Consensus Clinical Guidance for Diagnosis and Management of Adult COVID-19 Encephalopathy Patients
Abstract
Encephalopathy, a common condition among patients hospitalized with COVID-19, can be a challenge to manage and negatively affect prognosis. While encephalopathy may present clinically as delirium, subsyndromal delirium, or coma and may be a result of systemic causes such as hypoxia, COVID-19 has also been associated with more prolonged encephalopathy due to less common but nevertheless severe complications, such as inflammation of the brain parenchyma (with or without cerebrovascular involvement), demyelination, or seizures, which may be disproportionate to COVID-19 severity and require specific management. Given the large number of patients hospitalized with severe acute respiratory syndrome coronavirus–2 infection, even these relatively unlikely complications are increasingly recognized and are particularly important because they require specific management. Therefore, the aim of this review is to provide pragmatic guidance on the management of COVID-19 encephalopathy through consensus agreement of the Global COVID-19 Neuro Research Coalition. A systematic literature search of MEDLINE, medRxiv, and bioRxiv was conducted between January 1, 2020, and June 21, 2021, with additional review of references cited within the identified bibliographies. A modified Delphi approach was then undertaken to develop recommendations, along with a parallel approach to score the strength of both the recommendations and the supporting evidence. This review presents analysis of contemporaneous evidence for the definition, epidemiology, and pathophysiology of COVID-19 encephalopathy and practical guidance for clinical assessment, investigation, and both acute and long-term management.
While several neurological manifestations of severe acute respiratory syndrome coronavirus–2 (SARS-CoV-2) infection have been reported, the most common among patients hospitalized with COVID-19 is encephalopathy, which may be present in 2.3%–28.0% of cases in large observational studies or even more commonly in some settings, such as intensive care (1–11).
The identification of encephalopathy in association with COVID-19 is of considerable importance, particularly because it confers a negative impact on both mortality and morbidity that can be independent of COVID-19 disease severity (3, 6, 9, 10). Moreover, while the longer-term sequelae of encephalopathy associated with COVID-19 are yet to be determined, it is likely that individuals affected will require increased medical support and neurorehabilitation, resulting in substantial individual and public health care burden (10, 12, 13).
Description
Encephalopathy is a broad term that encompasses a wide range of presentations and etiologies. The term is often used in a heterogeneous manner, and conformity to strict definitions and confirmation of the pathophysiology can be lacking (14). Early in the pandemic, several studies highlighted the necessity for clear and consistent definitions to categorize neurological complications associated with COVID-19 and also suggested specific clinical case definitions (1, 12, 15).
In early 2020, prior to our more complete appreciation of the pandemic, 10 societies—largely representing North American and Northern European clinicians in the fields of neurology, neurointensive care, and delirium—published a position statement on encephalopathy and delirium terminology (16). This statement defined acute encephalopathy as “a rapidly developing pathobiological process in the brain,” which usually evolves over less than 4 weeks, and usually within hours to a few days (16). They proposed a nomenclature by which the clinical presentation (i.e., symptoms and signs) of encephalopathy could be labeled as “delirium” and “subsyndromal delirium,” or “coma,” on the basis of certain diagnostic criteria and level of alertness, respectively (16). In addition to semantic precision in the use of labels to describe clinical manifestations of encephalopathy, sufficient clinical evaluation and investigation are required to make clinically relevant diagnostic and treatment decisions.
Consequently, a diagnosis of COVID-19 encephalopathy must comprise the following four components: 1) inclusion of a robust diagnosis of COVID-19 (ideally through confirmation of SARS-CoV-2 by a validated method or in reference to the World Health Organization [WHO] COVID-19 criteria for “probable” or “possible” disease [17]); 2) documentation of a plausible temporal relationship between infection and symptom onset, such as that proposed by the Brighton Collaboration (18); 3) exclusion of alternative etiologies unrelated to SARS-CoV-2, such as primary organ dysfunction, intoxication, or primary autoimmune disease; and 4) through robust clinical examination and investigation, differentiation between brain dysfunction that is proportionate and secondary to systemic COVID-19 severity and a primary brain disease that may have a variable degree of systemic COVID-19 features (1, 6, 7, 9, 12–16).
The Epidemiological Picture of COVID-19 Encephalopathy
While anosmia, ageusia, and headache are common symptoms of COVID-19, encephalopathy is the most common neurological complication among hospitalized COVID-19 patients (19). In a study of more than 20,000 patients hospitalized with COVID-19 in the United Kingdom, nearly 25.0% had confusion (20). In a U.S. electronic health record analysis of 12,601 patients hospitalized with COVID-19, 8.7% developed acute encephalopathy (9). This estimate was similar (12.0%) among 4,491 patients hospitalized with COVID-19 at four New York City hospitals; of these, the majority developed encephalopathy immediately prior to admission (14). Moreover, acute encephalopathy was the most prevalent neurological complication, present in 49.0% of more than 3,500 consecutive hospitalized COVID-19 patients and in 17.0% and 25.0% of those who received a formal neurological consultation in the CoroNerve surveillance survey and GCS-COVID/ENERGY cohorts, respectively (21, 22). The prevalence of encephalopathy is higher among older patients and those requiring intensive support, with two critical care-based studies reporting encephalopathy in greater than 80.0% of patients (22, 23). Encephalopathy may also be more common among patients with acute respiratory distress syndrome (ARDS) secondary to SARS-CoV-2; for example, this was present in 54.9% of 2,088 such patients referred to critical care in one multicenter cohort study spanning 14 countries (24). However, encephalopathy was also reported during emergency department visits among 28.0% of 817 adults aged >65 years, making it the sixth most common presenting feature in this age group (25). Overall, these broad patterns of prevalence reflect the largest risk factors associated with COVID-19 encephalopathy: advancing age and COVID-19 illness severity (9, 14, 26).
One study estimated that being older than 75 years increases the risk of encephalopathy by 51.0% (relative risk=1.51, 95% CI=1.17–1.95) (25). In another study, the incidence of encephalopathy increased steadily with age, from 33.0% among those younger than 40 years to 74.0% among those older than 80 years (3). Patients with COVID-19 encephalopathy are also more likely to be male (9, 14, 24) and have comorbidities, for example, hypertension, obesity, diabetes mellitus, coronary artery disease, and chronic kidney or liver disease (9, 14). The risk of encephalopathy is also increased among patients with preexisting neurological conditions, including Parkinson’s disease and other movement disorders (9, 14, 25), history of ischemic stroke (9, 14, 25), and dementia (14, 25, 27), as well as history of a mental health disorder (14, 25).
However, encephalopathy disproportionate to age, premorbid health, and systemic severity of COVID-19 has also been reported and may be due to cerebrovascular events, demyelinating disease, and other inflammatory pathophysiology (2, 8, 10–13, 15). For example, in the CoroNerve U.K.-wide surveillance study, while 25 hospitalized adults (9.0%) met the criteria for delirium, an additional 13 (5.0%) had severe encephalopathy that was beyond the criteria for delirium due to significantly impaired conscious levels and/or new-onset seizures (2).
Given the high incidence of encephalopathy among patients with COVID-19, its association with poor outcome, and the complexities of diagnosis, investigation, and symptom management, we conducted a systematic collation of the literature to inform the development of pragmatic clinical management guidance for utility in high-, medium-, and low-income settings through the Global COVID-19 Neuro Research Coalition (28). We set out to provide practical guidance to answer the following clinical questions:
What is the pathophysiology of COVID-19 encephalopathy?
Which clinical features suggest COVID-19 encephalopathy?
Which clinical features suggest a primary central nervous system (CNS) pathology?
What investigations should be performed to establish these CNS diagnoses?
How should patients with COVID-19 encephalopathy be managed?
What is the prognosis for patients with COVID-19 encephalopathy?
What is the role of rehabilitation for these patients?
Search Strategy and Methods
A Medline search was conducted between January 1, 2020, and June 21, 2021, using the following search terms: (COVID-19 OR SARS-CoV2 OR SARS-CoV-2) AND (neurol* OR neuropath* OR nervous system OR brain OR encephal* OR meningit* OR stroke OR Guillain-Barré syndrome OR cerebr* OR psych* OR mania OR psycho* OR functional OR catatonia OR cognit* OR depress* OR anxi* OR obsessive OR posttraum* OR postraum* OR PTSD OR behavior OR epilep* OR seizure OR headache* OR migraine OR crani* OR cloza* OR deliri*). A medRxiv and bioRxiv search was conducted during the same time frame for the following search terms: COVID-19 OR SARS-CoV2 OR SARS-CoV-2 in “neurology” and/or “psychiatry” categories. We conducted a hand review of references cited in the identified bibliographies and additional publications, and preprints identified by the expert panel in the consortium were accessed.
A modified Delphi approach was undertaken by the Global COVID-19 Neuro Research Coalition to determine conclusions, along with a parallel approach to score the consensus strength of both the recommendations and the supporting evidence, as used in previous guidelines (29, 30).
The Global COVID-19 Neuro-Research Coalition is a consortium of clinical academic neurologists and neuropsychiatrists—and includes patient and public involvement—in both high-income and low- and middle-income settings spanning the spectrum of subspeciality interests (28). The central secretariat of the coalition are also members of the WHO Brain Health Unit Expert Forum on Neurological and Neuropsychiatric Complications of COVID-19. The coalition undertook a virtual scoping exercise to identify key clinical questions regarding the pathophysiology, diagnosis, and management of encephalopathy among COVID-19 patients. These questions were initially assigned to a partnership between one clinician in a high-income setting and one in a low-/middle-income setting and organized in subcategories reflecting clinical and academic experience. After a systematic literature review utilizing the neurology and neuropsychiatry of COVID-19 database (https://neuropsychcovid.net), answers to these questions were drafted by the coalition subgroups. The answers were then circulated to the main co-authorship group for review and comment regarding the grading of recommendations. After iterative review within the primary authorship group, the manuscript was circulated to the wider stakeholder authorship for critical review of the nature and strength of the recommendations. At each stage of iterative review, discrepancies in opinion were resolved through discussion, and the coalition consensus conclusion was included in the submitted manuscript.
We acknowledge that in this fast-moving field, recommendations and their underlying supporting evidence are likely to change rapidly for SARS-CoV-2-specific complications; indeed, there are some general points applicable to encephalopathy caused by other systemic perturbations with other infections. However, in part due to the rapid and large-scale clinical trials for COVID-19 treatment, we are able to provide more supporting evidence than for encephalopathy generically. We consider those components that are more specific to COVID-19 to be the parainfectious and postinfectious direct CNS complications of SARS-CoV-2 infection, such as cerebrovascular complications, acute disseminated encephalomyelitis (ADEM), and acute necrotizing encephalitis (ANE), among others. While demyelinating conditions have been reported with other infections that are not as widespread, the sheer scale of the denominator of people infected with SARS-CoV-2 has resulted in these relatively unlikely complications being common and have allowed for clinico-epidemiologic studies not previously possible to guide clinical management.
What is the Pathophysiology of COVID-19 Encephalopathy?
The consortium elected not to provide recommendations for this section because, following consideration, there were no clinically actionable conclusions. Nevertheless, the pertinent literature to guide clinicians is summarized here (Figure 1).
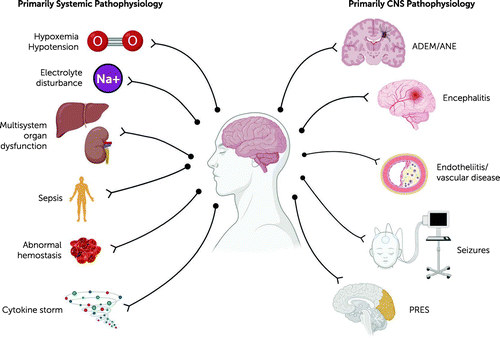
FIGURE 1. Potential pathophysiological mechanisms causing encephalopathy in patients infected with SARS-CoV-2a
aADEM=acute disseminated encephalomyelitis; ANE=acute necrotizing encephalopathy; Na+=natrium; PRES=posterior reversible encephalopathy syndrome. (Figure produced in BioRender, copyright 2021.)
Primary Systemic Pathophysiology
The pathophysiology of COVID-19 encephalopathy is often multifactorial in approximately four out of five hospitalized patients (14).
Hypoxemia and hypotension.
Low oxygen saturations (typically <88.0%) and hypotension are significantly related to encephalopathy in COVID-19 (14). Prolonged hypoxia accompanied by glutamate excitotoxicity results in oligodendrocyte cell injury, blood-brain barrier disruption, and microhemorrhages (31–33). Neuropathological evidence of acute hypoxic injury among COVID-19 patients whose clinical course was complicated by encephalopathy has been reported in multiple autopsy cohorts and was present in 28.0% of case subjects in the largest systematic review to date (34–40). Multiple MRI studies have also identified evidence of hypoxic-ischemic brain injury, including watershed injury, cortical and deep gray matter restricted diffusion, leukoencephalopathy, and microhemorrhages (31, 41–48). Additionally, several studies have described SARS-CoV-2 delayed posthypoxic leukoencephalopathy, characterized by a lucid interval following hypoxia, and delayed progressive encephalopathy leading to coma, with necrosis of oligodendrocytes and demyelination (47–50). It is possible that several reported MRI-detected cases of “COVID leukoencephalopathy,” when present with microhemorrhages, were actually due to hypoxic brain injury and iatrogenic complications from the treatment of hypoxia, in addition to metabolic disturbances, or these factors may have indeed combined with a viral endotheliopathy (51).
Electrolyte disturbance.
Hyponatremia has been reported in 10.0%–50.0% of COVID-19 patients and may be related to interleukin (IL)–6-mediated vasopressin release (52–55). Additionally, hypernatremia, hypokalemia, hypocalcemia, and acid-base disturbances have been reported and may contribute to metabolic encephalopathy (14, 54–57). Nevertheless, hyponatremia may also be seen in primarily CNS disorders, reflecting the syndrome of inappropriate antidiuretic hormone release or cerebral salt-wasting syndrome.
Multisystem organ dysfunction.
In severe COVID-19, multisystem organ dysfunction, including renal failure, is frequently the cause of encephalopathy. Overall, acute kidney injury has been reported in 8.0%–81.0% of patients with COVID-19, particularly among those requiring intensive care (14, 35, 47, 58), potentially due to cytokine storms, thrombotic events, and/or direct renal cellular viral injury (59). Secondary metabolic effects, including acidosis and electrolyte disturbance, can further compound uremic encephalopathy.
Sepsis.
Septic encephalopathy occurs in up to 70.0% of patients with bacteremia/viremia, mediated by inflammatory cytokines, blood-brain barrier dysfunction, microthrombosis, and alteration of neurotransmitters (60–62). SARS-CoV-2 sepsis is likely, at least in part, mediated by a cytokine storm, including IL-1, IL-6, and tumor necrosis factor alpha (TNFα) (63, 64).
Abnormal hemostasis.
In COVID-19, inflammatory mediators can trigger hypercoagulability, resulting in increased D-dimer, thrombocytopenia, and prolonged prothrombin time (65). This predisposes to microthrombosis and thromboembolic events in multiple organs, including the brain, which has been a common neuropathological finding in COVID-19 encephalopathy (66).
Cytokine storm.
Cytokine storm is the hallmark of ARDS and multiorgan dysfunction in severe COVID-19 and has been associated with interleukins (e.g., IL-6 and IL-1β), interferons, and TNFα; in particular, IL-6 correlates with COVID-19 severity, perhaps through blood-brain barrier dysfunction (63, 65, 67–69). Endothelial inflammation, microhemorrhages, and extravasation of fibrinogen, reflecting breakdown of the blood-brain barrier, have been reported in neuropathological studies (14, 70–72). Moreover, C-reactive protein (CRP), procalcitonin, d-dimer, and ferritin correlate with CNS damage and poor prognosis (64, 65). In addition, reduced natural killer cell counts and elevated soluble IL-2 receptor alpha chain have also been reported, although rarely (73, 74).
Primary CNS Pathophysiology
Endotheliitis/vascular disease.
Brain MRI of 31 encephalopathic patients without a systemic cause identified vessel wall enhancement, particularly of the vertebral arteries, and cerebrospinal fluid (CSF) and postmortem studies have further demonstrated evidence of blood-brain barrier disruption, which could contribute to encephalopathy (14, 70–72, 75). Cerebrovascular complications, including ischemic and hemorrhagic stroke, as well as cerebral venous sinus thrombosis, are widely recognized (1, 2). In addition, while cerebrovascular complications do not necessarily reflect systemic COVID-19 severity in all cases, they may still be associated with conventional cerebrovascular risk factors (2).
Acute disseminated encephalomyelitis/acute necrotizing encephalopathy.
Cases with MRI evidence of both ADEM and ANE have been reported and are thought to reflect a cytokine storm and/or adaptive host responses, and, to our knowledge, CSF evidence of SARS-CoV-2 has only been reported in one case (43, 57). Autopsy studies have confirmed ADEM-like pathology and sometimes hemorrhagic white matter lesions with axonal injury (35).
Encephalitis.
While evidence for direct viral encephalitis is lacking, parainfectious and postinfectious encephalitis have been reported (1, 2). Some of these cases may represent autoimmune encephalitis triggered by infection, although specific autoantibodies, including N-methyl-d-aspartate receptor (NMDA-R) and contactin-associated protein-like 2, as well as anti-Purkinje cell, striatal, and hippocampal antibodies, have only been identified in a small number of patients (76). Angiotensin-converting enzyme 2-receptor (ACE2-R) expression, which is essential for viral cell entry, has been reported in olfactory epithelial cells. However, single-cell sequencing for ACE2-R expression (which eliminates contamination) did not detect expression in olfactory neurons (77). While some investigators have reported SARS-CoV-2 by reverse transcription polymerase chain reaction (PCR) in neural tissue, most have reported low ribonucleic acid copies or not reported levels at all, and therefore the positive result may simply reflect blood contamin ation from within cerebral vessels or the result of a leaky blood-brain barrier; in such cases, SARS-CoV-2 may enter the CNS as an innocent bystander, rather than through neurotropism (34, 35, 78–80). Moreover, in two separate studies (79, 81) of in situ hybridization, SARS-CoV-2 was not detected in any neural tissue.
Seizures.
There are reports of de novo seizures, including some patients with nonconvulsive status epilepticus with resultant prolonged altered mental status, although this may be mechanistically heterogenous (61). In some cases, this may represent decompensation in older patients with underlying neurological degenerative disease; however, in some younger patients, new-onset seizures and status epilepticus have been identified (2).
Posterior reversible encephalopathy syndrome (PRES).
While PRES is driven by systemic processes, the primary clinical presentation reflects cerebral dysfunction. MRI studies have confirmed classical posterior appearances of PRES in the context of severe hypertension and presenting with symptoms of encephalopathy and often with seizures and visual cortex impairment (60).
Which Clinical Features Suggest COVID-19 Encephalopathy?
Recommendations
Encephalopathy manifests clinically as delirium, subsyndromal delirium, and coma. [Strongly recommended; evidence from nonrandomized studies.]
Premorbid factors associated with risk of developing COVID-19 encephalopathy due to systemic processes, including age and prior neurological diagnoses, especially dementia. [Recommended; evidence from nonrandomized studies.]
In this group, risk increases with duration of hospital stay and may be most common at approximately 2 weeks into respiratory symptom onset. [Recommended, but other alternatives may be acceptable; evidence from nonrandomized studies.]
However, patients may also present with symptoms of encephalopathy predating respiratory symptoms. [Recommended; evidence from nonrandomized studies.]
Hypoxia and/or hypercapnia is strongly associated with risk of COVID-19 encephalopathy. [Recommended; evidence from nonrandomized studies.]
Clinical Approach
Clinically, encephalopathy presents with delirium, subsyndromal delirium, and coma (16). In DSM-5, delirium is defined as a transient disturbance of consciousness presenting with change in cognition and predominantly decreased attention (82). The presentation is usually waxing and waning, with fluctuating levels of consciousness. The patient is generally disoriented and may have illusions, hallucinations, or delusions, along with autonomic nervous system symptoms, such as tachycardia, diaphoresis, and mydriasis (83, 84).
Taking a history may require the presence of an informant that is either a family member or caregiver (e.g., nursing home or hospital staff). A high index of suspicion is required because a change in orientation or cognitive function may be either gradual or sudden. Some patients may be aggressive or agitated and may be at risk for harm to themselves or others, termed “hyperactive delirium.” However, the patient may also be considered to have depression due to inactivity or withdrawal from daily tasks, termed “hypoactive delirium” (82). Symptoms specific to the etiology of encephalopathy may also be present. These symptoms may include fever, headache, polyuria, polydipsia, and seizures. Standardized tools for assessment, such as the confusion assessment method and the 4 A’s test, can also be used to screen patients for delirium and therefore provide evidence of acute encephalopathy (85, 86). We recommend that clinicians be alert to the potential for COVID-19 to cause encephalopathy or delirium and have a low threshold for using assessment tools to screen patients, especially those patients at high risk, such as those in intensive care or high-dependency units and potentially those requiring oxygen on a general ward. However, we acknowledge that outside of an observational study, it is a challenge to ask that potentially overstretched clinicians screen all patients.
Highest at risk for encephalopathy are COVID-19 patients of older age with underlying neurological disease or injury, such as dementia and stroke, as well as other systemic diseases (75, 87–89). Elderly patients are particularly vulnerable because their risk increases with longer hospital stays, with a median of 14 days from respiratory symptom onset in one national surveillance study (89, 90). However, while neurological abnormalities commonly follow respiratory symptoms of COVID-19, they may also precede them (75, 91).
Which Clinical Features Suggest a Primary Central Nervous System (CNS) Pathology?
Recommendations
Clinical features suggestive of a primary CNS disease include new-onset headache (especially if “thunderclap,” worse lying flat, or otherwise unusual for the patient), focal neurological deficits, symptoms and signs of raised intracranial pressure, seizures, movement disorders, and/or a Glasgow Coma Scale (GCS) score <13/15. [Recommended; evidence from nonrandomized studies.]
Encephalopathy in COVID-19 patients without clear symptoms, signs, or systemic parameters highly suggestive of a peripheral perturbation causing a secondary encephalopathy, such as hypoxia, hypercapnia, hyponatremia/hypernatremia, hypoglycemia/hyperglycemia, shock, or iatrogenic (e.g., shortly after ventilation or extubation), should be investigated for primary CNS complications of SARS-CoV-2 infection, especially if CNS symptoms and signs are identified (Table 1; Figure 2), as these would be less common in delirium due to systemic perturbations. [Recommended; evidence from nonrandomized studies.]
Pathology | Systemic cause | Investigation |
---|---|---|
Organ dysfunction | Hypercapnia/hypoxia, hepatic failure, acute kidney injury, thyroid disorders, and cardiac failure | Pulse oximetry and blood gas; ALT, AST, GGT, and ALP; ammonia; creatinine and urea; TSH and T4; ECG, echocardiography, and clinical examination |
Metabolic dysfunction | Hypernatremia/hyponatremia, hypercalcemia/hypocalcemia, hypomagnesemia, hyperglycemia/hypoglycemia, hypothermia, fever/hyperpyrexia, and cytokine storm | Plasma Na+, corrected plasma Ca2+, and plasma Mg2+; plasma/finger prick glucose; temperature/observations; IL-1, IL-6, and TNFα (guided by local expertise) |
Toxicity | Sedatives, corticosteroids, hydroxychloroquine, lopinavir, ritonavir, tocilizumab, and drugs and alcohol | Patient history, treatment review, blood alcohol level, and urinary drug screen |
Sepsis | Superinfection (typically bacterial or fungal) | Blood, urine, and sputum cultures; serology/PCR |
Vascular disease | Hypertensive encephalopathy and severe hypotension | Noninvasive or invasive blood-pressure monitoring |
Nutritional deficiency | Wernicke encephalopathy | B12/thiamine |
TABLE 1. Common systemic causes of encephalopathy in patients with SARS-CoV-2 infection and associated investigationa
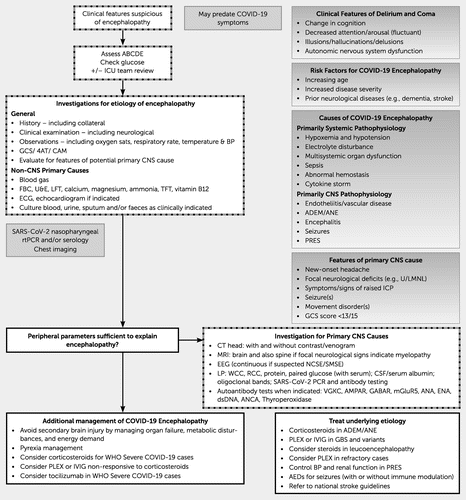
FIGURE 2. Algorithm for the diagnosis and management for adult patients with encephalopathy caused by SARS-CoV-2 infectiona
aABCDE=Airway Breathing Circulation Disability Exposure; ADEM=acute-demyelinating encephalomyelitis; AMPAR=alpha-amino-3-hydroxy-5-methyl-4-isoxazolepropionic acid receptor; ANA=antinuclear antibodies; ANCA=antineutrophil cytoplasmic antibodies; ANE=acute necrotizing encephalitis; BP=blood pressure; CAM=confusion assessment method; CNS=central nervous system; CSF=cerebrospinal fluid; CT=computerized tomography; CVST=cerebral venous sinus thrombosis; dsDNA=double stranded DNA; ECG=electrocardiogram; EEG=electroencephalogram; ENA=extractable nuclear antigen; FBC=full blood count; 4AT=4 A’s test; GABA-R=gamma-aminobutyric acid receptor; GBS=Guillain-Barré Syndrome; GCS=Glasgow Coma Scale; ICP=intracranial pressure; ICU=intensive care unit; IVIG=intravenous immunoglobulin; LFT=liver function test; LP=lumbar puncture; mGluR5=metabotropic glutamate receptor 5; NCSE=nonconvulsive status epilepticus; PCR=polymerase chain reaction; PLEX=plasma exchange; PRES=posterior reversible encephalopathy syndrome; RBC=red blood cells; RCC=red cell count; rtPCR=reverse transcription PCR; SMSE=simple motor status epilepticus; TFT=thyroid function test; U/LMNL=upper/lower motor neuron lesion; U&E=urea and electrolytes; VGKC=voltage-gated potassium channel; WBC=white blood cells; WCC=white cell count; WHO=World Health Organization.
Clinical Approach
It is critical that a diagnosis of encephalopathy is considered for all patients with COVID-19, and basic neurological assessment should be part of the evaluation. At a minimum, this should include recording of the GCS or Full Outline of Unresponsiveness scale score at hospital admission, and more complete neurological assessment should be conducted, as well as serial monitoring using these assessment tools, if the patient’s neurological function is compromised or if intensive care unit support is required (92, 93).
Clinical evidence from the history and/or examination that may point toward a primary CNS disease as a result of SARS-CoV-2 infection, such as cerebrovascular pathology, demyelination, and other CNS inflammatory complications, includes new-onset headache (especially if “thunderclap” in character, worse lying flat, or otherwise unusual for the patient), focal neurological features (such as hemiparesis, hemisensory disturbance, diplopia, or cortical blindness), symptoms and signs of raised intracranial pressure (such as transient visual obscuration or papilledema), meningism, seizures (including subtle motor and nonconvulsive status epilepticus), movement disorders (such as opsoclonus-myoclonus syndrome), and/or a GCS score <13 (Table 1) (1, 2, 75, 89, 94–96).
What Investigations Should be Performed to Establish These CNS Diagnoses?
Recommendations
Patients with symptoms and signs of encephalopathy should be evaluated for systemic causes, including hypoxia/hypercapnia, metabolic disturbance, organ dysfunction, coagulation disturbance, and iatrogenic contributing factors. [Strongly recommended; evidence from nonrandomized studies.]
Patients without a clear systemic cause and/or with symptoms and/or signs suggestive of direct CNS pathology should be investigated with neuroimaging. [Recommended; evidence from nonrandomized studies.]
The optimal neuroimaging modality for CNS inflammatory disorders, leukoencephalopathy, PRES, and demyelinating disorders, such as ADEM, is brain MRI, including contrast-enhanced MR angiography in those with suspected vasculitis. [Strongly recommended; evidence from nonrandomized studies.]
MRI should include imaging of the spine if myelopathic features are present, such as symmetrical upper motor neuron signs and/or bowel/bladder involvement. [Recommended; evidence from nonrandomized studies.]
In patients with acute focal neurological deficits, symptoms and signs of raised intracranial pressure, a thunderclap headache, and/or a rapid decline in conscious level, an urgent computerized tomography (CT) brain scan should be performed, including CT venography in those with suspected cerebral venous sinus thrombosis. [Recommended; evidence from nonrandomized studies.]
CSF studies, including SARS-CoV-2 PCR and antibody testing (matched to serum and CSF:serum albumin ratio) should be performed in patients for whom MRI is nondiagnostic, including investigation for other CNS pathogens. [Recommended; evidence from nonrandomized studies.]
Anti-CNS autoantibodies should be assessed in patients with refractory encephalopathy, especially in those with new-onset status epilepticus and/or movement disorders. [Recommended; evidence from nonrandomized studies.]
Electroencephalogram (EEG) should be performed in patients with otherwise unexplained encephalopathy, especially in those with features suggestive of subtle motor or nonconvulsive status epilepticus, such as focal twitching, posturing, and/or fluctuating consciousness, or those with prior clinical seizures. [Recommended; evidence from nonrandomized studies.]
Clinical Approach
Irrespective of whether the underlying pathology is of a cerebral or a more systemic nature, the potential etiologies must be identified as soon as possible and treated appropriately, and there is a particularly important role for neuroimaging and CSF analysis in patients without an obvious systemic cause (30).
The differential diagnosis of encephalopathy is extensive, and in areas of high incidence of SARS-CoV-2 infection, even relatively low-frequency complications may occur in significant numbers of patients. Therefore, in the absence of obvious systemic explanations for encephalopathy, the workup for patients must be extended for less frequent causes (30). Nevertheless, when it comes to more sophisticated investigations, it is important to consider which patients would benefit from specific investigations, especially where health care resources are limited (Table 2; Figure 2) (76, 96–105).
Imaging and test | Indication | Investigation |
---|---|---|
CT | Altered level of consciousness; headache with “red flags”b; altered behavior; focal neurological signs/symptoms; unexplained generalized seizures; focal/generalized seizures; suspected stroke; and suspected CVST | CT with and without contrast enhancement; CT angiography±CT perfusion in case of sudden-onset symptoms; and CT venography |
MRI (97) | Same as CT in cases of unclear cause | T1; T2; DWI; FLAIR; arterial time of flight; gradient echo/SWI; T1+gadolinium; and T2+gadolinium |
EEG (96, 98, 99) | Suspected nonconvulsive status epilepticus; suspected subtle motor status epilepticus (when unclear of a psychiatric cause or encephalopathy) | EEG; possible improvement of localization of seizures with video EEG; prolonged monitoring considered |
LP (76, 100–104) | In case of suspected encephalitis (including ADEM and ANE), as soon as possible; in the absence of another clear explanation for encephalopathy; unexplained seizures or status epilepticus (once stable); and suspected autoimmune encephalitis or Bickerstaff encephalopathy | WBC; RBC; protein concentration; paired serum/CSF glucose; paired CSF albumin/plasma albumin; paired oligoclonal bands; and paired SARS-CoV-2 PCR and antibody |
Autoantibody tests (105) | Unexplained encephalopathy, particularly if associated with psychiatric features, encephalomyelitis, memory decline, seizures, cerebellar syndrome, movement disorder, or sensory neuronopathy, which may suggest autoimmune encephalitis | Autoantibodies against the following receptors: NMDA-R, LGI-1, CASPR2, AMPAR, GABA-A/B, mGluR5, and DPPX; CRP; ANA; ENA; dsDNA; ANCA; thyroperoxidase autoantibodies; and antiganglioside antibodies |
TABLE 2. Main indications for evaluation of primary central nervous system (CNS) causes of encephalopathy in the context of SARS-CoV-2 and recommended investigationsa
Neuroimaging.
In the appropriate clinical context, CT scanning may identify or confirm evidence of an acute cerebrovascular event and/or evidence of brain shift reflecting cerebral edema. CT venography is indicated for those in whom a cerebral venous sinus thrombosis is suspected. However, MRI is optimal to identify the recognized neuroimaging substrates of CNS complications of SARS-CoV-2 infection, including encephalitis, leukoencephalopathy, and stroke, as well as demyelinating syndromes such as ADEM, while MR angiography is optimal for identifying CNS vasculitis (Figure 3) (2).
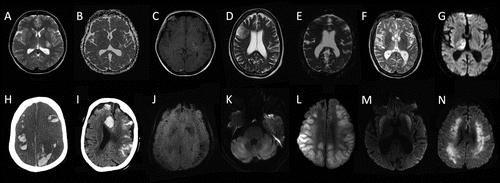
FIGURE 3. Examples of MRI and computerized tomography (CT) imaging identifying central nervous system complications associated with SARS-CoV-2 infection presenting with encephalopathya
aPanel A shows an axial T2-weighted image of patient 1 on day 2 of symptoms, with acute disseminated encephalomyelitis associated with SARS-CoV-2. The image illustrates an ill-defined hyperintensity in the posterior limb of the internal capsule and thalamus. Panel B shows a facilitated diffusion in the apparent diffusion coefficient map in the core of this patient’s lesion, while the periphery is isointense with the brain; and panel C shows the postgadolinium T1-weighted imaging of this patient’s lesion on day 5 from symptom onset, demonstrating incomplete ring enhancement in the medial and posterior aspect consistent with acute demyelination. Panel D shows the axial T2-weighted MRI for patient 2, demonstrating a large area of hyperintensity in the right frontal lobe, and panel E shows evidence of diffusion restriction consistent with an acute infarction in this patient. Panel F shows the MRI head with contrast in patient 3, with encephalopathy and focal neurology in the context of SARS-CoV-2 infection and suspected acute encephalitis, demonstrating high intensity within the right thalamus on axial T2-weighted images; and evidence of restricted diffusion in this patient is shown in panel G. Panel H shows a head CT of patient 4, with profound anoxic brain injury and superimposed intracranial hemorrhages while the patient was on therapeutic-dosed heparin. Panel I shows a CT head imaging demonstrating multicompartmental hemorrhages in patient 5, who had COVID-19 acute respiratory distress syndrome but was not anticoagulated and was without a history of trauma. Panel J shows MRI susceptibility weighted imaging for patient 6, who had COVID-19 acute respiratory distress syndrome, with multiple microhemorrhages at the gray-white matter junction. Panel K shows MRI with diffusion-weighted imaging for patient 7, demonstrating restricted diffusion consistent with hypoxic ischemic brain injury affecting the Purkinje cells of the cerebellum; also shown for this patient are striatal neurons of basal ganglia (panel L), the cortex (panel M), and late hypoxic changes seen in white matter tracts (panel N).
Examination of CSF for SARS-CoV-2 and antiviral antibodies.
Among 430 patients from 242 studies, of whom 321 had cerebral symptoms and signs and 304 had CSF PCR for SARS-CoV-2, only 17 (6.0%) tested positive (76). Clinical features included combinations of encephalopathy, coma, seizures, headache, cerebellar features, paraesthesia, and visual loss. An additional seven patients tested had intrathecal synthesis of anti-SARS-CoV-2 antibodies. Only four (5.0%) of 77 tested had autoimmune antibodies in the CSF. Overall, the CSF white blood cell count was reported in 409 cases and was 21–100 cells/μL in 28 (7.0%) patients and >100 cells/μL in 8 (2.0%) patients. CSF protein concentration was “increased” in 159 (40.0%), >200 mg/dL in 28 (7.0%), and >1,000 mg/dL in five (1.2%) of 397 patients for whom protein concentration was reported (76).
In another study of CSF analysis from six patients—of whom, four had encephalopathy, one had meningism, and one had fatigue and disorientation—SARS-CoV-2 was detected in the plasma and CSF in three patients (100). None had pleocytosis, the albumin ratio did not support blood-brain barrier impairment, and there was no intrathecal immunoglobulin G (IgG) synthesis; however, there was an increase of neopterin and beta-2 microglobulin. In one intensive care unit study of eight patients with prolonged encephalopathy, all had high levels of anti-SARS-CoV-2 IgG antibodies in serum and CSF, with a 1:10 dilution (101). In three cases, the blood-brain barrier was disrupted, and intrathecal synthesis was identified in one case. PCR was negative for SARS-CoV-2 in all cases, and 14-3-3 proteins were elevated in four.
Anti-CNS autoantibody detection.
Autoantibodies have been reported in some small series, including a series of 11 patients with negative SARS-CoV-2 PCR in CSF, but autoantibodies, including those directed against NMDA-R, cardiolipin, beta-2 glycoprotein, myelin, annexin, and intracellular and neuronal surface proteins, may have contributed to encephalopathy, myoclonus, and/or seizures (102). In large case series of patients with various neurological manifestations, most tested cases were negative for SARS-CoV-2 PCR, but a few showed altered albumin ratios, and a minority demonstrated oligoclonal bands (103, 104).
How Should Patients with COVID-19 Encephalopathy be Managed?
Recommendations
Assessment to detect neurological complications is mandatory, and ongoing monitoring is indicated for patients who show abnormalities, including consideration of EEG monitoring if available. [Recommended; evidence from nonrandomized studies.]
The use of advanced cardiorespiratory monitoring should be calibrated to the severity of systemic illness. [Strongly recommended; evidence from nonrandomized studies.]
An acute priority is avoidance of secondary brain injury by earliest possible initiation of intensive care management aimed at preventing or treating organ dysfunction, metabolic disturbances, and hypoxia and ensuring sufficient energy supply to the CNS. [Recommended; evidence from nonrandomized studies.]
Fever should be managed, in particular, avoiding hyperpyrexia. [Recommended; evidence from nonrandomized studies.]
Seizures and nonconvulsive status epilepticus should be identified promptly and managed with standard antiepileptic drug protocols. [Recommended; evidence from nonrandomized studies.]
Corticosteroids are indicated for those with severe systemic COVID-19, which may correlate with severity of encephalopathy in some patients. [Strongly recommended; evidence from randomized trials.]
Tocilizumab or sarilumab is indicated for those with severe systemic COVID-19. Patients with high CRP or IL-6 levels may show particular benefit, although the role of these medications for COVID-19 encephalopathy is not established. [Recommended; evidence from randomized trials.]
Corticosteroids, intravenous immunoglobulin (IVIG), and/or plasma exchange (PLEX) are indicated as an immunomodulatory intervention where encephalopathy is thought to be primarily CNS immune-mediated based on clinical, CSF, and MRI findings (e.g., ADEM, ANE, and encephalitis) and considered in de novo seizures in patients without prior CNS disease. [Recommended; evidence from nonrandomized studies.]
Corticosteroids, IVIG, and/or PLEX may be considered for patients with encephalopathy not adequately explained by systemic parameters or medications in the absence of clear evidence of a CNS immune-mediated cause based on clinical, CSF and imaging findings or where these investigations are not available. [Recommended other alternatives are acceptable; expert opinion only.]
Blood pressure should be controlled using established protocols in patients with PRES. [Recommended; evidence from nonrandomized trials.]
Clinical Approach
Prevention of encephalopathy is vital, and utilization of multicomponent, nonpharmacological interventions is crucial to avoid the development of COVID-19 encephalopathy when caused by systemic perturbations. Once encephalopathy is present, these interventions can continue alongside comprehensive management analogous to the urgency of treatment for sepsis to detect and reduce further insults to the critically ill brain (106–112).
Monitoring.
Systemic monitoring should be calibrated to the severity of systemic disease, with invasive blood hemodynamic monitoring when needed and available. In addition, specific neurological monitoring should include intermittent, or preferably continuous, EEG monitoring where indicated and available. Seizures are well recognized in COVID-19 and may be nonconvulsive (especially among patients who are sedated for mechanical ventilation) and are an often treatable cause of encephalopathy, including in many low- to middle-income country settings (Figure 4). While experience from other diseases suggests that more advanced measures (intracranial pressure and brain chemistry monitoring) may provide additional information for some patients (113), there is limited experience and no evidence of benefit in COVID-19 encephalopathy.
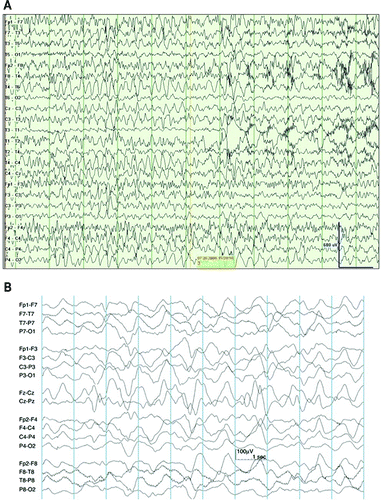
FIGURE 4. Examples of electroencephalogram (EEG) findings in SARS-CoV-2 infection presenting with encephalopathya
aPanel A shows EEG appearances of nonconvulsive status epilepticus; panel B shows EEG appearances of nonspecific encephalopathy, illustrating generalized slowing through both hemispheres.
General supportive care.
Management should target organ dysfunction identified by clinical, laboratory, and imaging evidence, including the correction of hypoxia and hypotension (108–112). Despite recent concerns about liberal oxygen saturation (Sp02) targets in critical illness, there is no evidence that very conservative targets (SpO2 <90.0%) show benefit, and SpO2 targets of 93.0%–96.0% are probably most appropriate (114). For patients with severe ARDS, careful clinical judgment is needed to balance protective lung ventilation against optimal blood gas levels. Blood pressure management should generally target normotension with a lower limit of 60–65 mmHg, but particular attention to tight blood pressure control may be needed for patients with PRES. Other regimens include aggressive treatment of pyrexia and hyperpyrexia (>40°C), aiming at a liberally defined normothermia (<38°C) (115), restoration of metabolic homeostasis (including renal replacement therapy where appropriate), and addressing additional organ dysfunction. While therapeutic hypothermia has been used in some cases, there is not currently sufficient evidence to support its use in routine care (116). Other concerns include controlling for hypoglycemia and severe hyperglycemia, as well as inhibiting rapid changes in sodium levels, which may precipitate osmotic demyelination and has been reported in COVID-19 (117, 118).
Iatrogenic insults.
Encephalopathy may also be due, at least in part, to medication specific to COVID-19 and agents used more generally in critical illness (119). The general critical care literature suggests the need for care with sedatives and drugs with anticholinergic effects (93). While high-dose corticosteroids may be lifesaving in severe COVID-19, they may precipitate or contribute to acute psychotic effects in some individuals. If possible, pre-COVID-19 steroid therapy should probably be continued, but in some instances, this may not be possible.
Systemic Immunomodulation
There is increasing recognition that the host response is a critical mediator of severe COVID-19, and there is now good evidence of benefit from a range of immunomodulatory interventions. While the available evidence is dynamic, interventions that are currently thought to show benefit in WHO-criteria severe COVID-19 include corticosteroids (dexamethasone and hydrocortisone), IL-6 inhibitors (tocilizumab and sarilumab), and Janus kinase (JAK) inhibitors (baricitinib) (120). While evidence of the impact of these interventions on neurological complications is unclear, the modulation of the host immune response may be expected to mitigate the effects of cytokine excess generally and also affect immune-mediated CNS diseases more specifically.
Specific CNS Therapy
In cases with well-characterized CNS syndromes (such as ADEM, ANE, encephalitis, and perhaps de novo seizures), immunomodulation is indicated, regardless of COVID-19 disease severity, including corticosteroids, PLEX, and/or IVIG, although the latter may increase the risk of thrombosis (Figure 2) (121). However, supporting data are largely from case reports and case series, and mechanisms of action remain unclear. In addition to well-defined CNS syndromes, these immunomodulatory approaches may also be useful for some patients, especially when a potential inflammatory CNS process driving encephalopathy is supported by clinical, CSF, and/or MRI features. The use of other immunomodulatory interventions (e.g., IL-1 or IL-6 antagonists) will depend on local experience and availability, as well as whether the drug is indicated on the basis of the systemic severity of COVID-19, regardless of CNS manifestations. Trials are currently under way for interferons and inhibitors of multiple inflammatory mediators, including IL-1, IL-6, TNFa, and JAK inhibitors, as well as neutralizing antibodies, which have been granted emergency use authorization in some countries (122, 123).
What is the Prognosis for Patients with COVID-19 Encephalopathy?
The consortium elected not to provide recommendations for this section because following consideration, there were no clinically actionable conclusions. However, the pertinent literature to guide clinicians is summarized in this review.
Acute COVID-19 encephalopathy is associated with increased mortality and morbidity, with varying estimates of effect size based on different case definitions and patient populations. In older populations, encephalopathy at COVID-19 onset is associated with an up to fourfold increase in mortality and unfavorable outcome (22, 124–128). In one of the largest multicenter cohort studies to date, comprising 3,055 hospitalized COVID-19 patients in the United States, those with acute encephalopathy had a 5.5-fold increased risk of death during hospitalization, even after adjusting for age, sex, race, ethnicity, and study site (3). For patients with coma, the adjusted risk for in-hospital death was 7.7 times higher. A large study conducted in Brazil found that 55.0% of in-hospital deaths occurred among patients with manifestations of encephalopathy, which was 1.75 times higher than that for those without encephalopathy (125). In those who survive beyond hospitalization, acute encephalopathy is associated with up to three-fold higher 30-day mortality and worse physical function at 4 weeks postadmission (4, 9, 126). The impact of acute encephalopathy on long-term neurological, cognitive, and psychiatric outcomes after COVID-19 remains unknown at this time, but early clinical experience suggests that sequelae, such as posttraumatic stress disorder (PTSD), may not be uncommon.
The association between the causal pathophysiology of encephalopathy and poor outcome in acute COVID-19 remains unclear and requires further investigation. Impaired consciousness at hospital admission is a key risk factor for developing critical illness with COVID-19 (129). In cases with encephalopathy reflecting systemic COVID-19 disease severity, common risk factors, such as older age, higher intensive treatment and unit/ventilator requirements, and more preexisting conditions, are more common, and these same factors may also negatively affect mortality and long-term outcome (3, 4, 9, 125). Future longitudinal studies using standardized ascertainment methods; case and control definitions; and comprehensive cognitive, psychiatric, and neurological assessments are under way to further understand the prognosis of COVID-19 patients who experience acute encephalopathy (130).
What is the Role of Rehabilitation for These Patients?
Recommendations
Patients experiencing ongoing issues post-COVID-19 encephalopathy should be referred to rehabilitation services operated by multidisciplinary teams. [Recommended; expert opinion only.]
Given the scale of the pandemic, thought should be given to rehabilitation interventions that are scalable, both nationally and internationally (e.g., simple screening processes, remote assessment and treatment options, home rehabilitation, and public-private collaborations). [Recommended but other alternatives acceptable; expert opinion only.]
Research into the long-term outcomes of COVID-19 encephalopathy should be undertaken in order to develop patient-centered pathways and rehabilitation guidelines that will maximize quality of life. [Strongly recommended; expert opinion only.]
Self-management programs and nonprofit third-sector organizations have important roles to play in supporting traditional rehabilitation, along with providing patient information and education on how to maximize recovery. [Recommended but other alternatives acceptable; expert opinion only.]
Clinical Approach
Patients who have recovered from COVID-19 may experience neurological, psychiatric, physical, and psycho-social difficulties, although we do not yet fully understand the long-term implications to guide rehabilitation (131). Nevertheless, these difficulties may reflect both physiological and psychological effects (e.g., PTSD), even among patients who do not require intensive care unit admission. Following hospital discharge, many patients report fatigue, cognitive symptoms, depression, anxiety, and adjustment disorders, and follow-up assessment is likely to be beneficial for many patients—and perhaps the majority—to regain their independence and return to work and/or school (132–135).
Those who have experienced encephalopathy and an acute primary CNS complication, such as brain inflammation or stroke, are likely to experience multiple physical symptoms and may require significant rehabilitation investment (136). Moreover, as many of the most severely affected patients with COVID-19 encephalopathy may have comorbid conditions, rehabilitation needs and goal setting can be even more challenging (131). Currently, there is no evidence of encephalitis lethargica presentation similar to that seen in the epidemic of the late 1800s and early 1900s, although clinicians seeing patients for rehabilitation should be alert to the possibility of delayed emergent phenotypes among patients during rehabilitation and follow-up care.
In conclusion, given the large number of patients hospitalized with COVID-19 encephalopathy and the complexity of their rehabilitation needs, these services for survivors must be prioritized, have a long-term focus, be innovative and scalable, and be delivered by multidisciplinary teams (131, 137, 138).
1 : Neurological and neuropsychiatric complications of COVID-19 in 153 patients: a U.K.-wide surveillance study. Lancet Psychiatry 2020; 7:875–882Crossref, Medline, Google Scholar
2 : Spectrum, risk factors, and outcomes of neurological and psychiatric complications of COVID-19: a U.K.-wide cross-sectional surveillance study. SSRN J 2021Crossref, Google Scholar
3 : Global incidence of neurological manifestations among patients hospitalized with COVID-19: a report for the GCS-NeuroCOVID Consortium and the ENERGY Consortium. JAMA Netw Open 2021; 4:e2112131Crossref, Medline, Google Scholar
4 : Frequent neurologic manifestations and encephalopathy‐associated morbidity in COVID‐19 patients. Ann Clin Transl Neurol 2020; 7:2221–2230Crossref, Medline, Google Scholar
5 : Frequency of neurologic manifestations in COVID-19: a systematic review and meta-analysis. Neurology 2021; 97:e2269–e2281Crossref, Medline, Google Scholar
6 : A prospective study of neurologic disorders in hospitalized patients with COVID-19 in New York City. Neurology 2021; 96:e575–e586Crossref, Medline, Google Scholar
7 : Neurology of COVID-19 in Singapore. J Neurol Sci 2020; 418:117118Crossref, Medline, Google Scholar
8 : Spectrum of neuropsychiatric manifestations in COVID-19. Brain Behav Immun 2020; 88:71–74Crossref, Medline, Google Scholar
9 : Acute encephalopathy is associated with worse outcomes in COVID-19 patients. Brain Behav Immun Health 2020; 8:100136Crossref, Medline, Google Scholar
10 : Central and peripheral nervous system complications of COVID-19: a prospective tertiary center cohort with 3-month follow-up. J Neurol 2021; 268:3086–3104Crossref, Medline, Google Scholar
11 : Neurological complications in COVID-19 patients from Latin America. Brain 2021; 144:e29Crossref, Medline, Google Scholar
12 : Neurological associations of COVID-19. Lancet Neurol 2020; 19:767–783Crossref, Medline, Google Scholar
13 : Cognitive impairments four months after COVID-19 hospital discharge: pattern, severity and association with illness variables. Eur Neuropsychopharmacol 2021; 46:39–48Crossref, Medline, Google Scholar
14 : Toxic metabolic encephalopathy in hospitalized patients with COVID-19. Neurocrit Care 2021; 35:693–706Crossref, Medline, Google Scholar
15 : Provisional case definitions for COVID-19-associated neurological disease–authors’ reply. Lancet Neurol 2020; 19:891–892Crossref, Medline, Google Scholar
16 : Updated nomenclature of delirium and acute encephalopathy: statement of ten societies. Intensive Care Med 2020; 46:1020–1022Crossref, Medline, Google Scholar
17 : Public health surveillance for COVID-19: interim guidance. Geneva, World Health Organization, 2020. www.who.int/publications/i/item/who-2019-nCoV-surveillanceguidance-2020.7. Accessed June 23, 2021Google Scholar
18 : Safety Platform for Emergency vACcines: SO2- D2.5.2.1–AESI case definition companion guide for 1st tier AESI: acute encephalitis. Brighton Collaboration, 2021. brightoncollaboration.us/wp-content/uploads/2021/03/SPEAC_D2.5.2.1_Encephalitis-Case-Definition-Companion-Guide_V1.0_format12064-1.pdf. Accessed June 23, 2021Google Scholar
19 : Prognostic indicators and outcomes of hospitalised COVID-19 patients with neurological disease: a systematic review and individual patient data meta-analysis. SSRN J 2021; PreprintGoogle Scholar
20 : Features of 20 133 U.K. patients in hospital with COVID-19 using the ISARIC WHO Clinical Characterisation Protocol: prospective observational cohort study. BMJ 2020; 369:m1985Crossref, Medline, Google Scholar
21 : Global Consortium Study of Neurological Dysfunction in COVID-19 (GCS-NeuroCOVID): study design and rationale. Neurocrit Care 2020; 33:25–34Crossref, Medline, Google Scholar
22 : Delirium and encephalopathy in severe COVID-19: a cohort analysis of ICU patients. Crit Care 2020; 24:491Crossref, Medline, Google Scholar
23 : Delirium incidence, duration, and severity in critically ill patients with coronavirus disease 2019. Crit Care Explor 2020; 2:e0290Crossref, Medline, Google Scholar
24 : Prevalence and risk factors for delirium in critically ill patients with COVID-19 (COVID-D): a multicentre cohort study. Lancet Respir Med 2021; 9:239–250Crossref, Medline, Google Scholar
25 : Delirium in older patients with COVID-19 presenting to the emergency department. JAMA Netw Open 2020; 3:e2029540Crossref, Medline, Google Scholar
26 : Delirium is common in patients hospitalized with COVID-19. Intern Emerg Med 2021; 16:1997–2000Crossref, Medline, Google Scholar
27 : Delirium in patients with SARS‐CoV ‐2 infection: a multicenter study. J Am Geriatr Soc 2021; 69:293–299Crossref, Medline, Google Scholar
28 : A call for a Global COVID-19 Neuro Research Coalition. Lancet Neurol 2020; 19:482–484Crossref, Medline, Google Scholar
29 : Management of suspected viral encephalitis in children—Association of British Neurologists and British Paediatric Allergy, Immunology and Infection Group National Guidelines. J Infect 2012; 64:449–477Crossref, Medline, Google Scholar
30 : Management of suspected viral encephalitis in adults—Association of British Neurologists and British Infection Association National Guidelines. J Infect 2012; 64:347–373Crossref, Medline, Google Scholar
31 : COVID‐19 is associated with an unusual pattern of brain microbleeds in critically ill patients. J Neuroimaging 2020; 30:593–597Crossref, Medline, Google Scholar
32 : The pattern of brain microhemorrhages after severe lung failure resembles the one seen in high-altitude cerebral edema. Crit Care Med 2015; 43:e386–e389Crossref, Medline, Google Scholar
33 : Critical illness-associated cerebral microbleeds. Stroke 2017; 48:1085–1087Crossref, Medline, Google Scholar
34 : Correlates of critical illness-related encephalopathy predominate postmortem COVID-19 neuropathology. Acta Neuropathol 2020; 140:583–586Crossref, Medline, Google Scholar
35 : Neuropathological features of COVID-19. N Engl J Med 2020; 383:989–992Crossref, Medline, Google Scholar
36 : Microvascular injury in the brains of patients with COVID-19. N Engl J Med 2021; 384:481–483Crossref, Medline, Google Scholar
37 : Histopathological findings and viral tropism in U.K. patients with severe fatal COVID-19: a post-mortem study. Lancet Microbe 2020; 1:e245–e253Crossref, Medline, Google Scholar
38 : Neuropathologic features of four autopsied COVID‐19 patients. Brain Pathol 2020; 30:1012–1016Crossref, Medline, Google Scholar
39 : Microvascular injury and hypoxic damage: emerging neuropathological signatures in COVID-19. Acta Neuropathol 2020; 140:397–400Crossref, Medline, Google Scholar
40 : Neuropathologic findings of patients with COVID-19: a systematic review. Neurol Sci 2021; 42:1255–1266Crossref, Medline, Google Scholar
41 : Human coronaviruses and other respiratory viruses: underestimated opportunistic pathogens of the central nervous system? Viruses 2020; 12:14Crossref, Google Scholar
42 : Structural basis of receptor recognition by SARS-CoV-2. Nature 2020; 581:221–224Crossref, Medline, Google Scholar
43 : COVID-19-associated acute hemorrhagic necrotizing encephalopathy: imaging features. Radiology 2020; 296:E119–E120Crossref, Medline, Google Scholar
44 : Clinical, imaging, and lab correlates of severe COVID-19 leukoencephalopathy. AJNR Am J Neuroradiol 2021; 42:632–638Crossref, Medline, Google Scholar
45 : COVID-19-related diffuse posthypoxic leukoencephalopathy and microbleeds masquerades as acute necrotizing encephalopathy. Int J Neurosci (Online ahead of print, December 30, 2020) 1–6. doi:10.1080/00207454.2020.1865346Medline, Google Scholar
46 : Severity of chest imaging is correlated with risk of acute neuroimaging findings among patients with COVID-19. AJNR Am J Neuroradiol 2021; 42:831–837Crossref, Medline, Google Scholar
47 : COVID-19-associated diffuse leukoencephalopathy and microhemorrhages. Radiology 2020; 297:E223–E227Crossref, Medline, Google Scholar
48 : Cerebral microbleeds and leukoencephalopathy in critically ill patients with COVID-19. Stroke 2020; 51:2649–2655Crossref, Medline, Google Scholar
49 : COVID-19-associated delayed posthypoxic necrotizing leukoencephalopathy. J Neurol Sci 2020; 415:116945Crossref, Medline, Google Scholar
50 : Delayed post-hypoxic leukoencephalopathy in an adult with COVID-19. J Neurovirol 2021; 27:1–5Crossref, Medline, Google Scholar
51 : Pearls & Oy-sters: leukoencephalopathy in critically ill patients with COVID-19. Neurology 2020; 95:753–757Crossref, Medline, Google Scholar
52 : Prevalence and impact of hyponatremia in patients with coronavirus disease 2019 in New York City. Crit Care Med 2020; 48:e1211–e1217Crossref, Medline, Google Scholar
53 : Clinical features, laboratory characteristics, and outcomes of patients hospitalized with coronavirus disease 2019 (COVID-19): early report from the United States. Diagnosis (Berl) 2020; 7:91–96Crossref, Medline, Google Scholar
54 : Disorders of sodium balance and its clinical implications in COVID-19 patients: a multicenter retrospective study. Intern Emerg Med 2021; 16:853–862Crossref, Medline, Google Scholar
55 : Electrolyte imbalances in patients with severe coronavirus disease 2019 (COVID-19). Ann Clin Biochem 2020; 57:262–265Crossref, Medline, Google Scholar
56 : Correlating arterial blood gas, acid-base and blood pressure abnormalities with outcomes in COVID-19 intensive care patients. Ann Clin Biochem 2021; 58:95–101Crossref, Medline, Google Scholar
57 : Acute disseminated encephalomyelitis after SARS-CoV-2 infection. Neurol Neuroimmunol Neuroinflamm 2020; 7:e797Crossref, Medline, Google Scholar
58 : A first case of meningitis/encephalitis associated with SARS-coronavirus-2. Int J Infect Dis 2020; 94:55–58Crossref, Medline, Google Scholar
59 : Clinical characteristics of 58 children with a pediatric inflammatory multisystem syndrome temporally associated with SARS-CoV-2. JAMA 2020; 324:259–269Crossref, Medline, Google Scholar
60 : Posterior reversible encephalopathy syndrome (PRES) as a neurological association in severe COVID-19. J Neurol Sci 2020; 414:116943Crossref, Medline, Google Scholar
61 : Encephalitis associated with COVID-19 infection in an 11-year-old child. Pediatr Neurol 2020; 109:94Crossref, Medline, Google Scholar
62 : Complications and pathophysiology of COVID-19 in the nervous system. Front Neurol 2020; 11:573421Crossref, Medline, Google Scholar
63 : COVID-19: consider cytokine storm syndromes and immunosuppression. Lancet 2020; 395:1033–1034Crossref, Medline, Google Scholar
64 : The cytokine storm in COVID-19: an overview of the involvement of the chemokine/chemokine-receptor system. Cytokine Growth Factor Rev 2020; 53:25–32Crossref, Medline, Google Scholar
65 : Pathophysiology of SARS-CoV-2: targeting of endothelial cells renders a complex disease with thrombotic microangiopathy and aberrant immune response: the Mount Sinai COVID-19 autopsy experience. medRxiv 2020. PreprintGoogle Scholar
66 : Neurologic aspects of coronavirus disease of 2019 infection. Curr Opin Infect Dis 2021; 34:217–227Crossref, Medline, Google Scholar
67 : Clinical features of patients infected with 2019 novel coronavirus in Wuhan, China. Lancet 2020; 395:497–506Crossref, Medline, Google Scholar
68 : Neuroimaging findings in conjunction with severe COVID-19. Rofo 2021; 193:822–829Medline, Google Scholar
69 : COVID-19-related intracranial imaging findings: a large single-centre experience. Clin Radiol 2021; 76:108–116Crossref, Medline, Google Scholar
70 : Radiographic and clinical neurologic manifestations of COVID-19 related hypoxemia. J Neurol Sci 2020; 418:117119Crossref, Medline, Google Scholar
71 : Incidence of acute kidney injury and its association with mortality in patients with COVID-19: a meta-analysis. J Investig Med 2020; 68:1261–1270Crossref, Medline, Google Scholar
72 : Outcomes for patients with COVID-19 and acute kidney injury: a systematic review and meta-analysis. Kidney Int Rep 2020; 5:1149–1160Crossref, Medline, Google Scholar
73 : High levels of soluble CD25 in COVID‐19 severity suggest a divergence between anti‐viral and pro‐inflammatory T‐cell responses. Clin Transl Immunol 2021; 10:e1251Crossref, Medline, Google Scholar
74 : Impaired natural killer cell counts and cytolytic activity in patients with severe COVID-19. Blood Adv 2020; 4:5035–5039Crossref, Medline, Google Scholar
75 : COVID‐19 encephalopathy: clinical and neurobiological features. J Med Virol 2021; 93:4374–4381Crossref, Medline, Google Scholar
76 : Cerebrospinal fluid in COVID-19: a systematic review of the literature. J Neurol Sci 2021; 421:117316Crossref, Medline, Google Scholar
77 : Non-neuronal expression of SARS-CoV-2 entry genes in the olfactory system suggests mechanisms underlying COVID-19-associated anosmia. Sci Adv 2020; 6:eabc5801Crossref, Medline, Google Scholar
78 : Neuropathology of patients with COVID-19 in Germany: a post-mortem case series. Lancet Neurol 2020; 19:919–929Crossref, Medline, Google Scholar
79 : Olfactory transmucosal SARS-CoV-2 invasion as a port of central nervous system entry in individuals with COVID-19. Nat Neurosci 2021; 24:168–175Crossref, Medline, Google Scholar
80 : Unspecific post-mortem findings despite multiorgan viral spread in COVID-19 patients. Crit Care 2020; 24:495Crossref, Medline, Google Scholar
81 : The cellular basis of loss of smell in 2019-nCoV-infected individuals. Brief Bioinform 2021; 22:873–881Crossref, Medline, Google Scholar
82 : Diagnostic and Statistical Manual of Mental Disorders, 5th ed. Washington, DC, American Psychiatric Association, 2013Crossref, Google Scholar
83 : Insight into delirium. Innov Clin Neurosci 2011; 8:25–34Medline, Google Scholar
84 : Acute toxic-metabolic encephalopathy in adults. Edited by Basow DS. UpToDate, Waltham, Mass., 2012. https://www.uptodate.com/contents/acute-toxic-metabolic-encephalopathy-in-adults. Accessed May 31, 2021Google Scholar
85 : Clarifying confusion: the Confusion Assessment Method. Ann Intern Med 1990; 113:941–948Crossref, Medline, Google Scholar
86 : Validation of the 4AT, a new instrument for rapid delirium screening: a study in 234 hospitalised older people. Age Ageing 2014; 43:496–502Crossref, Medline, Google Scholar
87 : New onset neurologic events in people with COVID-19 in 3 regions in China. Neurology 2020; 95:e1479–e1487Crossref, Medline, Google Scholar
88 : Clinical features and outcomes of patients with dementia compared to an aging cohort hospitalized during the initial New York City COVID-19 wave. J Alzheimers Dis 2021; 81:679–690Crossref, Medline, Google Scholar
89 : Neurological predictors of clinical outcomes in hospitalized patients with COVID-19. Front Neurol 2020; 11:585944Crossref, Medline, Google Scholar
90 : Neurological complications of COVID-19: underlying mechanisms and management. Int J Mol Sci 2021; 22:4081Crossref, Medline, Google Scholar
91 : COVID‐19-associated encephalopathy and cytokine‐mediated neuroinflammation. Ann Neurol 2020; 88:860–861Crossref, Medline, Google Scholar
92 : Neurological examination of critically ill patients: a pragmatic approach: report of an ESICM expert panel. Intensive Care Med 2014; 40:484–495Crossref, Medline, Google Scholar
93 : Delirium. Nat Rev Dis Primers 2020; 6:90Crossref, Medline, Google Scholar
94 : Neurologic emergencies during the coronavirus disease 2019 pandemic. Neurol Clin 2021; 39:671–687Crossref, Medline, Google Scholar
95 : Posterior reversible leukoencephalopathy syndrome in a patient after acute COVID-19 infection. Case Rep Neurol Med 2021; 2021:5564802Medline, Google Scholar
96 : Meta-analysis of EEG findings in patients with COVID-19. Epilepsy Behav 2021; 115:107682Crossref, Medline, Google Scholar
97 : Nervous system involvement in coronavirus disease 2019: results from a retrospective consecutive neuroimaging cohort. Radiology 2020; 297:E324–E334Crossref, Medline, Google Scholar
98 : How to carry out and interpret EEG recordings in COVID-19 patients in ICU? Clin Neurophysiol 2020; 131:2023–2031Crossref, Medline, Google Scholar
99 : Systematic review of EEG findings in 617 patients diagnosed with COVID-19. Seizure 2020; 83:234–241Crossref, Medline, Google Scholar
100 : CSF biomarkers in patients with COVID-19 and neurological symptoms. Neurology 2021; 96:e294Medline, Google Scholar
101 : Anti-SARS-CoV-2 antibodies in the CSF, blood-brain barrier dysfunction, and neurological outcome. Neurol Neuroimmunol Neuroinflamm 2020; 7:e893Crossref, Medline, Google Scholar
102 : High frequency of cerebrospinal fluid autoantibodies in COVID-19 patients with neurological symptoms. Brain Behav Immun 2021; 93:415–419Crossref, Medline, Google Scholar
103 : Cerebrospinal fluid findings in COVID-19 patients with neurological symptoms. J Neurol Sci 2020; 418:117090Crossref, Medline, Google Scholar
104 : Neurological presentations of COVID-19: findings from the Spanish Society of Neurology NeuroCOVID-19 Registry. J Neurol Sci 2021; 423:117283Crossref, Medline, Google Scholar
105 : Updated diagnostic criteria for paraneoplastic neurologic syndromes. Neurol Neuroimmunol Neuroinflamm 2021; 8:e1014Crossref, Medline, Google Scholar
106 : Encephalopathy in patients with COVID‐19: a review. J Med Virol 2021; 93:206–222Crossref, Medline, Google Scholar
107 : Clinical characteristics of 113 deceased patients with coronavirus disease 2019: retrospective study. BMJ 2020; 368:m1091Crossref, Medline, Google Scholar
108 : Bacterial Sepsis. Treasure Island, Fla., StatPearls Publishing, 2021Google Scholar
109 : Systemic Inflammatory Response Syndrome. Treasure Island, Fla., StatPearls Publishing, 2021Google Scholar
110 : Individualized hemodynamic management in sepsis. J Personalized Med 2021; 11:157Crossref, Medline, Google Scholar
111 : Updates in sepsis resuscitation. Emerg Med Clin North America 2020; 38:807–818Crossref, Medline, Google Scholar
112 : Analysis of noninvasive ventilation in subjects with sepsis and acute respiratory failure. Respir Care 2021; 66:1063–1073Crossref, Medline, Google Scholar
113 : Multimodal monitoring in neurointensive care. J Neurol Neurosurg Psychiatry 1996; 60:131–139Crossref, Medline, Google Scholar
114 : Oxygen therapy for the critically ill. N Engl J Med 2020; 382:1054–1056Crossref, Medline, Google Scholar
115 : Prognostic significance of body temperature in the emergency department vs. the ICU in patients with severe sepsis or septic shock: a nationwide cohort study. PLoS One 2020; 15:e0243990Crossref, Medline, Google Scholar
116 : Successful use of mild therapeutic hypothermia as compassionate treatment for severe refractory hypoxemia in COVID-19. J Crit Care 2021; 63:260–263Crossref, Medline, Google Scholar
117 : Potentially reversible and recognizable acute encephalopathic syndromes: disease categorization and MRI appearances. AJNR Am J Neuroradiol 2020; 41:1328–1338Medline, Google Scholar
118 : Cerebral imaging in patients with COVID-19 and neurological symptoms: first experience from two university hospitals in Northern Germany. Rofo 2021; 193:667–671Crossref, Medline, Google Scholar
119 : Psychopharmacology of COVID-19. Psychosomatics 2020; 61:411–427Crossref, Medline, Google Scholar
120 : Everything you need to know about the COVID-19 therapy trials. Pharm J 2022. pharmaceutical-journal.com/article/feature/everything-you-need-to-know-about-the-covid-19-therapy-trials. Accessed June 23, 2021Google Scholar
121 : Neurotrauma and Critical Care of the Brain, 2nd ed. New York, Thieme, 2018Google Scholar
122 : Cytokine storm induced by SARS-CoV-2 infection: the spectrum of its neurological manifestations. Cytokine 2021; 138:155404Crossref, Medline, Google Scholar
123 : Neutralizing monoclonal antibodies for treatment of COVID-19. Nat Rev Immunol 2021; 21:382–393Crossref, Medline, Google Scholar
124 : Clinical characteristics and outcomes of inpatients with neurologic disease and COVID-19 in Brescia, Lombardy, Italy. Neurology 2020; 95:e910–e920Crossref, Medline, Google Scholar
125 : Delirium and adverse outcomes in hospitalized patients with COVID ‐19. J Am Geriatr Soc 2020; 68:2440–2446Crossref, Medline, Google Scholar
126 : The impact of delirium on outcomes for older adults hospitalised with COVID-19. Age Ageing 2020; 49:923–926Crossref, Medline, Google Scholar
127 : Functional and cognitive outcomes after COVID-19 delirium. Eur Geriatr Med 2020; 11:857–862Crossref, Medline, Google Scholar
128 : Prevalence and prognostic value of delirium as the initial presentation of COVID-19 in the elderly with dementia: an Italian retrospective study. EClinicalMedicine 2020; 26:100490Crossref, Medline, Google Scholar
129 : Comorbidity and its impact on 1590 patients with COVID-19 in China: a nationwide analysis. Eur Respir J 2020; 55:2000547Crossref, Medline, Google Scholar
130 : COVID-19 clinical neuroscience study. Liverpool, United Kingdom, University of Liverpool, 2021. https://www.liverpool.ac.uk/covid-clinical-neuroscience-study/. Accessed June 10, 2022Google Scholar
131 : Rehabilitation after COVID-19: an evidence-based approach. Clin Med 2020; 20:359–365Crossref, Medline, Google Scholar
132 : Post-COVID-19 syndrome among symptomatic COVID-19 patients: a prospective cohort study in a tertiary care center of Bangladesh. PLoS One 2021; 16:e0249644Crossref, Medline, Google Scholar
133 : COVID-19 cognitive deficits after respiratory assistance in the subacute phase: a COVID-rehabilitation unit experience. PLoS One 2021; 16:e0246590Crossref, Medline, Google Scholar
134 : Frequency and profile of objective cognitive deficits in hospitalized patients recovering from COVID-19. Neuropsychopharmacol 2021; 46:2235–2240Crossref, Medline, Google Scholar
135 : Long-lasting cognitive abnormalities after COVID-19. Brain Sci 2021; 11:235Crossref, Medline, Google Scholar
136 : 6-month neurological and psychiatric outcomes in 236 379 survivors of COVID-19: a retrospective cohort study using electronic health records. Lancet Psychiatry 2021; 8:416–427Crossref, Medline, Google Scholar
137 : Postdischarge symptoms and rehabilitation needs in survivors of COVID‐19 infection: a cross‐sectional evaluation. J Med Virol 2021; 93:1013–1022Crossref, Medline, Google Scholar
138 : COVID-19: maintaining essential rehabilitation services across the care continuum. BMJ Glob Health 2020; 5:e002670Crossref, Medline, Google Scholar