Neurobiology of Adult ADHD: Emerging Evidence for Network Dysfunctions
Attention deficit hyperactivity disorder (ADHD) was once thought to be primarily a disorder of childhood, with symptoms remitting with maturation into adulthood.8–13 However, research began to gain traction in the 1990s demonstrating a significant proportion of children with ADHD continued to meet diagnostic criteria into adulthood although clinical presentation may change. In addition, some studies suggest that individuals can meet symptom criteria for ADHD as adults without meeting criteria as children.9,14 Current epidemiological studies have estimated the prevalence rate for adults with ADHD to be 2.5%−5%.12 It is important to note that adults with ADHD are not a homogeneous group. Dimensions of potential variability within the broad ADHD classification include comorbid diagnoses, gender, age, and predominate symptom presentation.8,10,13 These areas of variability, combined with other complicating factors (e.g. treated versus medication naïve) have added to the complexity of elucidating this disorder. Clinical variability may indicate the possibility of multiple developmental pathways.13 The flawed conception that ADHD is only a disorder of children lingers, and may contribute to the under recognition of this disorder in adults.10,12 Studies indicate that only a minority of adults with ADHD are currently receiving treatment.12 Comorbidities are common, particularly mood, anxiety, and substance use disorders (odds ratios of 3.9, 4.0, and 4.0 respectively).15 Furthermore, if comorbidities are present, those conditions are more likely to be the focus of treatment.11,12 Some environments are enriched with adults who have ADHD. A recent meta-analysis reported a prevalence of 26.2% in incarcerated adults, ten times the rate in the general population.16 While the etiology of this disorder is still not fully understood, studies of heritability suggest a very strong genetic component (∼0.77).17 Other developmental pathways such as exposure to teratogens may also be important.17
Clinical Symptoms and Neuropsychological Deficits
The symptoms of childhood ADHD are commonly divided into two overarching categories: inattentive and hyperactive/impulsive. Studies indicate that the hyperactive/ impulsive cluster of symptoms tends to wane with age relative to the more enduring inattentive cluster.8,9,18,19 It has also been suggested that expression of hyperactivity in adults may become internalized as restlessness or inner tension, which may be misinterpreted as anxiety.9,12 In addition, hyperactive/impulsive symptoms may become less apparent due to a changing environment with different behavioral constraints. A recent study that divided impulsivity into four dimensions (urgency, premeditation, perseverance, sensation seeking) reported that compared with controls, medication naïve adults with ADHD had higher urgency (tendency to act rashly), lower premeditation (ability to consider consequences of actions), and lower perseverance (ability to stay on task when bored).20 A very common behavior reported by adults with ADHD that is not specifically mentioned in the DSM-5 diagnostic criteria is evidence of risky driving, (e.g. elevated rates of motor vehicle collisions, citations, and arrests).21 Studies using driving simulators indicate contributions from both impaired attentional control and greater fatigue under monotonous conditions.21 One study also reported significantly elevated daytime sleepiness in one-third of adults with ADHD that was associated with impaired driving simulator performance.22
Understanding the most commonly identified neuropsychological deficits on formal testing in adult ADHD can inform the neuroanatomical study of this disorder. It should be noted that a significant portion of those who meet diagnostic criteria for ADHD perform within normal limits on neuropsychological testing. The rate of false negatives varies based on the strength of the test administered. Tests are administered in a controlled environment (e.g. limiting extraneous stimuli) and are typically relatively brief (e.g. limiting fatigue). As a result, impairments may be underestimated.23 A meta-analysis examining 33 studies of adults with ADHD compared with controls reported the largest impairments were in the domains of attention, memory, and behavioral inhibition, based on effect sizes.24 In brief, effect size is an objective measure of the magnitude of an observed difference. In contrast, a significance test determines only whether the null hypothesis (no difference between groups) should be rejected. Effect size is useful for determining whether a statistically significant difference has practical (i.e. clinical) relevance. This metric is also used in meta-analyses to standardize group differences observed across studies, enabling results to be pooled and analyzed together. Weighted effect sizes are commonly interpreted with cutoffs of 0.20, 0.50, and 0.80 for small, medium and large respectively.
Attentional deficits are the hallmark of ADHD and are the most likely symptoms to persist into adulthood.8 A meta-analysis of tasks involving attention found effect sizes in the medium range (0.51−0.76).24 Attention is often assessed using a measure of continuous performance (e.g. Conner’s Continuous Performance Task). Increases in errors of omission and reaction time variability (standard error) characterize ADHD. A meta-analysis of studies found deficits in adults with ADHD were much more pronounced on measures of complex attention (medium to large effect sizes) compared with simple attention (small to medium effect sizes).25 Studies in adult ADHD have examined multiple aspects of memory (e.g. working, short term, long term, and metamemory (knowledge of one’s own memory capabilities)). Meta-analyses indicate adults with ADHD have greater impairment in tasks of verbal memory (pooled effect size=−0.56) relative to those of visual or figural memory (pooled effect size=−0.18).26 A meta-analysis examining studies of long term memory found that adults with ADHD had similar deficits in recall and recognition trials, suggesting that the impairment is in encoding rather than in retrieval.27 This finding highlights the challenges of disentangling memory deficits from attentional deficits. Research on metamemory indicates that adults with ADHD had the same level of accuracy compared with controls in predicting their memory performance.28 Meta-analyses confirm that deficits in behavioral inhibition are reliably found in adults with ADHD.24,29 Compared with controls, adults with ADHD are more susceptible to proactive interference for semantic, but not spatial tasks.30 Furthermore, they have a higher rate of incorrect responses on implicit sequence learning tasks.31
Neurobiology
Over the decades, multiple neurobiological models have been proposed for the symptoms of ADHD.8,13,32–35 The behavioral neuroenergetics theory postulates neuronal energy insufficiency as a result of inadequate production of lactate by astrocytes.32,34 State (vigilance) regulation theories hypothesize that the core deficit in ADHD is impaired regulation of activation state (arousal).33,36 Some symptoms are attributed to dysfunctional fluctuations in arousal (e.g. deficits in sustained attention) whereas others are a result of autoregulatory efforts to reach stability by increasing external stimulation (e.g. hyperactivity, sensation seeking). An alternative theory is impaired top-down (central executive) regulation of behavior, which gives rise to deficits in multiple domains.13,35 One variant of this theory specifically implicates inconsistent suppression of default mode network (DMN) activity.34 Impaired bottom-up (limbic) regulation of reward-related processing has also been proposed.13,33,35 Multiple pathway theories postulate that individually variable deficits in multiple core functions (e.g. timing, inhibiting responses, tolerating delay) give rise to the highly diverse symptom expression that characterizes ADHD.13,33 Delayed development within the later maturing areas of the brain has been suggested as a causal mechanism in some theories.13,34
Structural Neuroimaging
Several recent reviews have detailed structural imaging in children with ADHD.37–40 Multiple studies have shown smaller total brain volume as well as smaller volume in a variety of subcortical (e.g. amygdala, thalamus, basal ganglia, cerebellum) and cortical (e.g. prefrontal, premotor, anterior cingulate, temporal, anterior insula, supplementary motor, primary sensorimotor) areas compared with typically developing controls. A review of structural imaging studies in adults with ADHD noted abnormalities (e.g. reduced volume, reduced gray matter density, reduced cortical thickness) in many of the same regions (e.g. superior frontal, orbitofrontal, anterior cingulate, inferior frontal, dorsolateral prefrontal, posterior cingulate, temporoparietal, cerebellar, and occipital regions).37 Two recent meta-analyses that included studies in both children and adults reported significantly smaller global gray matter volume and volume within portions of the basal ganglia in children with ADHD compared with controls, but not when adults with ADHD were compared with controls.41,42 One meta-analysis reported that adults with ADHD had smaller gray matter volumes compared with controls only in anterior cingulate cortex (bilateral).42 The other found that both increasing age and medication use were associated with normalization of group differences.41
The theory that ADHD is a disorder of developmental delay in brain maturation has received support from a large longitudinal study comparing a cohort with childhood diagnosis of ADHD to controls.1,43,44 For most measures, values differed in early childhood between groups (e.g. ADHD group had smaller total cerebral and cerebellar volumes) and the groups had very similar growth trajectories (developmental curves). The ADHD group reached peak cortical thickness (a measure of brain maturation) significantly later than controls in most areas (median age 10.5 years versus 7.5 years).1 The lateral prefrontal region (middle prefrontal gyrus) had the longest delay (approximately 5 years).1 Similarly, portions of the basal ganglia (primarily caudate) were smaller from the earliest time point in the ADHD group by both volume and surface area measures.44 These differences persisted into adolescence indicating similar growth trajectories. In contrast, the ventral striatum was initially similar but the groups had opposite trajectories. Those with ADHD demonstrated a contraction of the ventral striatal surface area with age, whereas the control group had an expansion.44 This finding was not confirmed in two recent large cross-sectional studies utilizing a different method of automated segmentation.45,46 The study of a cohort of adults with ADHD from childhood found no main effect of ADHD diagnosis on the volume of caudate, putamen or nucleus accumbens (ventral striatum).45 The study comparing older children and young adults with ADHD (mean age 17.06 years), their unaffected siblings (mean age 17.52 years), and controls (mean age 16.66 years) found that volumes of subcortical structures (nucleus accumbens, caudate, putamen, globus pallidus, amygdala, thalamus, hippocampus, brainstem) did not differ.46 The ADHD group had significantly smaller total brain volume (2.5%) and gray matter volume (3%) than controls, but white matter volumes did not differ. Unaffected siblings were found to have brain volumes (total and gray matter) intermediate between the ADHD and control groups, adding support to the idea of a strong genetic component in this disorder.46
Other recent cross-sectional studies also support normalization of volumetric measures, as no significant differences were found in total or regional gray matter or white matter volumes between adults with ADHD and controls.45,47,48 Although some small areas differed between groups, there was little agreement across studies in localizations. In an effort to address the potentially confounding effects of medications, two studies have compared medication naïve adults with ADHD to controls.48,49 One study assessed volumetric differences within six predefined regions of interest (anterior cingulate, dorsolateral prefrontal, orbitofrontal and inferior parietal cortices, caudate, cerebellum).49 Only the smaller cerebellar volume in the ADHD group compared with controls remained significant after adjustment for multiple comparisons. The other study utilized a newly validated method of optimally-discriminative voxel-based analysis.48 Areas with lower gray matter volume (right angular gyrus) and higher gray matter volume (right supplementary motor area and right superior frontal gyrus) were identified in the ADHD group compared with controls, but no abnormality survived correction for multiple comparisons. A later study from the longitudinal cohort as young adults (mean 23.8 years) reported differences in the trajectory of cortical thinning by adult diagnostic grouping (remitted ADHD versus persistent ADHD).2 The rate of thinning in several cortical areas correlated positively with adult symptom expression (more symptoms associated with greater thinning) (Figure 1). Thinning associated with inattention had a larger anatomic extent than thinning associated with hyperactivity-impulsivity. Compared with controls, the rate of cortical thinning was slower in the remitted ADHD group, resulting in normalization with age. This is consistent with a smaller cross-sectional study of adults with childhood ADHD which reported multiple areas in which cortex was thinner in the persistent ADHD group compared with the remitted ADHD group (Figure 1) although these did not survive correction for multiple comparisons.3
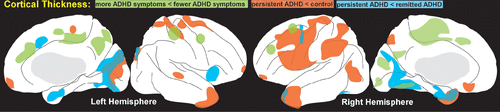
FIGURE 1. Changes in cortical thickness provide one measure of brain maturation. A large longitudinal study found that for most areas of cortex, children with attention deficit hyperactivity disorder (ADHD) reach peak cortical thickness several years later than typically developing children, supporting presence of developmental delay.1 The rate of cortical thinning also differed between the group who continued to meet diagnostic criteria into adulthood (persistent ADHD) and those who did not (remitted ADHD).2 Areas of cortex in which the rate of thinning correlated with adult symptom level (green, more symptoms associated with more thinning) are approximated on medial and lateral simplified representations of cortex.2 An earlier study also identified multiple areas in which cortex was thinner in adults with persistent ADHD compared with controls (orange).3 In addition, this study noted some areas of thicker cortex in remitted ADHD when compared with persistent ADHD (blue).
As detailed in recent reviews, studies using diffusion tensor imaging (DTI) have reported a diversity of white matter areas (e.g. fronto-striatal, cingulate, fronto-parietal, fronto-cerebellar, parieto-occipital tracts) with either increased or decreased metrics (fractional anisotropy, FA; mean, radial and/or axial diffusivity, MD, RD, AD) associated with ADHD.37–40,50 A recent meta-analysis (nine studies; 53 foci of altered FA) identified only five significant clusters (right anterior corona radiata and forceps minor, left cerebellar white matter, bilateral internal capsule).50 The most common findings in more recent DTI studies in adults with ADHD compared with controls are lower FA and/or higher MD within the orbitofrontal and temporal white matter (including portions of the uncinate fasciculus, inferior longitudinal fasciculus, inferior fronto-occipital fasciculus, and external capsule).51–53 DTI metrics within the uncinate and inferior fronto-occipital fasculi correlated with more inattentive symptoms in one study.53 Other studies have reported changes in the opposite direction (higher FA and/or lower MD) within these areas.48,54 Several studies have also reported altered metrics in more dorsal areas of the cerebral white matter.48,52–56
There are some intriguing differences between comparisons based on diagnostic grouping (adult ADHD versus control) and symptom measures. A recent study comparing older children and young adults with ADHD and controls reported multiple clusters in which either FA or MD were lower in the ADHD group compared with controls.57 In contrast, greater symptom severity within the ADHD group was associated with widespread clusters in which FA was higher.57 In another study of this cohort, DTI of adolescents with persistent ADHD, remitted ADHD, and controls were compared with identify white matter differences that correlated with symptom reduction.55 The only area of significant difference across groups was in the left corticospinal tract, in which lower FA and higher MD were associated with greater improvement in hyperactive/impulsive symptoms.55 In contrast, a study comparing young adults with persistent ADHD or remitted ADHD to controls reported an association between lower FA in this area and higher inattentive symptoms.53 Neither of these studies reported significant differences between remitted ADHD and controls.53,55
Functional Imaging
Task-activated functional imaging is utilized to probe for differences between groups in brain areas supporting specific domains of function. Two recent meta-analyses of task-activated functional magnetic resonance imaging (fMRI) studies reported that patterns of hypo- and hyper-activations differed between adults with ADHD and children with ADHD when compared with controls. One meta-analysis utilized activation likelihood estimation and combined studies that included a range of tasks (inhibition, vigilance/attention, working memory).58 Children with ADHD had more widespread differences (hypoactivations in frontal, parietal and temporal cortices and putamen; hyperactivations in angular and middle occipital gyri, posterior cingulate and midcingulate cortices) than adults with ADHD (hypoactivations in middle frontal and precentral gyri and central sulcus; hyperactivations in angular and middle occipital gyri).58 This study also related all identified voxels to seven predetermined networks (DMN, frontoparietal, dorsal attention, ventral attention, limbic, visual and sensorimotor). Children with ADHD displayed the most hypoactivated voxels in the frontoparietal (39%) and ventral attention (44%) networks and hyperactivated voxels in the default (37%), ventral attention (23%), and somatomotor (22%) networks. In contrast, for adults with ADHD almost all (97%) of the hypoactivated voxels were located in the frontoparietal network and no hyperactivated voxels were assigned to the somatomotor network.58 These differences are consistent with some functional normalization as children with ADHD mature into adulthood. The other meta-analysis, which utilized effect size signed differential mapping, reported differences in the areas that were hypoactivated in children and adults with ADHD compared with controls on tasks requiring inhibition.59 Adults with ADHD exhibited significantly reduced activation only in inferior frontal cortex and thalamus. Children with ADHD had significantly reduced activation in supplementary motor cortex and the basal ganglia.
Two recent fMRI studies in a cohort of adults with childhood diagnosis of ADHD (both persistent and remitted) utilized cued go/no-go tasks to assess both activations and functional connectivity compared with controls.4,5 As would be expected, the study using an emotion recognition paradigm found that the ADHD group (persistent or partially remitted ADHD) performed significantly worse on both no-go (fewer correct inhibitions, a measure of cognitive control) and go (fewer correct responses) trials.5 There were no significant between group differences in the areas activated by cognitive control (correct no-go trials minus correct go trials). The area within right dorsolateral prefrontal cortex (dlPFC) maximally activated in that analysis was used as a seed to assess whole brain connectivity associated with cognitive control. The ADHD group had lower connectivity to multiple areas (left putamen, left inferior frontal gyrus, and bilateral subgenual cingulate cortex) compared with controls (Figure 2), but connectivity did not correlate with symptom severity.5 The study that used a cued reaction time task reported that the ADHD group as a whole (persistent ADHD and remitted ADHD) had a similar pattern of cue related activation (cued trials minus no cue trials, response preparation) compared with controls, but lower activation in multiple areas (dlPFC, anterior cingulate cortex, supplementary motor area, thalamus and inferior parietal lobe).4 The ADHD group also had lower response preparation related functional connectivity between the seed in right thalamus and the brainstem. Contrary to expectations, there were no significant differences in thalamo-cortical system activation between the persistent and remitted ADHD groups. However, the persistent ADHD group had lower response preparation related connectivity between thalamus and prefrontal regions compared with the remitted ADHD group (Figure 2).4 A recent electrophysiological study used a cued go/no-go task (auditory) to test the hypoarousal model of ADHD. They examined the contingent negative variation (CNV), a late component of the event related potential that reflects cortical arousal.60 Groups performed similarly, but the ADHD group had lower CNV amplitude at central sites during response preparation.60 The authors noted that reduced CNV amplitude is consistent with presence of cortical hypoarousal in adult ADHD and thus might have potential as a biomarker, although they acknowledged that CNV amplitude did not correlate significantly with ADHD symptoms. A longitudinal study demonstrated that CNV amplitude remained significantly reduced in individuals with ADHD into young adulthood, although most of the group no longer met diagnostic criteria.61 The authors suggested that this evidence supports reduced CNV amplitude as a developmentally stable biomarker for ADHD, likely reflecting impairments in time processing and cognitive preparation. Another electrophysiological study reported evidence indicating impairments in earlier (attentional and motor response selection) rather than later (motor execution) processes in adults with childhood onset ADHD.62 Adults with ADHD have also been shown to have significantly lower sensory gating (difference between the auditory evoked potentials to the first and second tone) than controls, suggesting impaired ability to filter incoming information.63
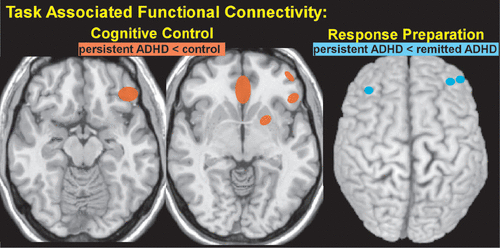
FIGURE 2. Two recent functional magnetic resonance imaging (fMRI) studies have used cued reaction time tasks to assess differences between adults with a childhood diagnosis of ADHD and controls in task-related activations and functional connectivity.4,5 Left. One study used the area with the highest level of activation associated with cognitive control (right dorsolateral prefrontal cortex) as the seed to assess functional connectivity associated with cognitive control.5 The ADHD group had lower connectivity than the control group to multiple areas (orange, left putamen, left inferior frontal gyrus, and bilateral subgenual cingulate cortex), but connectivity was not correlated with symptom severity. Right. The other study assessed thalamocortical functional connectivity and brain activations associated with response preparation.4 The persistent ADHD group had lower connectivity compared with the remitted ADHD group from the seed region in thalamus to several areas in prefrontal cortex. The locations of the cluster centers (blue, bilateral frontopolar cortex and left dorsolateral prefrontal cortex) are approximated onto a dorsal view of the cortical surface. An unexpected finding was that there were no significant differences between the remitted ADHD group and persistent ADHD in brain activations associated with response preparation.
Resting state functional imaging supports simultaneous assessment of multiple brain networks. Networks (interacting regions) are identified based on the time course of signal changes. High temporal correlation is considered to indicate high functional connectivity. The default mode network (DMN) is composed of regions of the brain that are most active during resting conditions, with very little activity during performance of tasks (anticorrelated with task-activated regions).64 Brain regions commonly included are the inferior parietal lobules, the posterior cingulate/retrosplenial cortex (PCC), and the medial PFC.65 The DMN interference hypothesis postulates that lapses in attention during task performance arise from failures in suppression of these areas.66 Several studies utilizing resting state fMRI from a large multisite ADHD cohort (age range 7 to 22 years) have reported alterations in network metrics consistent with this hypothesis.66–68 The study utilizing network contingency analysis reported reduced connectivity within the DMN and between the DMN and the frontoparietal (executive control) and ventral attention (salience) networks compared with controls.66 Regression modeling suggested maturational lag in connectivity development.67 Greater severity of inattention was associated with longer lag in maturation. The study using graph theory analysis to assess connectivity at a given path length (number of steps) from seeds in primary sensory cortices reported that ADHD was associated with increased short path (one or two steps) connections to multimodal cortices, and long path connections to medial PFC relative to controls.68 ADHD was associated with reduced short path connections to frontoinsular and anterior cingulate cortices and reduced long path connections to dlPFC and inferior parietal cortices.68
Recent studies in adults suggest normalization of some of these differences with remission of ADHD but not others. A study in adults with a childhood diagnosis of ADHD reported that persistent ADHD was associated with reduced connectivity within the DMN (medial PFC and PCC) compared with both controls and remitted ADHD, which did not differ (Figure 3).6 However, the expected negative connectivity (anticorrelation) between the dlPFC and medial PFC was present in the control group, but not in either the persistent or remitted ADHD groups. The authors of this study suggested this may indicate executive functioning weakness, as the ADHD groups were similarly impaired on neuropsychological testing.6 Another study that included two groups of medication naïve adults with ADHD reported increased connectivity between the DMN and a small region within left lateral PFC based on independent component analysis (Figure 3).7 In addition, higher levels of resting state activity within that area of PFC were associated with lower levels of performance on an attentional tasks. Increased connectivity between some DMN areas in ADHD relative to controls has also been reported.69
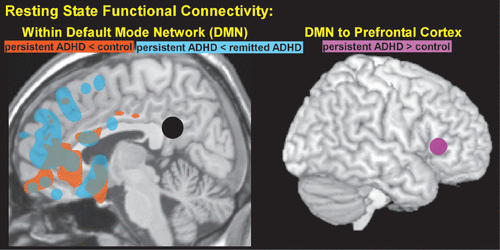
COVER and FIGURE 3. Left. A resting state fMRI study in adults with a childhood diagnosis of ADHD reported significantly reduced functional connectivity within the default mode network in persistent ADHD.6 The areas in which either remitted ADHD (blue) or controls (orange) had greater connectivity from the seed region (black, posterior cingulate cortex) compared with persistent ADHD are approximated on a midline MRI.6 This study also reported that neither ADHD group exhibited the expected negative functional connectivity between the other default mode network seed region (medial prefrontal cortex) and dorsolateral prefrontal cortex . Right. A resting state fMRI study in medication naive adults with ADHD reported positive connectivity between an area in lateral prefrontal cortex and default mode network areas identified by independent component analysis.7 The location and extent are approximated on a lateral view of the cortical surface (violet). Higher resting state activity in this region was inversely correlated with attentional performance (higher activity, lower performance).
Conclusions
In conclusion, current evidence indicates that ADHD can continue into adulthood, although symptom configuration may well change. Although studies vary widely in methodology and subject populations, both structural and functional imaging support changes in neurobiological substrates with advancing maturity. In addition, normalization of many imaging abnormalities appears to occur with symptom diminution. As always, it is important to acknowledge the limitations of this type of research. Most studies are cross-sectional and include relatively small numbers. Thus, results can be confounded by low statistical power and differences in study populations, as well as the known high interindividual differences in brain volumes.38,70 Although meta-analytic approaches have been developed to address some of these issues, these are better for testing robustness of positive results of the included studies than for improving detection of false-negative findings.38
1 : Attention-deficit/hyperactivity disorder is characterized by a delay in cortical maturation. Proc Natl Acad Sci USA 2007; 104:19649–19654Crossref, Medline, Google Scholar
2 : Trajectories of cerebral cortical development in childhood and adolescence and adult attention-deficit/hyperactivity disorder. Biol Psychiatry 2013; 74:599–606Crossref, Medline, Google Scholar
3 : Brain gray matter deficits at 33-year follow-up in adults with attention-deficit/hyperactivity disorder established in childhood. Arch Gen Psychiatry 2011; 68:1122–1134Crossref, Medline, Google Scholar
4 : Thalamo-cortical activation and connectivity during response preparation in adults with persistent and remitted ADHD. Am J Psychiatry 2013; 170:1011–1019Crossref, Medline, Google Scholar
5 : Emotional bias of cognitive control in adults with childhood attention-deficit/hyperactivity disorder. Neuroimage Clin 2014; 5:1–9Crossref, Medline, Google Scholar
6 : Brain differences between persistent and remitted attention deficit hyperactivity disorder. Brain 2014; 137:2423–2428Crossref, Medline, Google Scholar
7 : An independent components and functional connectivity analysis of resting state fMRI data points to neural network dysregulation in adult ADHD. Hum Brain Mapp 2014; 35:1261–1272Crossref, Medline, Google Scholar
8 : Attention-deficit/hyperactivity disorder: diagnosis, lifespan, comorbidities, and neurobiology. J Pediatr Psychol 2007; 32:631–642Crossref, Medline, Google Scholar
9 : Structure and diagnosis of adult attention-deficit/hyperactivity disorder: analysis of expanded symptom criteria from the Adult ADHD Clinical Diagnostic Scale. Arch Gen Psychiatry 2010; 67:1168–1178Crossref, Medline, Google Scholar
10 : European consensus statement on diagnosis and treatment of adult ADHD: The European Network Adult ADHD. BMC Psychiatry 2010; 10:67Crossref, Medline, Google Scholar
11 : The unmet needs of all adults with ADHD are not the same: a focus on Europe. Expert Rev Neurother 2014; 14:799–812Crossref, Medline, Google Scholar
12 . Underdiagnosis of attention-deficit/hyperactivity disorder in adult patients: a review of the literature. Prim Care Companion CNS Disord. 2014;16(3).Medline, Google Scholar
13 : Attention deficit hyperactivity disorder. Curr Top Behav Neurosci 2014; 16:235–266Crossref, Medline, Google Scholar
14 . Is Adult ADHD a Childhood-Onset Neurodevelopmental Disorder? Evidence From a Four-Decade Longitudinal Cohort Study. Am J Psychiatry. 2015:appiajp201514101266.Crossref, Medline, Google Scholar
15 : Cross-national prevalence and correlates of adult attention-deficit hyperactivity disorder. Br J Psychiatry 2007; 190:402–409Crossref, Medline, Google Scholar
16 : A meta-analysis of the prevalence of attention deficit hyperactivity disorder in incarcerated populations. Psychol Med 2015; 45:247–258Crossref, Medline, Google Scholar
17 : Towards conceptualizing a neural systems-based anatomy of attention-deficit/hyperactivity disorder. Dev Neurosci 2009; 31:36–49Crossref, Medline, Google Scholar
18 : ADHD in DSM-5: a field trial in a large, representative sample of 18- to 19-year-old adults. Psychol Med 2015; 45:361–373Crossref, Medline, Google Scholar
19 :
20 : A multidimensional approach of impulsivity in adult attention deficit hyperactivity disorder. Psychiatry Res 2015; 227:290–295Crossref, Medline, Google Scholar
21 : Adult attention-deficit/hyperactivity disorder and driving: why and how to manage it. Curr Psychiatry Rep 2011; 13:345–350Crossref, Medline, Google Scholar
22 : Excessive daytime sleepiness in adult patients with ADHD as measured by the Maintenance of Wakefulness Test, an electrophysiologic measure. J Clin Psychiatry (Epub ahead of print, Jan 6, 2015)Medline, Google Scholar
23 : Working memory deficits in adults with attention-deficit/hyperactivity disorder (ADHD): an examination of central executive and storage/rehearsal processes. J Abnorm Psychol 2013; 122:532–541Crossref, Medline, Google Scholar
24 : Neuropsychology of adults with attention-deficit/hyperactivity disorder: a meta-analytic review. Neuropsychology 2004; 18:485–503Crossref, Medline, Google Scholar
25 : Attention deficit hyperactivity disorder (ADHD): gender- and age-related differences in neurocognition. Psychol Med 2009; 39:1337–1345Crossref, Medline, Google Scholar
26 : Neuropsychological performance in adult attention-deficit hyperactivity disorder: meta-analysis of empirical data. Arch Clin Neuropsychol 2005; 20:727–744Crossref, Medline, Google Scholar
27 : Long-Term Memory Performance in Adult ADHD: A Meta-Analysis. J Atten Disord (Epub ahead of print, Nov 14, 2013)Medline, Google Scholar
28 : Isolating metamemory deficits in the self-regulated learning of adults with ADHD. J Atten Disord 2012; 16:650–660Crossref, Medline, Google Scholar
29 : Executive functioning in adult ADHD: a meta-analytic review. Psychol Med 2005; 35:1097–1108Crossref, Medline, Google Scholar
30 : Inhibitory control of proactive interference in adults with ADHD. J Atten Disord 2007; 11:141–149Crossref, Medline, Google Scholar
31 : Impaired Behavioral Inhibition in Implicit Sequence Learning in Adult ADHD. J Atten Disord (Epub ahead of print, Nov 27, 2012)Google Scholar
32 : A behavioral neuroenergetics theory of ADHD. Neurosci Biobehav Rev 2013; 37:625–657Crossref, Medline, Google Scholar
33 : Hyperactivity and Motoric Activity in ADHD: Characterization, Assessment, and Intervention. Front Psychiatry 2014; 5:171Crossref, Medline, Google Scholar
34 : Working memory and intraindividual variability as neurocognitive indicators in ADHD: examining competing model predictions. Neuropsychology 2014; 28:459–471Crossref, Medline, Google Scholar
35 : Neuroanatomic and cognitive abnormalities in attention-deficit/hyperactivity disorder in the era of ‘high definition’ neuroimaging. Curr Opin Neurobiol 2015; 30:1–8Crossref, Medline, Google Scholar
36 : The vigilance regulation model of affective disorders and ADHD. Neurosci Biobehav Rev 2014; 44:45–57Crossref, Medline, Google Scholar
37 : A review of fronto-striatal and fronto-cortical brain abnormalities in children and adults with Attention Deficit Hyperactivity Disorder (ADHD) and new evidence for dysfunction in adults with ADHD during motivation and attention. Cortex 2012; 48:194–215Crossref, Medline, Google Scholar
38 : Neurobiology of ADHD From Childhood to Adulthood: Findings of Imaging Methods. J Atten Disord (Epub ahead of print, Oct 4, 2013)Medline, Google Scholar
39 : Imaging the ADHD brain: disorder-specificity, medication effects and clinical translation. Expert Rev Neurother 2014; 14:519–538Crossref, Medline, Google Scholar
40 : Brain development in ADHD. Curr Opin Neurobiol 2015; 30:106–111Crossref, Medline, Google Scholar
41 : Gray matter volume abnormalities in ADHD: voxel-based meta-analysis exploring the effects of age and stimulant medication. Am J Psychiatry 2011; 168:1154–1163Crossref, Medline, Google Scholar
42 : Meta-analysis of structural MRI studies in children and adults with attention deficit hyperactivity disorder indicates treatment effects. Acta Psychiatr Scand 2012; 125:114–126Crossref, Medline, Google Scholar
43 : Developmental trajectories of brain volume abnormalities in children and adolescents with attention-deficit/hyperactivity disorder. JAMA 2002; 288:1740–1748Crossref, Medline, Google Scholar
44 . Mapping the development of the basal ganglia in children with attention-deficit/hyperactivity disorder. J Am Acad Child Adolesc Psychiatry. 2014;53(7):780-789.e711.Crossref, Medline, Google Scholar
45 : Brain alterations in adult ADHD: effects of gender, treatment and comorbid depression. Eur Neuropsychopharmacol 2014; 24:397–409Crossref, Medline, Google Scholar
46 : Developmentally stable whole-brain volume reductions and developmentally sensitive caudate and putamen volume alterations in those with attention-deficit/hyperactivity disorder and their unaffected siblings. JAMA Psychiatry 2015; 72:490–499Crossref, Medline, Google Scholar
47 : Neuroanatomical abnormalities and cognitive impairments are shared by adults with attention-deficit/hyperactivity disorder and their unaffected first-degree relatives. Biol Psychiatry 2014; 76:639–647Crossref, Medline, Google Scholar
48 : Multimodal magnetic resonance imaging study of treatment-naïve adults with attention-deficit/hyperactivity disorder. PLoS ONE 2014; 9:e110199Crossref, Medline, Google Scholar
49 : Toward Defining the Neural Substrates of ADHD: A Controlled Structural MRI Study in Medication-Naive Adults. J Atten Disord (Epub ahead of print, Nov 4, 2013)Google Scholar
50 : Diffusion tensor imaging in attention deficit/hyperactivity disorder: a systematic review and meta-analysis. Neurosci Biobehav Rev 2012; 36:1093–1106Crossref, Medline, Google Scholar
51 : White matter abnormalities and their impact on attentional performance in adult attention-deficit/hyperactivity disorder. Eur Arch Psychiatry Clin Neurosci 2012; 262:351–360Crossref, Medline, Google Scholar
52 : White matter alterations at 33-year follow-up in adults with childhood attention-deficit/hyperactivity disorder. Biol Psychiatry 2013; 74:591–598Crossref, Medline, Google Scholar
53 : White matter microstructure and the variable adult outcome of childhood attention deficit hyperactivity disorder. Neuropsychopharmacology 2015; 40:746–754Crossref, Medline, Google Scholar
54 : Disturbed structural connectivity is related to inattention and impulsivity in adult attention deficit hyperactivity disorder. Eur J Neurosci 2010; 31:912–919Crossref, Medline, Google Scholar
55 : White matter microstructure and developmental improvement of hyperactive/impulsive symptoms in Attention-Deficit/Hyperactivity Disorder. J Child Psychol Psychiatry (Epub ahead of print, Jan 10, 2015)Medline, Google Scholar
56 : Deviant white matter structure in adults with attention-deficit/hyperactivity disorder points to aberrant myelination and affects neuropsychological performance. Prog Neuropsychopharmacol Biol Psychiatry 2015; 63:14–22Crossref, Medline, Google Scholar
57 . Different mechanisms of white matter abnormalities in attention-deficit/hyperactivity disorder: a diffusion tensor imaging study. J Am Acad Child Adolesc Psychiatry. 2014;53(7):790-799.e793.Crossref, Medline, Google Scholar
58 : Toward systems neuroscience of ADHD: a meta-analysis of 55 fMRI studies. Am J Psychiatry 2012; 169:1038–1055Crossref, Medline, Google Scholar
59 : Meta-analysis of functional magnetic resonance imaging studies of inhibition and attention in attention-deficit/hyperactivity disorder: exploring task-specific, stimulant medication, and age effects. JAMA Psychiatry 2013; 70:185–198Crossref, Medline, Google Scholar
60 : Underarousal in Adult ADHD: How Are Peripheral and Cortical Arousal Related? Clin EEG Neurosci (Epub ahead of print, March 23, 2015)Google Scholar
61 : A neurophysiological marker of impaired preparation in an 11-year follow-up study of attention-deficit/hyperactivity disorder (ADHD). J Child Psychol Psychiatry 2013; 54:260–270Crossref, Medline, Google Scholar
62 Cross-Villasana F, Finke K, Hennig-Fast K, et al. The Speed of Visual Attention and Motor-Response Decisions in Adult Attention-Deficit/Hyperactivity Disorder. Biol Psychiatry. 2015.Google Scholar
63 : Sensory gating in adult with attention-deficit/hyperactivity disorder: Event-evoked potential and perceptual experience reports comparisons with schizophrenia. Biol Psychol 2015; 107:16–23Crossref, Medline, Google Scholar
64 : Functional connectivity of default mode network components: correlation, anticorrelation, and causality. Hum Brain Mapp 2009; 30:625–637Crossref, Medline, Google Scholar
65 : Default mode network activity and connectivity in psychopathology. Annu Rev Clin Psychol 2012; 8:49–76Crossref, Medline, Google Scholar
66 : Disrupted network architecture of the resting brain in attention-deficit/hyperactivity disorder. Hum Brain Mapp 2014; 35:4693–4705Crossref, Medline, Google Scholar
67 : Lag in maturation of the brain’s intrinsic functional architecture in attention-deficit/hyperactivity disorder. Proc Natl Acad Sci USA 2014; 111:14259–14264Crossref, Medline, Google Scholar
68 : Sensation-to-cognition cortical streams in attention-deficit/hyperactivity disorder. Hum Brain Mapp 2015; 36:2544–2557Crossref, Medline, Google Scholar
69 : Attention network hypoconnectivity with default and affective network hyperconnectivity in adults diagnosed with attention-deficit/hyperactivity disorder in childhood. JAMA Psychiatry 2013; 70:1329–1337Crossref, Medline, Google Scholar
70 : Child psychiatry branch of the National Institute of Mental Health longitudinal structural magnetic resonance imaging study of human brain development. Neuropsychopharmacology 2015; 40:43–49Crossref, Medline, Google Scholar