Von Economo Neuron Involvement in Social Cognitive and Emotional Impairments in Neuropsychiatric Disorders
In 1925, the first morphological descriptions of a distinctive group of nerve cells, von Economo neurons (VENs), were published in an epic atlas depicting the cortical organization of the human brain (2). The book containing these descriptions was written by the Australian neurologist Constantin Alexander von Economo (1876–1931) and his colleague George Koskinas (1885–1975) (17). von Economo named these neurons “rod and corkscrew cells,” denoting their straight and twisted morphological variations (2, 4). VENs were described as large and spindle-shaped bipolar neurons, localized primarily within frontolimbic regions. Over the past century, VENs have been identified as specialized cells in humans and in other primates in the hominid lineage (e.g., bonobos, common chimpanzees, orangutans, and gorillas) but not nonhominid primates (e.g., particular monkeys and apes) and nonprimate mammals (e.g., crows and pigs) (3–5). They are located in the deep portion of cortical layer V (sublayer Vb), as well as in the frontoinsular (FI) cortex, prefrontal cortex, and anterior cingulate cortex (ACC) (3–5) (Figure 1), and they are widely distributed in the temporal lobe, including the entorhinal cortex, subiculum, and hippocampal formation (1, 11–13, 15) (Figures 2 and 3).
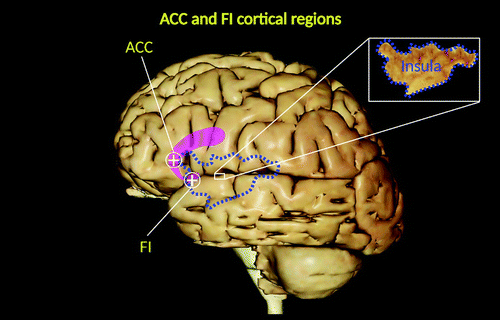
FIGURE 1. Lateral view of the left hemisphere of the human brain, emphasizing cortical regions containing von Economo neurons (VENs) in some regions of the frontal and insular lobes (1). The location of the anterior segment of the cingulate cortex is shaded pink. The blue dashed lines and inset denote the territorial boundaries of the insula (i.e., the island of Reil). VENs are localized primarily within limbic regions and specifically in the frontoinsular (FI) cortex and anterior cingulate cortex (ACC) in humans and other hominid primates (e.g., bonobos, common chimpanzees, orangutans, and gorillas) but not nonhominid primates and nonprimate mammals (2–6). The FI cortex and ACC represent key functional centers, part of a wide neuronal circuit (salience network) involved in social-emotional, homeostatic, and autonomic responses (7–10). All images were created under the terms of the Creative Commons Attribution License and created with VH Dissector and BioRender.com.
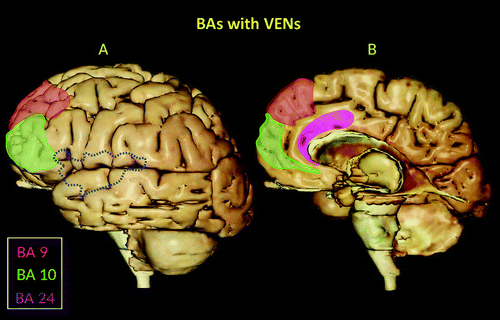
FIGURE 2. Lateral (A) and medial (B) views of the left and right hemispheres, respectively, highlighting Brodmann’s areas (BAs) with VENs. VENs are distributed throughout the medial portions of the prefrontal region (BAs 9 and 10) and the ACC (BA 24) (1, 11–15). The red shaded areas depict the location of VENs in BA 9. The green shaded areas indicate the location of VENs in BA 10. The anterior segment of the cingulate cortex (BA 24) is shaded pink. The blue dashed lines denote the territorial boundaries of the insula.
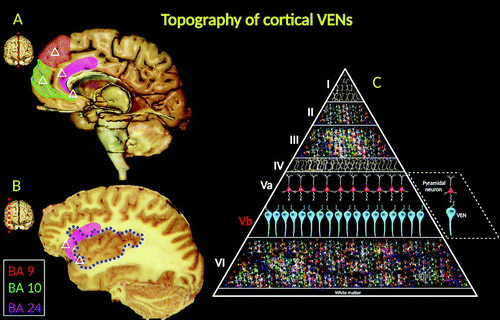
FIGURE 3. Distribution and topography of cortical VENs (indicated by small white triangles in A and B) in the human brain. Medial (A) and parasagittal (B) views are shown. The red shaded area depicts the location of VENs in BA 9. The green shaded area indicates the location of VENs in BA 10. The anterior segment of the cingulate cortex (BA 24) is shaded pink. The blue dashed lines denote the territorial boundaries of the insula. The large triangle (C) illustrates the histological topographic structure of the cerebral cortex. The roman numerals denote the cortical laminated structure. VENs have been consistently found in the deep portion of cortical layer V (sublayer Vb) (1, 11–13). VENs are projection neurons, typically exhibiting larger cylindrical soma than pyramidal nerve cells (see the parallelogram) (15). Morphologically, VENs differ from pyramidal cells in that they have a singular large basal dendrite (pyramidal neurons have multiple smaller dendrites). VENs possess a distinctive narrow dendritic arborization pattern, spanning into the laminated architecture of the cerebral cortex and possibly allowing faster relay of neuronal signals (16).
COVER. Digital depiction of the human brain, emphasizing the cerebral cortex cytoarchitecture and the morphology of a von Economo neuron as visualized in a fluorescent artistic illustration. Created under the terms of the Creative Commons Attribution License. Created with VH Dissector, Canva and BioRender.com.
Of these regions, the FI cortex and ACC represent key functional centers within a wide neural network (salience network) involved in social–emotional, homeostatic, and autonomic responses (7–10). Clinical neuroscientists have been increasingly interested in VENS because of their possible connection with mental disorders, especially those affecting social skills (i.e., social awareness, social judgment, and social humor), self-control, empathy, and self-perceived life satisfaction (1, 5, 18, 19).
Neurobiological Profiles
VENs are projection neurons, typically exhibiting larger cylindrical soma than pyramidal nerve cells (15). Morphologically, VENs differ from pyramidal cells in that they have a singular large basal dendrite; by contrast, pyramidal neurons have smaller dendrites. VENs possess a distinctive, narrow dendritic arborization pattern, spanning into the laminated architecture of the cerebral cortex and possibly allowing faster relay of neuronal signals (16) (Figure 3C).
Another distinctive feature of VENs is their structural symmetry. One study suggests that there is a high level of symmetry between the apical and basal dendrites of VENs (16). Other investigators have described apical and basal dendritic processes that appear to be perpendicular extensions toward the pial surface and the subcortical white matter, respectively (11, 13). A recent morphological study reported that the basal part of the body axis of VENs is distinctive, exhibiting a uniquely spiral and relatively thick origin of the apical stem followed by a gradual thinning. Perhaps the most peculiar characteristic of VENs is their axon, originating from the ending of the basal extension and occasionally sharing a common origin with a dendrite (20).
The cellular density distribution of VENS in humans seems to vary based on the cortical regions where VENs reside (6). Interestingly, the density of VENs appears to decrease from the ACC and insula toward the frontal surface and the superior frontal gyrus (1). Other studies have examined the embryological development of VENs in the ACC and FI cortex in humans. While neurogenesis of cortical layer V pyramidal neurons occurs approximately between gestational weeks 6.5 and 10, VENs first appear in week 36 postconception and are limited in number at birth (6). However, the number of VENs in the human brain increases significantly during the first 8 months after birth and postnatal asymmetries in VEN numbers develop, with more VENs in the right than the left FI cortex and limbic anterior region of the ACC (6).
VENs express high levels of nonphosphorylated neurofilament protein, as well as other important receptors, including vasopressin (1A), dopamine (D3), serotonin (2B), and gamma-aminobutyric acid (GABA) subunit theta (GABRQ) receptors (21–23). VENs, as well as fork cells, are intensely labeled by the bombesin peptides neuromedin B (NMB) and gastrin-releasing peptide (1). These peptides have peripheral roles regulating the release of digestive enzymes, peristaltic contractions, and immunological responses to the ingestion of toxic agents. In the insula, labeling by these peptides suggests that VENs may be involved in the neurophysiological integration of visceral interoceptive mechanisms potentiated by higher brain functions (i.e., motivation and decision making) (21, 24).
Several studies have investigated the expression of transcription factors in VENs. One study reported that VENs express the transcription factors FEZF2 and CTIP2, which regulate the developmental endpoint and differentiation of projection neurons toward that end. This finding suggests that VENs provide a potential link between certain autonomic control sites in the cortex (i.e., layer V of the FI cortex and ACC) and the brainstem or spinal cord regions (25). Another study analyzed the expression of the protein cyclic adenosine monophosphate–dependent activating transcription factor 3 (ATF3), as well as the expression of other proteins (interleukin-4 receptor alpha and NMB), in the ACC (26). In nonhuman animals, the function of ATF3 includes the regulation of pain in the spinal cord and modulatory stress responses from physiological stressors, such as liver trauma, myocardial ischemia, and seizures (27). Thus, the expression of ATF3 in VENs in the ACC suggests a possible modulatory role of these neurons in pain perception (26).
Neuropsychiatric Implications
A growing body of evidence implicates the disrupted-in-schizophrenia 1 protein (DISC1; a product of the DISC1 gene) as a genetic susceptibility factor for schizophrenia (28–32). DISC1 was initially discovered and linked to a translocation in chromosome 1 through four generations of a large Scottish family and cosegregated with a spectrum of mental illnesses, including not only schizophrenia but also schizoaffective disorder, recurrent major depression, and adolescent conduct and emotional disorders (33). Another study reported similar genetic mutations at the DISC1 carboxyl-terminus in a smaller American family with a history of mental illnesses and suggested that DISC1 gene mutations could increase the risk for schizophrenia and schizoaffective disorder (34). This gene is also associated with cell proliferation, differentiation, and migration regulatory processes, as well as with the organization of dendritic architecture (1, 25, 35). DISC1 protein is expressed in the soma and dendritic processes of VENs (6). Accordingly, DISC1 protein involvement in the regulation of dendritic structure may affect VEN morphology and cytoarchitecture organization (1, 6, 25) and, by extension, suggests a role for DISC1-related alterations of VENs in schizophrenia spectrum disorders and related psychiatric disorders.
Individuals with early-onset schizophrenia have been shown to have lower VEN density in the ACC than individuals with bipolar disorder and individuals without psychiatric diagnoses (36). Age, sex, postmortem interval, brain weight, and cortical thickness appear to have no significant impact on the reduction in VEN density in the ACC in individuals with early-onset schizophrenia (36). Ultrastructural alterations in ACC VENs, including an increased number of lysosomal aggregations, have also been observed among persons with schizophrenia. VENs in limbic structures (i.e., the ACC and FI cortex) may be neurodevelopmentally altered in schizophrenia and possibly associated with suicide among persons with psychotic disorders (36–38). Furthermore, these pathological changes possibly explain the changes in morphology and connectivity of the ACC seen among patients at risk for developing psychosis (i.e., psychosis in 22q11.2 deletion syndrome) (39).
Another neuropsychiatric condition in which the alteration or loss of VENs may be clinically relevant is frontotemporal dementia (FTD). In this neurodegenerative disorder, social behavior and emotions are characteristically affected, particularly in the behavioral variant of FTD (bvFTD) (40, 41). Seeley et al. (41) noted that FTD is associated with early, severe, and selective VEN losses (up to 74%) in the ACC-FI network. They further noted that similar VEN reductions were not observed in Alzheimer’s disease, even in its late stages. Stereological studies have indicated that VENs are selectively targeted in the early stages of bvFTD and appear to be related to impairments in empathy, self-control, and social awareness (42, 43). Other investigations have supported the idea that VENs are more susceptible to loss in persons with FTD than in a control group and in patients with Alzheimer’s disease (44, 45). Accordingly, VEN pathobiology appears to contribute to social-emotional deficits in persons with FTD (46), including empathy deficits among individuals with bvFTD (41).
The role of VENs among persons with bvFTD with a C9orf72 expansion also merits consideration. Persons with C9orf72 bvFTD have early social, emotional, and cognitive impairments that have been linked to alterations in VENs (47). A reduction in the number of VENs, including VENs expressing GABRQ in the ACC, was observed in C9orf72 bvFTD (47). The GABRQ is expressed on a monoaminergic phenotype of VEN, and its selective vulnerability in C9orf72 bvFTD was associated with impairments in social cognition but not psychotic symptoms, memory or speech impairments, or motor neuron disease (47). This suggests that this subgroup of VENs is a key modulator of social and emotional functioning and that its alteration is an important contributor to the clinical phenotype of C9orf72 bvFTD; by extension, these neurons may be similarly critical to disturbances in this domain of neuropsychiatric function in other disorders (47).
Among the other neuropsychiatric disorders linked to alterations in the number, structure, and location of VENs are autism spectrum disorders (1). Social cognitive impairments are key features of this class of disorders (40). Allman et al. (21) reported that the social disabilities observed in those with autism spectrum disorders may be related, in part, to abnormal VEN development. Additionally, reductions in VENs in the anterior insula also may result from alcohol dependence (48), possibly reflecting the sensitivity of the GABRQ VEN subgroup to GABA receptor stimulation by alcohol (49). Given the effects of chronic alcohol use disorder on socioemotional function and social cognition, the role of VENs in the development of these and related adverse outcomes from alcohol use disorder warrants further study (50).
Conclusions
VENs have a unique anatomical distribution within limbic system structures of the human brain. In particular, the ACC and FI cortex possess a high population of VENs. Although the specific function of VENs remains unknown, their exclusive topographic location within the FI cortex and ACC suggests a potential role in socioemotional function, including social cognition, and related autonomic functions. Given the role of VENs in these aspects of neuropsychiatric function, the findings linking alterations in the number, structure, or function of VENs in neuropsychiatric disorders, such as schizophrenia spectrum disorders, bvFTD, autism, and alcohol use disorders, are understandable. VENs perform novel and nonclassical cortical modulatory functions in the FI cortex and ACC, which distinguish them from most neurons within limbic, paralimbic, and prefrontal cortices. Further research is necessary to refine the function of VENs and to identify their relevance as potential therapeutic targets for the neuropsychiatric conditions in which they feature prominently.
1. : Von Economo neurons: cellular specialization of human limbic cortices? J Anat 2022; 241:20–32Crossref, Medline, Google Scholar
2. : The Cytoarchitectonics of the Adult Human Cortex [in German]. Berlin, Springer, 1925 Google Scholar
3. : Functional anatomy of cortical areas characterized by Von Economo neurons. Brain Struct Funct 2013; 218:1–20Crossref, Medline, Google Scholar
4. : A new type of special cells of the cingulate and insular lobes [in German]. Z Für Gesamte Neurol Psychiatr 1926; 100:706–712 Crossref, Google Scholar
5. : Von Economo neurons: clinical and evolutionary perspectives. Cortex 2013; 49:312–326Crossref, Medline, Google Scholar
6. : The von Economo neurons in frontoinsular and anterior cingulate cortex in great apes and humans. Brain Struct Funct 2010; 214:495–517Crossref, Medline, Google Scholar
7. : The salience network: a neural system for perceiving and responding to homeostatic demands. J Neurosci 2019; 39:9878–9882Crossref, Medline, Google Scholar
8. : Significance of the insula for the evolution of human awareness of feelings from the body. Ann N Y Acad Sci 2011; 1225:72–82Crossref, Medline, Google Scholar
9. : Dissociable intrinsic connectivity networks for salience processing and executive control. J Neurosci 2007; 27:2349–2356Crossref, Medline, Google Scholar
10. : The neuropsychological impact of insular cortex lesions. J Neurol Neurosurg Psychiatry 2010; 81:611–618Crossref, Medline, Google Scholar
11. : Two phylogenetic specializations in the human brain. Neuroscientist 2002; 8:335–346Crossref, Medline, Google Scholar
12. : The organization of the primate insular cortex. Front Neuroanat 2019; 13:43Crossref, Medline, Google Scholar
13. : Von Economo neurons are present in the dorsolateral (dysgranular) prefrontal cortex of humans. Neurosci Lett 2008; 435:215–218Crossref, Medline, Google Scholar
14. : Von Economo neurons in the human medial frontopolar cortex. Front Neuroanat 2018; 12:64Crossref, Medline, Google Scholar
15. : A neuronal morphologic type unique to humans and great apes. Proc Natl Acad Sci U S A 1999; 96:5268–5273Crossref, Medline, Google Scholar
16. : Dendritic architecture of the von Economo neurons. Neuroscience 2006; 141:1107–1112Crossref, Medline, Google Scholar
17. : Brain and aviation: on the 80th anniversary of Constantin von Economo’s (1876–1931) death. Neurol Sci 2013; 34:387–391Crossref, Medline, Google Scholar
18. : Frontoinsular cortical microstructure is linked to life satisfaction in young adulthood. Brain Imaging Behav 2021; 15:2775–2789Crossref, Medline, Google Scholar
19. : Brain activation during sight gags and language-dependent humor. Cereb Cortex 2007; 17:314–324Crossref, Medline, Google Scholar
20. : Somato-dendritic morphology and axon origin site specify von Economo neurons as a subclass of modified pyramidal neurons in the human anterior cingulate cortex. J Anat 2019; 235:651–669Crossref, Medline, Google Scholar
21. : Intuition and autism: a possible role for Von Economo neurons. Trends Cogn Sci 2005; 9:367–373Crossref, Medline, Google Scholar
22. : Spindle neurons of the human anterior cingulate cortex. J Comp Neurol 1995; 355:27–37Crossref, Medline, Google Scholar
23. : Von Economo neurons and fork cells: a neurochemical signature linked to monoaminergic function. Cereb Cortex 2018; 28:131–144Crossref, Medline, Google Scholar
24. : International Union of Pharmacology, LXVIII: mammalian bombesin receptors: nomenclature, distribution, pharmacology, signaling, and functions in normal and disease states. Pharmacol Rev 2008; 60:1–42Crossref, Medline, Google Scholar
25. : Human von Economo neurons express transcription factors associated with layer V subcerebral projection neurons. Cereb Cortex 2015; 25:213–220Crossref, Medline, Google Scholar
26. : Biochemical specificity of von Economo neurons in hominoids. Am J Hum Biol 2011; 23:22–28Crossref, Medline, Google Scholar
27. : Analysis of ATF3, a transcription factor induced by physiological stresses and modulated by gadd153/Chop10. Mol Cell Biol 1996; 16:1157–1168Crossref, Medline, Google Scholar
28. : Linking neurodevelopmental and synaptic theories of mental illness through DISC1. Nat Rev Neurosci 2011; 12:707–722Crossref, Medline, Google Scholar
29. : A review of disrupted-in-schizophrenia-1 (DISC1): neurodevelopment, cognition, and mental conditions. Biol Psychiatry 2006; 59:1189–1197Crossref, Medline, Google Scholar
30. : Disrupted-in-schizophrenia-1 (DISC1) protein disturbs neural function in multiple disease-risk pathways. Hum Mol Genet 2017; 26:2634–2648Crossref, Medline, Google Scholar
31. : Structural interaction between DISC1 and ATF4 underlying transcriptional and synaptic dysregulation in an iPSC model of mental disorders. Mol Psychiatry 2021; 26:1346–1360Crossref, Medline, Google Scholar
32. : The D2R-DISC1 protein complex and associated proteins are altered in schizophrenia and normalized with antipsychotic treatment. J Psychiatry Neurosci 2022; 47:E134–E147Crossref, Medline, Google Scholar
33. : Genomic structure and localisation within a linkage hotspot of disrupted in schizophrenia 1, a gene disrupted by a translocation segregating with schizophrenia. Mol Psychiatry 2001; 6:173–178Crossref, Medline, Google Scholar
34. : A frameshift mutation in disrupted in schizophrenia 1 in an American family with schizophrenia and schizoaffective disorder. Mol Psychiatry 2005; 10:758–764Crossref, Medline, Google Scholar
35. : Disrupted-in-schizophrenia 1 regulates integration of newly generated neurons in the adult brain. Cell 2007; 130:1146–1158Crossref, Medline, Google Scholar
36. : Von Economo neuron density in the anterior cingulate cortex is reduced in early onset schizophrenia. Acta Neuropathol 2010; 119:771–778Crossref, Medline, Google Scholar
37. : Ultrastructural alterations of von Economo neurons in the anterior cingulate cortex in schizophrenia. Anat Rec (Hoboken) 2017; 300:2017–2024Crossref, Medline, Google Scholar
38. : Neuroanatomical correlates of suicide in psychosis: the possible role of von Economo neurons. PLoS One 2011; 6:e20936Crossref, Medline, Google Scholar
39. : A mini review on the contribution of the anterior cingulate cortex in the risk of psychosis in 22q11.2 deletion syndrome. Front Psychiatry 2018; 9:372Crossref, Medline, Google Scholar
40. Diagnostic and Statistical Manual of Mental Disorders (DSM-5-TR), 5th ed, text rev, Washington, DC, American Psychiatric Association, 2022 Google Scholar
41. : Early frontotemporal dementia targets neurons unique to apes and humans. Ann Neurol 2006; 60:660–667Crossref, Medline, Google Scholar
42. : Selective functional, regional, and neuronal vulnerability in frontotemporal dementia. Curr Opin Neurol 2008; 21:701–707Crossref, Medline, Google Scholar
43. : Divergent social functioning in behavioral variant frontotemporal dementia and Alzheimer disease: reciprocal networks and neuronal evolution. Alzheimer Dis Assoc Disord 2007; 21:S50–S57Crossref, Medline, Google Scholar
44. : von Economo neurones are selectively targeted in frontotemporal dementia. Neuropathol Appl Neurobiol 2013; 39:572–579Crossref, Medline, Google Scholar
45. : Selective frontoinsular von Economo neuron and fork cell loss in early behavioral variant frontotemporal dementia. Cereb Cortex 2012; 22:251–259Crossref, Medline, Google Scholar
46. : Salience network atrophy links neuron type-specific pathobiology to loss of empathy in frontotemporal dementia. Cereb Cortex 2020; 30:5387–5399Crossref, Medline, Google Scholar
47. : Von Economo neurons are part of a larger neuronal population that are selectively vulnerable in C9orf72 frontotemporal dementia. Neuropathol Appl Neurobiol 2019; 45:671–680Crossref, Medline, Google Scholar
48. : Reduced anterior insula, enlarged amygdala in alcoholism and associated depleted von Economo neurons. Brain 2015; 138:69–79Crossref, Medline, Google Scholar
49. : The neurobiological markers of acute alcohol’s subjective effects in humans. Neuropsychopharmacology 2022 (Epub June 14, 2022)Crossref, Medline, Google Scholar
50. : Pharmacological compounds targeting emotional cognition in alcohol use disorder: a systematic review. Prog Neuropsychopharmacol Biol Psychiatry 2022; 116:110535Crossref, Medline, Google Scholar