate the impact of epilepsy on patients. Pharmacological manipulation of neuronal function has been the mainstay of epilepsy management for many years. Drugs could thus be targeted to act on the regions specific to loss of consciousness, to block the pathways responsible for epileptiform activity propagation, or to influence receptors that have a key role in sustaining consciousness. This may permit maintenance of consciousness, even if seizure frequency could not be reduced.
A more invasive method of preserving consciousness in patients with epilepsy may be via use of deep brain stimulation (DBS). On the basis of previous experience in movement disorders, some research groups have begun to develop DBS approaches for epilepsy, vegetative state, and traumatic brain injury, with an aim of improving consciousness functions.58–60 Further work on this technique may ultimately improve management of seizures that affect consciousness in patients with refractory epilepsy.
The “Explanatory Gap” and Neurophilosophy
Our understanding of consciousness and its impairment has come a long way since the time when epilepsy was considered a manifestation of witchcraft.61 We have developed a sophisticated conceptual framework to address the “mind–body problem” within the realm of neuroscience. We have identified some of the key brain regions that are involved in generation and maintenance of conscious experience.62 However, modern neuroscience is still facing a conundrum: we know that specific cerebral activation-states correlate with the experience of subjective conscious contents, but the crucial link between the two is missing: this is known as the “explanatory gap.”63 Physicists have proposed quantum theories as a solution, where the movement of dissociated electrons (the physical domain) generates consciousness (the non-physical domain).64–66 Philosophers have analyzed evidence from psychophysiological experiments (e.g., Libet's famous demonstration of the neural time factor67) to conclude that perhaps the gap is not what we believe it to be because our perception of consciousness has been misled.68 In the 1970s, Benjamin Libet and his group conducted a series of investigations into human consciousness; one of these experiments demonstrated that the unconscious electrical processes in the brain, called “readiness potential” (Bereitschaftspotential), actually precedes conscious decisions to perform volitional acts, which are retrospectively felt to be consciously motivated by the subject. These experiments pose challenging questions to the neuroscientific quest for a comprehensive explanation of the brain mechanisms underpinning consciousness. Neuroscientific investigations in both experimental models and pathophysiological states in humans must aim to fill in the blanks between our current knowledge and what other disciplines tell us about conscious experience.
Neurophilosophy is the term used to describe the interplay between neuroscience and philosophy. Philosophy can help to inform neuroscientists and propose avenues for exploration, whereas advances in neuroscience can change the way that philosophers think about certain concepts.69 For instance, neurosciences approach consciousness within the conceptual framework of reductionist philosophy: that is, changes in conscious states will ultimately be explained via cellular and molecular mechanisms.70 It can be argued that reductionism leads to a deterministic theory of human behavior, where all our actions are determined by molecular events. This view, which is in line with the results of Libet's experiments, clearly poses a challenge to the philosophical concept of “free will.” Moreover, if consciousness is reduced to the activity of neurons, then it does not transcend the natural realm of physics and, by definition, fails to cross the boundary between metaphysical and physical: the main argument against classical dualism.11 The neurophilosophy of consciousness has important implications for future scientific inquiry in this area: according to the reductionist model, the continued development of electrophysiological and functional neuroimaging paradigms addressing brain activity in patients with (epilepsy-induced) alterations of consciousness will be one of the most promising ways forward in the consciousness-research arena.
SUMMARY
Consciousness can be operationally defined by its two dimensions: 1) the general level of awareness; and 2) the subjective contents of experience. Assessment of alteration of consciousness during epileptic seizures is important both for the diagnosis and the management of patients with epilepsy. There is evidence that the ARAS, thalamus, and fronto-parietal association cortices represent key areas for maintenance of normal consciousness. However, our current understanding leaves us with an explanatory gap, as the neural processes do not fully explain the experience of consciousness. It is likely that other regions of the brain are involved, including the limbic system,7,71 basal ganglia,72,73 and cerebellum. Further research is required to fully elucidate the mechanisms underlying impairment of consciousness in epilepsy, with the dual aim of improving patient management and understanding of the pathophysiology of disorders of consciousness.
1. : The Rediscovery of the Mind. Cambridge, MA, MIT Press, 1992Google Scholar
2. : Brain mechanisms of altered conscious states during epileptic seizures. Nat Rev Neurol 2009; 5:267–276Crossref, Medline, Google Scholar
3. : Measuring the level and content of consciousness during epileptic seizures: The Ictal Consciousness Inventory. Epilepsy Behav 2008; 13:184–188Crossref, Medline, Google Scholar
4. : Assessment of coma and impaired consciousness: a practical scale. Lancet 1974; 2:81–84Crossref, Medline, Google Scholar
5. : What is it like to be a bat? Philosophical Rev 1974; 83:435–450Crossref, Google Scholar
6.
7. : Consciousness as a neurological concept in epileptology: a critical review. Epilepsia 1986; 27(suppl 2):S14–S26Crossref, Medline, Google Scholar
8. : The risk of automobile accidents with seizures occurring while driving: relation to seizure type. Neurology 1987; 37:1613–1616Crossref, Medline, Google Scholar
9. : The consequences of uncontrolled epilepsy. CNS Spect 2004; 9:98–101Crossref, Medline, Google Scholar
10. : Relationships between seizure severity and health-related quality of life in refractory localization-related epilepsy. Epilepsia 2000; 41:760–764Crossref, Medline, Google Scholar
11. : Consciousness. Brain 2001; 124:1263–1289Crossref, Medline, Google Scholar
12. : Theories of impaired consciousness in epilepsy. Ann N Y Acad Sci 2009; 1157:48–60Crossref, Medline, Google Scholar
13. : Consciousness and epilepsy: why are patients with absence seizures absent? Prog Brain Res 2005; 150:271–286Crossref, Medline, Google Scholar
14. : Why do seizures cause loss of consciousness? Neuroscientist 2003; 9:301–310Crossref, Medline, Google Scholar
15. : Positive and negative network correlations in temporal lobe epilepsy. Cereb Cortex 2004; 14:892–902Crossref, Medline, Google Scholar
16. : Experiential phenomena of temporal lobe epilepsy: facts and hypotheses. Brain 1990; 113:1673–1694Crossref, Medline, Google Scholar
17. : The role of corticothalamic coupling in human temporal lobe epilepsy. Brain 2006; 129:1917–1928Crossref, Medline, Google Scholar
18. : Cerebral perfusion changes in mesial temporal lobe epilepsy: SPM analysis of ictal and interictal SPECT. Neuroimage 2005; 24:101–110Crossref, Medline, Google Scholar
19. : Some physiological mechanisms involved in epileptic automatisms. Epilepsia 1964; 5:1–20Crossref, Medline, Google Scholar
20. : Uber das elektrenkephalogramm des menschen. Arch Psychiat 1929; 87:527–570Crossref, Google Scholar
21. : Focal physiological uncoupling of cerebral blood flow and oxidative metabolism during somatosensory stimulation in human subjects. Proc Natl Acad Sci U S A 1986; 83:1140–1144Crossref, Medline, Google Scholar
22. : Brain magnetic resonance imaging with contrast dependent on blood oxygenation. Proc Natl Acad Sci U S A 1990; 87:9868–9872Crossref, Medline, Google Scholar
23. : The neuroanatomy of visual consciousness, in Consciousness: At The Frontiers of Neuroscience. Edited by Jasper HHDescarries LCastelucci VF. Philadelphia, PA, Lippincott-Raven, 1998, pp 229–243Google Scholar
24. : Consciousness and the integration of information in the brain, in Consciousness: At The Frontiers of Neuroscience. Edited by Jasper HHDescarries LCastelucci VF. Philadelphia, PA, Lippincott-Raven, 1998, pp 245–280Google Scholar
25. : Brainstem reticular formation and activation of the EEG. Electroencephalogr Clin Neurophysiol 1949; 1:455–473Crossref, Medline, Google Scholar
26. : Arousal systems and attention, in The Cognitive Neurosciences. Edited by Gazzaniga MS. Cambridge, MA, MIT Press, 1995, pp 703–720Google Scholar
27. : The neural basis of consciousness across the sleep–waking cycle, in Consciousness: At The Frontiers of Neuroscience. Edited by Jasper HHDescarries LCastelucci VF. Philadelphia, PA, Lippincott-Raven, 1998, pp 75–94Google Scholar
28. : Cerveau isolé et physiologie du sommeil. C R Senac Soc Biol 1929; 102:1235–1241Google Scholar
29. : Centrencephalic integrating system. Brain 1958; 81:231–234Crossref, Medline, Google Scholar
30. : Encephalitis Lethargica: Its Sequelae and Treatment. London, UK, Oxford University Press, 1931Google Scholar
31. : Dynamic time course of typical childhood absence seizures: EEG, behavior, and functional magnetic resonance imaging. J Neurosci 2010; 30:5884–5893Crossref, Medline, Google Scholar
32. : Epilepsy and consciousness, in The Neurology of Consciousness. Edited by Laureys STononi G. Amsterdam, The Netherlands, Elsevier, 2009, pp 247–260Crossref, Google Scholar
33. : Mechanism of thalamocortical augmentation and repetition. Am J Physiol 1943; 138:297–308Crossref, Google Scholar
34. : The electro-encephalogram in epilepsy and in conditions of impaired consciousness. Arch Neurol Psychiatry 1935; 34:1133–1148Crossref, Google Scholar
35. : Experimental studies on the functional anatomy of petit mal epilepsy. Assoc Res Nerv Ment Dis 1947; 26:272–298Google Scholar
36. : Highest-level seizures. Assoc Res Nerv Ment Dis Proc 1947; 26:252–271Google Scholar
37. : Mediodorsal thalamus plays a critical role in the development of limbic motor seizures. J Neurosci 1998; 18:9002–9009Crossref, Medline, Google Scholar
38. : Midline thalamic region: widespread excitatory input to the entorhinal cortex and amygdala. J Neurosci 2002; 22:3277–3284Crossref, Medline, Google Scholar
39. : Patterns of human local cerebral glucose metabolism during epileptic seizures. Science 1982; 218:64–66Crossref, Medline, Google Scholar
40. : Positron-emission tomography and autoradiographic studies of glucose utilization following electroconvulsive seizures in humans and rats. Ann N Y Acad Sci 1986; 462:263–269Crossref, Medline, Google Scholar
41. : Distribution of [14C]2-deoxyglucose after various forms and durations of status epilepticus induced by stimulation of a kindled amygdala focus in rats. Epilepsy Res 1991; 10:119–133Crossref, Medline, Google Scholar
42. : Painful generalised clonic and tonic–clonic seizures with retained consciousness. J Neurol Neurosurg Psychiatry 1997; 63:792–795Crossref, Medline, Google Scholar
43. : Physiologic observations following total hemispherectomy in man. Surgery 1955; 38:239–258Medline, Google Scholar
44. : Cerebral dominance for consciousness. Electroencephalogr Clin Neurophysiol 1997; 102:24–25Crossref, Google Scholar
45. : The localizing value of ictal consciousness and its constituent functions: a video-EEG study in patients with focal epilepsy. Brain 2002; 125:2691–2698Crossref, Medline, Google Scholar
46. : Consciousness and amnesia after penetrating head injury: neurology and anatomy. Neurology 1986; 36:178–187Crossref, Medline, Google Scholar
47. . Loss of consciousness in temporal lobe epilepsy: observations obtained with stereotaxis depth electrode recordings and stimulations, in Advances in Epileptology:
48. : The neural correlates of consciousness: an analysis of cognitive skill learning. Philos Trans R Soc Lond B Biol Sci 1998; 353:1889–1901Crossref, Medline, Google Scholar
49. : Top-down modulation of early sensory cortex. Cereb Cortex 1997; 7:193–206Crossref, Medline, Google Scholar
50. : The emotional modulation of cognitive processing: an fMRI study. J Cogn Neurosci 2000; 12(suppl 2):157–170Crossref, Medline, Google Scholar
51. : Focal features in patients with idiopathic generalized epilepsy. Epilepsy Res 2002; 50:293–300Crossref, Medline, Google Scholar
52. : Neural correlates of perceptual rivalry in the human brain. Science 1998; 280:1930–1934Crossref, Medline, Google Scholar
53. : A default mode of brain function. Proc Natl Acad Sci U S A 2001; 98:676–682Crossref, Medline, Google Scholar
54. : Metabolic reduction in the posterior cingulate cortex in very early Alzheimer's disease. Ann Neurol 1997; 42:85–94Crossref, Medline, Google Scholar
55. : The role of subcortical structures in human epilepsy. Epilepsy Behav 2002; 3:219–231Crossref, Medline, Google Scholar
56. : Decreased cerebral blood flow during seizures with ictal SPECT injections. Epilepsy Res 2000; 40:53–61Crossref, Medline, Google Scholar
57. : A proposed diagnostic scheme for people with epileptic seizures and with epilepsy: report of the ILAE Task Force on Classification and Terminology. Epilepsia 2001; 42:796–803Crossref, Medline, Google Scholar
58. : Behavioural improvements with thalamic stimulation after severe traumatic brain injury. Nature 2007; 448:600–603Crossref, Medline, Google Scholar
59. : Brain stimulation for epilepsy. Lancet Neurol 2004; 3:111–118Crossref, Medline, Google Scholar
60. : Deep brain stimulation therapy for the vegetative state. Neuropsychol Rehabil 2005; 15:406–413Crossref, Medline, Google Scholar
61. : A Disease Once Sacred: A History of the Medical Understanding of Epilepsy. Eastleigh, John Libbey & Co., Ltd., 2001Google Scholar
62. : Consciousness explained. Boston, MA, Little, Brown & Co, 1991Google Scholar
63. : The Conscious Mind. Oxford, UK, Oxford University Press, 1996Google Scholar
64. : Medical constraints on the quantum mind. J R Soc Med 2000; 93:571–575Crossref, Medline, Google Scholar
65. : Consciousness and Bose-Einstein condensates. New Ideas in Psychol 1989; 7:73Crossref, Google Scholar
66. : Shadows of the Mind. Oxford, UK, Oxford University Press, 1994Google Scholar
67. : The neural time factor in conscious and unconscious events. Ciba Found Symp 1993; 174:123–137Medline, Google Scholar
68. : There is no stream of consciousness. J Consciousness Stud 2002; 9:17–28Google Scholar
69. : The impact of neuroscience on philosophy. Neuron 2008; 60:409–411Crossref, Medline, Google Scholar
70. : Neural processes in the production of conscious experience, in The Science of Consciousness. Edited by Velmans M. London, UK, Routledge, 1996, pp 96–117Google Scholar
71. : The orchestration of conscious experience by subcortical structures. Biol Rev Camb Philos Soc 2009; 85:281–299Crossref, Medline, Google Scholar
72. : The roles of the cerebellum and basal ganglia in timing and error prediction. Eur J Neurosci 2002; 16:1609–1619Crossref, Medline, Google Scholar
73. : Neuropsychiatry of the basal ganglia. J Neurol Neurosurg Psychiatry 2002; 72:12–21Crossref, Medline, Google Scholar