Neuropsychiatric Significance of Subcortical Hyperintensity
Subcortical brain systems play an important role in the regulation of a variety of cognitive and emotional processes.1 The basal ganglia and thalamus, along with the surrounding limbic structures, comprise MacLean's paleomammalian brain,2 a region that serves to integrate appetitive drives with approach or avoidance to environmental stimuli. In humans, frontal–subcortical circuits comprise one of the major organizing neural networks in the brain. Discrete pathways connect prefrontal neurons sequentially to caudate, globus pallidus, dorsomedial thalamus, and finally back to the original prefrontal locus.3 These loop circuits process information supporting executive cognitive functions such as organization, motivation, and social comportment.4 This region of the brain receives its blood supply from relatively long, small-diameter, penetrating branches of the anterior and middle cerebral arteries. This unique circulatory arrangement is a consequence of the rapid evolutionary expansion of the neocortex and leaves these deep white and gray matter tissues vulnerable to ischemic injury and resulting neuropsychiatric dysfunction.
Changes in subcortical white matter and deep gray matter nuclei are often noted in the brains of elderly people. Typically, these changes appear as foci of increased signal (hyperintensity) on T2-weighted magnetic resonance (MR) imaging or as areas of low density (attenuation) on computed tomography (CT) (Figure 1A, Figure 1B, Figure 1C). Such changes were previously interpreted to reflect degeneration of the white matter typical of Binswanger's disease. More recently, similar changes have been reported in patients with Alzheimer's disease, in nondemented patients with cerebrovascular disease or psychiatric illness, and in apparently asymptomatic individuals. As a result, important questions are being raised about the frequency, pathophysiology, and potential clinical significance of these subcortical changes.
Given the frequent use of brain MR imaging in neuropsychiatry, clinicians and neuroscientists are likely to encounter patients with changes in the subcortical white and gray matter. The purpose of this article is to review relevant data on the occurrence, etiology, and correlates (both clinical and neurobiological) of these subcortical changes. The potential implications of these changes for the pathophysiology of neuropsychiatric disorders will be discussed, with a particular focus on the relationship between subcortical brain changes and aging, cognitive function, and mood in older adults.
HISTORY AND TERMINOLOGY
Pathologic changes in the subcortical white matter were noted as early as 1854 by Durand-Fardel, who described “L'atrophie interstitielle du cerveau” (brain interstitial atrophy) on macroscopic examination of the brain.5 Binswanger was the first to suggest that these white matter changes might be associated with clinical symptoms. In 1894, he reported 8 patients with a form of dementia characterized pathologically by subcortical white matter lesions and ventricular enlargement, but with strikingly normal-appearing cerebral cortex.6 Although no histology was described, he suggested that the white matter atrophy resulted from reduced perfusion caused by arteriosclerosis. Alzheimer, in 1902,7 provided histological confirmation of the cortical sparing and relatively focal white matter involvement and named the condition after his colleague, Binswanger. He also noted severe atherosclerosis of the medullary arteries as well as the presence of lacunar infarctions in the white matter, internal capsule, basal ganglia, thalamus, and pons. In a major review, Olszewski, in 1965,8 translated the publications of Binswanger and Alzheimer, described two additional neuropathologic cases of Binswanger's disease, and introduced the term subcortical atherosclerotic encephalopathy. Since then numerous reviews and case studies have provided helpful clinical and neuropathological data on patients with Binswanger's disease.9–11
More recently, emphasis has shifted toward an antemortem recognition of subcortical white and gray matter changes on brain MR imaging or CT. The observation that these changes may occur in clinically nondemented individuals has resulted in considerable confusion in terminology. Although the term Binswanger's has frequently been used as if it were synonymous with these MR imaging or CT changes, this usage is technically incorrect, and the diagnosis of Binswanger's disease should be reserved for those cases in which it is justified by clinical features such as a slowly developing dementia appearing typically in middle age.12,13
Newer terms have been introduced that refer to these imaging findings, including white matter lucency, incidental MR lesions, periventricular hyperintensity, patchy white matter lesions, leukoencephalopathy, and unidentified bright objects.14–17 Hachinski et al.18 proposed the term leukoaraiosis to refer to rarefaction (“araiosis”) or diminution of density of the white (“leuko”) matter. These terms can be criticized because they imply that the imaging changes are limited to the subcortical white matter, despite the common occurrence of deep gray matter and brainstem involvement.15,18–20 We prefer Awad and colleagues' term subcortical encephalomalacia (“malacia” meaning morbid softening or thinning), which emphasizes the anatomic distribution of the imaging changes without restricting them to white matter.19 As will be seen below, changes in subcortical white and gray matter may be produced by a variety of different pathologic processes that may be indistinguishable by radiologic findings alone.
RADIOLOGIC APPEARANCE OF SUBCORTICAL ENCEPHALOMALACIA
On CT, the white matter changes of subcortical encephalomalacia appear as areas of hypodensity that involve the periventricular and deep white matter of the centrum semiovale, as well as the deep gray matter nuclei (Figure 1A, Figure 1B, Figure 1C). The changes are frequently symmetric, may extend for varying distances into the deep white matter, and do not enhance with contrast.
Brain MR imaging is more sensitive than CT for detecting periventricular and deep white matter changes (Figure 1A, Figure 1B, Figure 1C). The changes usually appear as areas of increased signal (hyperintensity) on both proton density– and T2-weighted images; on T1-weighted images these lesions may appear hypointense; some authors take this finding as evidence of frank infarction. Fluid-attenuated inversion-recovery (FLAIR) imaging sequences are especially sensitive to these white matter changes and allow for heavily T2-weighted images without the obscuring of adjacent brain by the high CSF signal. The white matter changes on MR imaging may appear in a variety of patterns. Independent punctate or multipunctate/diffuse foci are common. Additionally, a thin, continuous or discontinuous hyperintense lining of periventricular white matter known as a “rim” is often seen. Rounded hyperintense foci at the angles of the frontal or occipital horns are referred to as “caps.” Finally, a “halo” of periventricular hyperintensity of a variable thickness and patchy distribution can extend irregularly into deep white matter. The arcuate U fibers are typically spared.
Punctate foci of hypodensity on CT or hyperintensity on proton density– and T2-weighted MR imaging images may also be seen in deep gray matter nuclei such as the basal ganglia and thalamus. The pons may also be involved.20 MR imaging is more sensitive than CT for detecting these changes. Additional neuroanatomic findings may include ventricular dilatation and cortical atrophy.15,17,21
RADIOLOGIC DIFFERENTIAL DIAGNOSIS OF SUBCORTICAL ENCEPHALOMALACIA
Subcortical encephalomalacia on brain imaging should be distinguished from similar-appearing changes produced by other disease processes. The differential diagnosis includes white matter edema from tumor, stroke, or trauma; demyelination from diseases such as multiple sclerosis or following infection, radiation, anoxia, or toxins; and the late-onset leukodystrophies.22 Typically, these conditions show diffuse involvement of the white matter, including the arcuate fibers, and the foci of increased T2 signal enhance with contrast. A variety of dementing conditions may be associated with subcortical changes, including Pick's disease, progressive subcortical gliosis, presenile glial dystrophy, vascular dementia, and normal-pressure hydrocephalus (NPH). NPH can mimic Binswanger's disease since it includes ventriculomegaly and the clinical triad of dementia, gait apraxia, and incontinence. Isotope cisternography, dynamic MR imaging, and lumbar puncture may assist in the diagnosis of NPH. Similar subcortical white and gray matter changes are occasionally noted in patients with probable Alzheimer's disease, where recent speculation has considered cerebral amyloid angiopathy as a possible cause of the subcortical lesions in some patients.23 CADASIL (cerebral autosomal dominant arteriosclerotic encephalopathy and leukomalacia) is a familial disorder characterized by diffuse cerebral amyloid angiopathy and a progressive dementia. MR imaging studies reveal diffuse subcortical hyperintensity on T2-weighted images.24
ETIOLOGY OF SUBCORTICAL ENCEPHALOMALACIA
For most elderly subjects, these disease processes are readily excluded and the subcortical encephalomalacia appears as an incidental or unexpected finding on MR or CT imaging. In these cases, the precise etiology of the subcortical changes remains unclear because of limited cliniconeuropathologic data. Periventricular hyperintensities on proton density– or T2-weighted MR imaging in the form of caps or rims are quite common in normal samples and do not appear to constitute a disease state. Histological studies suggest that the caps and rims (Figure 1A, Figure 1B, Figure 1C) reflect increased water content from a variety of structural conditions, including loosely arranged fine-fiber tracts with low myelin, a patchy loss of ependyma with astrocytic gliosis (“ependymitis granularis”), and the normal convergence of flow of interstitial fluid within the periventricular region.24,25 Periventricular hyperintensity in the form of a smooth halo (Figure 1A, Figure 1B, Figure 1C) has been associated histologically with disruption of the ependymal lining, with subependymal gliosis and concomitant loss of myelin.25
The histopathologic correlates of punctate and multipunctate/diffuse subcortical hyperintensity (SH) in the deep white matter are varied, but most evidence points to changes in perivascular tissue.25 Punctate SH usually does not represent infarction, but rather tissue changes induced by perivascular edema, impaired diffusion of nutrients through thickened vessel walls, état criblé (a state of dilated perivascular—Virchow-Robin—spaces together with sclerosis and ectasia of the medullary arteries and arterioles), or mechanical damage to the adjacent tissue induced by a water-hammer effect of pulsating arterioles. The multipunctate/diffuse SH lesions are linked to more severe tissue changes, including gliosis, edema, reduced myelination, and axonal destruction and infarction.26 The relative proportions of these various tissue changes are not readily separable with conventional MR imaging techniques. Furthermore, although the tissue changes associated with the various types of SH changes represent a continuum of severity, it has not been established whether these tissue changes truly evolve through such stages of severity.
To summarize, the imaging changes of SH (excluding mild periventricular changes such as caps and rims) appear to reflect a combination of edema, vascular ectasia, dilated perivascular spaces, demyelination, and occasionally lacunar infarctions. The pathogenesis of these structural changes is likely related to the unique anatomy of the vascular supply to the subcortical white and gray matter regions, which renders these areas vulnerable to ischemia.9,10,19,27 As noted above, these areas are perfused by long, penetrating medullary arteries that are relatively small in diameter, originate at right angles from the parent vessel, and have few or no collaterals (Figure 2). These anatomic features predispose the medullary vessels to arteriosclerotic damage, which results in decreased perfusion of the end zone or watershed areas that they supply.25 Clinicopathologic changes might then evolve that are indistinguishable by MR imaging alone, along a spectrum from asymptomatic état criblé in the earliest stages, to edema and white matter tract degeneration, to the accumulation of lacunar and nonlacunar infarctions and clinically symptomatic cerebrovascular disease such as Binswanger's disease and vascular dementia. Contributing to this process would be a variety of other conditions that might affect perfusion of the subcortical regions, including hypertensive arteriosclerosis, chronic recurrent hypotension, cerebral amyloid angiopathy, senile arteriolar hyalinosis, age-related meningeal thickening, and diminished autoregulation of cerebral circulation associated with aging.
Thus, as was conceptualized by Awad et al.,19 SH may actually reflect “wear and tear” of brain parenchyma that accompanies aging and chronic cerebrovascular disease. It should be noted, however, that progressive vasculopathy per se does not account for all visible subcortical MR imaging lesions. A wide variety of other lesions can mimic the MR imaging appearance of SH, including telangiectasias, isolated white matter infarcts, diverticula of the trigone of the lateral ventricle, and even asymptomatic zones of multiple sclerosis.28 Furthermore, in a few cases of severe vascular disease in the deep subcortical regions, postmortem MR imaging has been normal.19,28 Clearly, additional neuropathologic studies are needed to resolve these issues.
CLINICAL CORRELATES OF SUBCORTICAL HYPERINTENSITY
Subcortical Hyperintensity in Aging
Numerous MR imaging studies have demonstrated that aging is associated with an increased occurrence and severity of SH.29–33 The precise frequency and distribution of SH in usual or normal aging are difficult to determine, however, given differences between studies in subject sample (age range, health status, etc.), imaging technology, and lesion definition and measurement. In our study of healthy adults with no lifetime history of neurologic or psychiatric illness, SH was present in the deep white matter in 48 subjects (64.0%), in the periventricular white matter in 9 (12.0%), in the basal ganglia in 9 (12.0%), in the thalamus in 4 (5.3%), and in the pons in 16 (21.3%).34 We found that the age-specific odds of subcortical hyperintensity increased by 5% to 9% per year of age, depending on the anatomical region involved (Figure 3). The literature consistently demonstrates that patients with risk factors for vascular disease (e.g., smoking, diabetes mellitus, hypertension) and those with a high medical burden have a greater prevalence and severity of SH. Indeed, evidence on MR imaging of old cerebral “microbleeds” is reported in approximately 6% of neuropsychiatrically normal community volunteers and is associated with more extensive SH.35 Recent data in twins suggest that genetic factors may also contribute to individual differences in volume of subcortical hyperintensity.36
Little is known about the natural history of SH in usual aging. Lesion progression has been documented in longitudinal imaging studies with follow-up of from 2 to 5 years.37–39 In about half of the subjects the worsening was minor, whereas in the remainder more extensive changes were observed.37 Reported risk factors for progression are diastolic hypertension and lesion severity.37,38
An emerging literature suggests that SH may have functional and clinical significance in normal elders. Quantitative EEG studies in normal aging show a relationship between SH and EEG abnormalities, most notably decreased coherence (a measure of shared electrical activity between brain regions) in the frontal and visual networks of connections.40 In contrast, studies of evoked related potentials found no relationship between SH and P300 latency in normal elderly subjects.41 Metabolic brain imaging studies have reported reductions in cerebral blood flow and metabolism among healthy elderly volunteers with relatively large volumes of SH.42–44 These metabolic changes may be especially prominent in the frontal lobes,42 where the SH lesions predominate. MR imaging has recently been used to examine the functional correlates of SH.45 On MR spectroscopy, SH has been associated with a decreased ratio of adenosine triphosphate/inorganic phosphate, but not with any changes in phosphocreatine, phosphomonoester, or phosphodiester,46 and with an increased ratio of choline/N-acetyl aspartate (NAA), but not with any changes in creatine/NAA or lactate/NAA.47
Most of the research on the clinical significance of SH has focused on vascular events, equilibrium, and cognition. Given its presumed vascular etiology, it is not surprising that in patients with clinical vascular disease, SH is predictive of vascular events,48 ischemic or hemorrhagic stroke,49,50 and vascular death.48 In patients with transient ischemic attack or minor stroke within the past 6 months, SH also predicts risk of hemorrhagic complications from oral anticoagulation with phenprocoumon.50 Less is known about the impact of SH upon risk of overt cerebrovascular disease in normal elders.
Fibers essential for proper gait and balance course through the periventricular region. Several studies have found relationships between the extent of periventricular SH and impairment in equilibrium among elderly patients with complaints of disturbed balance51–53 as well as in asymptomatic elderly volunteers.54–56
Although a growing literature exists on the relation between cognition and changes in subcortical white and gray matter in normal aging (Table 1A, Table 1B, Table 1C, Table 1D, Table 1E), these results are conflicting, and the studies are difficult to compare given methodological differences. Most studies find no relationship between SH and scores on dementia rating scales,57–62 but such measures may be relatively insensitive to subtle changes in cognition. Positive findings have generally been more common among studies using more sensitive neuropsychological assessment batteries, with measures of frontal lobe function demonstrating the closest relationship with SH.36,37,42,57,63–67,69 Most57,70 but not all39 of the small number of longitudinal studies find associations between SH and cognitive decline, but the correlations are generally small and thus account for a relatively small proportion of the variance in test scores (Table 1A, Table 1B, Table 1C, Table 1D, Table 1E).
In an early study from our laboratory,71 we examined relationships between SH and two specific neuropsychological instruments: the Benton Facial Recognition Test72 and the WAIS-R Digit Symbol. The former was chosen because it had yielded the highest level of significance of any test reported to be associated with SH; the latter, because it had been reported to be related to SH by two independent groups (Table 1A, Table 1B, Table 1C, Table 1D, Table 1E). In addition, both the Benton Facial Recognition Test and the Digit Symbol subtest of the Wechsler Adult Intelligence Scale–Revised (WAIS-R)73 were favored a priori because subcortical pathology might be expected to disrupt visuospatial perception and visuomotor execution, respectively. We found that performance on both tests was highly related to age and education, but not to the presence of SH. The majority of our subjects had relatively mild findings of SH, however, and it thus remains possible that more severe changes that reach a certain “threshold” volume might be associated with cognitive dysfunction in otherwise healthy adults.42,61,62,64,65 Indeed, extensive damage to subcortical white matter tracks would disrupt frontal-subcortical circuitry and possibly provide an anatomical substrate for the mental slowing and disturbed executive functioning seen with aging. Lesion location may also be important, as evidenced by the work of DeGroot et al.,65 who found relationships between cognitive dysfunction and periventricular SH but not deep white matter SH, when each was analyzed conditional upon the other.
In summary, the occurrence of SH increases with age, and its severity increases in the presence of risk factors for vascular disease or high medical burden. Pathologic correlates of SH vary but appear to reflect the effects of brain hypoperfusion. The presence and severity of SH are associated with changes in brain electrophysiology, blood flow, and metabolism, as well as with dysequilibrium and alterations in cognitive (especially frontal lobe) functioning in normal elderly subjects.
Subcortical Hyperintensity in Patients With Dementia
The precise frequency, severity, and distribution of SH in patients with dementia is difficult to determine because of methodological differences between studies in their subject samples (e.g., inclusion of patients with underlying vascular disease), imaging technology, and definitions of SH (Table 2A, Table 2B, Table 2C, Table 2D). In general, the literature suggests that patients with vascular dementia have a greater frequency and severity of SH (both periventricular and deep white matter) than do patients with Alzheimer's disease or control subjects,74–83 consistent with the presumed vascular etiology of SH (Table 2A, Table 2B, Table 2C, Table 2D). Thus, SH has been identified in 33% to 97% of patients with vascular dementia75–78 compared with 5% to 56% of patients with Alzheimer's disease.75,76,79–83
The data are less clear in studies of Alzheimer's disease, again in part because reports differ in the inclusion of patients with risk factors for vascular disease and in the definition of lesions58,60,84–97 (Table 2A, Table 2B, Table 2C, Table 2D). Most studies examining SH in the deep white matter do not find a greater prevalence of SH in patients in patients with Alzheimer's disease than control subjects (Table 2A, Table 2B, Table 2C, Table 2D). Conflicting findings have been reported for periventricular SH, perhaps due in part to the methodological differences described above (Table 2A, Table 2B, Table 2C, Table 2D).
In the one published study of dementia with Lewy bodies, Barber et al.94 found a higher prevalence of SH (both periventricular and deep white matter) in patients than control subjects.
The pathophysiology of SH in patients with Alzheimer's disease is not known but is presumed to be similar to that in control samples, namely a subcortical microvasculopathy.15,98,99 No relationship has been found between apolipoprotein E E4 allele and the occurrence of SH in patients with probable Alzheimer's disease, vascular dementia, or dementia with Lewy bodies.100,101
Few studies have addressed brain anatomic correlates of SH in patients with Alzheimer's disease.90,91 Periventricular SH appears to be associated with ventricular dilation (Table 2A, Table 2B, Table 2C, Table 2D). Fazekas et al.85 have proposed that ventricular enlargement caused by the loss of periventricular fibers in Alzheimer's disease may disrupt the integrity of the ventricular lining. This disruption may result in increased transependymal flow of CSF into the periventricular white matter with resulting imaging changes of SH.
Data conflict regarding the relationship between SH and cognitive function in patients with Alzheimer's disease (Table 2A, Table 2B, Table 2C, Table 2D). Studies using global screening instruments such as the Mini-Mental State Examination (MMSE) or Cognitive Capacity Screening Examination generally show no effect of SH on global cognitive functioning.87,88,90,93,102–104 One exception is Bondareff et al.,101 who noted a correlation between a volumetric measurement of SH (the “lesion:brain ratio”) and lower MMSE scores. Positive findings are more common among studies that employ a more extensive neuropsychological test battery,103,105–107 although negative findings have also been reported.108 Cognitive domains most commonly affected include attention,107 tactile identification of objects,103 and frontal lobe function (Table 2A, Table 2B, Table 2C, Table 2D), although the amount of variance in cognition accounted for by the SH is typically quite small.105
The various functional and clinical consequences of SH in dementia have been examined in several studies. Deep white matter/basal ganglia SH has been associated with reductions in global cerebral perfusion;109 frontal perfusion;110 bilateral perfusion in frontal lobe, basal ganglia, and thalamus;108 and reduced hippocampal perfusion90 in studies using single-photon emission computed tomography (SPECT). Other SPECT studies, however, show no correlation between SH and cerebral perfusion in patients with Alzheimer's disease.111 Yamaji et al.,112 using positron emission tomography (PET), noted significantly lower global cerebral blood flow in their cohort of patients with Alzheimer's disease and SH versus patients without SH. It is of interest that oxygen extraction was increased in involved areas, suggesting adequate compensation for the reduction in blood flow. This finding may account for the number of studies that show little or no cognitive impairment in the setting of SH.
Other reported clinical correlates of SH in patients with Alzheimer's disease include a higher risk of subsequent stroke,113 higher apathy scores, motor incoordination and gait disturbance,108 urinary incontinence, and purposeless activity such as wandering.114
Barber et al.94 reported that frontal white matter hyperintensities were associated with depressive symptomatology in patients with Alzheimer's disease, dementia with Lewy bodies, or vascular dementia. They also observed that occipital white matter hyperintensities were associated with the absence of visual hallucinations.
In a one-year longitudinal study, Lopez et al.115 found no difference in cognitive change between patients with Alzheimer's disease and periventricular SH versus those patients without SH. In addition, the frequency of major depression, delusions, and hallucinations did not differ between the two groups. However, the presence of periventricular SH did predict the development of clinically significant cerebrovascular disease.
In summary, both periventricular and deep white matter/basal ganglia hyperintensities appear to be more common and generally more severe in patients with vascular dementia than in those with probable Alzheimer's disease or Lewy body dementia, and all three groups may have more extensive changes than healthy age-matched control subjects. In patients with dementia, more extensive or severe SH may be associated with brain structural (especially ventricular enlargement) and metabolic changes; with impairment in cognition, gait, and motor coordination; with depressive symptoms (especially for frontal SH); and with subsequent risk of stroke.
Subcortical Hyperintensity in Patients With Mood Disorders
It has been known for some time that patients with Binswanger's disease may present not only with dementia but also with a variety of other neuropsychiatric symptoms, including delirium, behavioral disturbances, mania, psychosis, social withdrawal, abulia, and depression.9,11,14,15,17
Early clinical reports suggested an increased rate of SH in elderly patients with severe depression referred for ECT14,15,17,116 or in patients with bipolar illness.117 Subsequently a large number of controlled investigations demonstrated that SH is indeed more common or severe in patients with major depression or bipolar disorder (Table 3A, Table 3B, Table 3C, Table 3D, Table 3E118–147). The precise frequency of SH in these populations is difficult to determine, however, given differences between studies in subject samples (e.g., in age, sex, and health status including vascular risk factors or disease), in imaging technology, and in lesion definition and measurement (Table 3A, Table 3B, Table 3C, Table 3D, Table 3E). A recent meta-analytic study148 of the extant literature reported a common odds ratio (Mantel-Haenszel technique) of 3.2 (95% confidence intervals of 2.11 and 4.82) for SH in patients with major depression, and 3.29 (95% CI of 2.14 and 5.07) in patients with bipolar disorder. The SH lesions in patients with mood disorders are typically localized to the frontal white matter and the subcortical gray nuclei (thalamus, basal ganglia; Table 3A, Table 3B, Table 3C, Table 3D, Table 3E). As noted above for nonpatient samples, SH in patients with mood disorders is associated with age and overall medical burden, including risk factors for vascular disease (Table 3A, Table 3B, Table 3C, Table 3D, Table 3E). As such, SH in patients with mood disorders is also associated with other age- or illness-related changes in brain structure, including global or regional atrophy and ventricular enlargement (Table 3A, Table 3B, Table 3C, Table 3D, Table 3E). Finally, SH and microinfarction are associated with depression in patients with vascular dementia.149
The pathophysiology of SH in patients with mood disorders is not known but is presumed to be similar to that in control samples, that is to say a subcortical microvasculopathy.15,98,99 Little is known about the natural history of SH in patients with mood disorders. One study of 7 relatively young adults with bipolar disorder found no changes in SH ratings at 1-year follow-up.134 In a prospective study of the brain anatomic effects of ECT, we observed an increase in the severity of SH 6 months after a course of ECT in 5 of 35 patients with depression.150 Such changes were interpreted to reflect progression of ongoing cerebrovascular disease rather than an effect of ECT.
Although studies in control samples have demonstrated functional brain correlates of SH by using quantitative EEG, PET, functional MR imaging, and MR spectroscopy as noted above, very few such data have been reported for patients with mood disorders.151,152 Ebmeier et al.153 found a correlation between periventricular SH and widespread periventricular and bilateral temporal perfusion defects on PET among 39 elderly patients with major depression. No relationships were observed for SH in the deep white matter. We observed a relationship between SH in the pons and shortened REM sleep latency in patients with major depression, a finding of interest given that REM sleep is felt to be mediated by systems located in the pons.154
A growing literature has focused on the clinical significance of SH in patients with mood disorders. Onset of major depression at a late age (>60 years old) has been associated with SH in most15,17,123,155–160 but not all119,120,125,126 studies of patients with unipolar disease, as described below. Most studies in patients with bipolar disorder find no relationship between SH and age at onset.134,144,145 Most reports find no relationship between SH and psychotic symptoms128,134,138,145 or severity of depression.17,58,129,134,138,156,161 Apathy, anhedonia, and functional disability have been suggested as possible correlates of SH in elderly patients with major depression.162 No relationship between SH and a family history of mood disorder has been found for patients with major depression,155 but conflicting findings have been reported for patients with bipolar disorder.134,140,145,147 Although SH is associated with dysequilibrium in elderly control samples as mentioned above, no study has examined such relationships in elderly patients with mood disorders.
Several studies have found a relationship between SH and cognitive impairment in patients with major depression.17,58,155,156,161,163 The few studies that have reported negative findings typically used screening measures rather than more sensitive neuropsychological test batteries119,129 (Table 3A, Table 3B, Table 3C, Table 3D, Table 3E). Consistent with the effects seen in control samples as described above, the cognitive disturbance is typically manifest as cognitive slowing, memory deficits, and frontal lobe/executive dysfunction (Table 3A, Table 3B, Table 3C, Table 3D, Table 3E). Conflicting findings have been reported for bipolar disorder.134,147
Little is known about the impact of SH on treatment outcomes in patients with mood disorders. In a small (N=39) study of older inpatients with severe (mainly psychotic) depression, Hickie et al.155 found that SH was associated with a poorer response to either pharmacotherapy or ECT. While our experience is consistent with the former finding, we observe a good response to ECT even in depressed patients with extensive SH.14,15,17,150 We and others have found, however, that the presence or severity of SH may be predictive of treatment side effects, including delirium from ECT116 or antidepressant medications164 as well as neuroleptic-induced parkinsonism.165 Similar observations were reported by Fujikawa et al.166 in a study of patients with major depression and silent cerebral infarction on brain MR imaging. They found that those patients with silent cerebral infarction (SH or cortical infarct) had a greater frequency of “adverse central nervous system reactions” to antidepressant drugs; these reactions included delirium, parkinsonism, dyskinesia, and akathisia.
With regard to the long-term prognosis of patients with SH and mood disorders, one group found a correlation between SH and number of prior hospitalizations but not duration of current bipolar illness.134,140 In a longitudinal investigation of mainly older patients with depression, Hickie et al.167 found that cognitive decline and functional impairment were associated with the severity of SH on MR imaging when SH were noted 6 months to 2 years previously.
The association of SH and mood disorders has led to interesting speculation about the direction of causation. Clearly, the occurrence of SH will be greater in patients with overt vascular disease, but as noted in Table 3A, Table 3B, Table 3C, Table 3D, Table 3E, the relationship between SH and mood disorders remains significant in some studies even after adjustment for medical burden and traditional vascular risk factors. The association between SH and late-age-onset mood disorders has also led to the suggestion that SH or the vascular disease with which it is associated may be causative of the depression, at least in some patients.162,168,169 Consistent with this view is the finding of a lack of family history of mood disorder in patients with SH and depression.155 Indeed, depression is a common symptom of subcortical brain disease (e.g., Parkinson's disease, Huntington's disease, multiple sclerosis) and the frontal predominance of the SH lesions in patients with mood disorders positions such lesions to disrupt (disconnect?) frontal-subcortical circuits that appear to be important in regulation of emotional behavior.17,170,171 At a neurochemical level, one report suggests that SH in geriatric depression is associated with reduced platelet [3H]-imipramine binding, a potential marker for presynaptic serotonergic function.172 Another potential causative mechanism is suggested by the association of SH and cognitive impairment; such impairment might result in limited coping strategies with which to respond to stress, leading to depression.160
There are other lines of evidence to suggest, however, that depression may be causative of cerebrovascular disease. Depression is associated with a variety of pathophysiologic alterations that may increase vulnerability to vascular disease,173 including increased platelet activation.174 Indeed, recent prospective data demonstrate an association between baseline depressive symptoms and subsequent stroke as well as stroke mortality,175–177 even after adjustment for established stroke risk factors. Treatment of depression with medications that induce hypotension could also theoretically cause or worsen SH and cerebrovascular disease,118 but no such relationships have been found.134,135,138,140,145 There is no evidence that SH in patients with mood disorders is causally related to treatment with ECT.17,120,129,145,150
Of course, as noted by Videbech,148 association does not prove causation, and there are other possible mechanisms to explain the relationship of SH and mood disorders. For example, the relationship may be mediated through a nonrelated confounder (e.g., medical burden), or SH could even be a result of treatment of depression (although, as noted above, the data do not appear to support this latter contention). Clearly, our understanding of the relationships between SH and mood disorders requires longitudinal studies of the development of SH and of mood disturbance, using appropriately matched control groups and standardized techniques for lesion assessment.
In summary, the prevalence and severity of SH is increased in elderly patients with major depression, in patients with bipolar disorder, and possibly in non-elderly patients with major depression. The SH in these populations is presumed to reflect subcortical microvascular disease, but the precise etiology of the vasculopathy is unknown. The SH may be associated with late-age onset of the major depression, cognitive impairment, a relatively poorer response to treatment, and an increased risk of cerebral side effects from treatment. The association of SH and mood disorders has led to interesting speculation on the mechanisms of mood disorder in patients with cerebrovascular disease.
Subcortical Hyperintensity in Patients With Other Mental Disorders
Most brain MR imaging studies of schizophrenia emphasize changes in frontotemporal and ventricular structures.178 More recently, SH has been described in some patients with late-onset schizophrenia179,180 and late-onset atypical psychosis.181 Sachdev and Brodaty180 reported that subjects with late-onset schizophrenia had greater periventricular SH than either age-matched subjects with onset of schizophrenia before age 50 or normal control subjects. In addition, the late-onset schizophrenia group had more discrete lesions in the thalamus than the other subjects. This study did not control for the presence of cerebrovascular risk factors. The authors postulate that cerebrovascular disease may promote the onset of psychosis in vulnerable individuals. These reports are consistent with our findings of an association between SH and late-age-onset psychiatric disorder, and they warrant further study.
SUMMARY
SH is now recognized as a common finding on brain MR imaging and CT of elderly individuals. Current clinical and neuropathologic data suggest that SH likely reflects in part abnormal water content and a spectrum of structural changes (which are difficult to distinguish by MR imaging alone), resulting from the effects of hypoperfusion of subcortical brain regions. However, because other lesions may mimic the appearance of SH and because even severe vascular disease may appear normal on postmortem MR imaging, additional neuropathologic and in vitro MR imaging studies are needed to clarify the potential etiologies of these brain imaging changes.
SH has been identified in the normal elderly population, but the lesions generally are not very extensive or severe. More data are needed to determine the effects of SH on cognitive functioning in the normal population. SH appears to be more common and generally more severe in patients with dementia, especially vascular dementia, but the severity of the cognitive impairment has not been found to be closely correlated with that of the disease process. Although little is known about the psychiatric manifestations of SH, it appears to be surprisingly common in elderly depressed patients (especially those referred for ECT), where it may serve as a risk marker for the development of late-age-onset mood disorder. The precise pathophysiologic mechanisms linking SH to clinical disorders such as dementia and late-age-onset mood disorder are unknown. The clinical implications of SH for the diagnosis, clinical phenomenology, treatment, and long-term prognosis of patients with neuropsychiatric illness require further study.
Although brain imaging may be indicated in some patients with dementia or mood disorders, current data do not support such testing solely for the purpose of “ruling out” SH in these groups. It is reasonable for the clinician to assume that SH will be present in any patient with vascular disease or risk factors or with high medical disease burden. Likewise, if SH is revealed as part of the evaluation of a patient, it may be taken as evidence of brain microvascular disease, assuming other etiologies discussed above have been excluded. In such patients, interventions aimed at the vascular disease may be appropriate. Indeed, given the potential neuropsychiatric implications of brain microvascular disease, clinicians should become proficient at helping their patients take steps to reduce the risk of vascular disease (e.g., stop smoking, maintain a healthy lifestyle, control blood pressure). In such cases, the proverbial ounce of prevention may be worth a pound of cure.
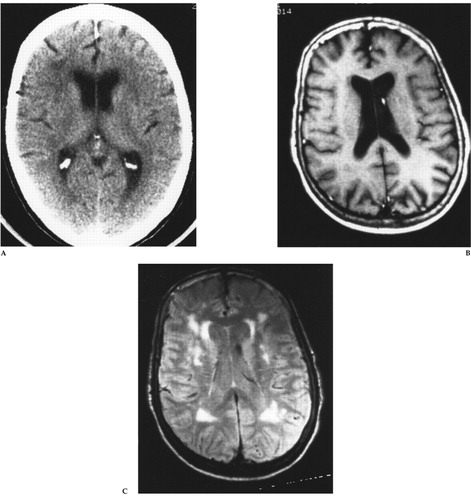
FIGURE 1. (A) Axial brain CT, (B) T1-weighted spin-echo MR (TR=500 ms, TE=20 ms), and (C) intermediate T2-weighted spin-echo MR (TR=2,500 ms, TE=40 ms) images at the level of the body of the lateral ventriclesThis 72-year-old woman was referred for ECT treatment of late-age-onset, recurrent major depression with melancholia and severe suicidal ideation that had been refractory to antidepressant drug therapy. The brain CT scan (A) revealed mild decreased density of the periventricular white matter bilaterally. The T1-weighted MR image (B) is normal, but the intermediate T2-weighted MR image (C) reveals multiple areas of tissue abnormality (“hyperintensity”) scattered throughout the periventricular and deep white matter, as well as in the basal ganglia bilaterally. Reprinted with permission from Coffey CE, Figiel GS, “Neuropsychiatric significance of subcortical encephalomalacia,” in Psychopathology and the Brain, edited by Carroll BJ, Barrett JE (New York, Raven, 1991).
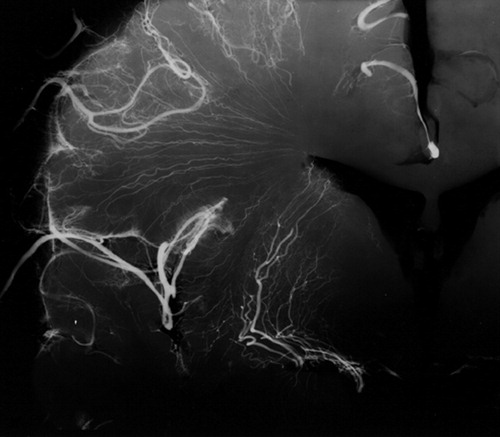
FIGURE 2. Postmortem radiograph of a coronal section of a normal cerebral hemisphere after injection of a barium sulfate-gelatin suspension into the middle cerebral arteryThis X-ray demonstrates that the deep white matter and gray matter nuclei (basal ganglia, thalamus) are irrigated by long, penetrating medullary arteries that are relatively small in diameter, exit at right angles from their parent vessel, and give few or no collaterals. As such, the regions supplied by these vessels constitute a “watershed” territory susceptible to ischemia in the presence of decreased cerebral perfusion. Reprinted with permission from Coffey CE, Figiel GS, “Neuropsychiatric significance of subcortical encephalomalacia,” in Psychopathology and the Brain, edited by Carroll BJ, Barrett JE (New York, Raven, 1991).
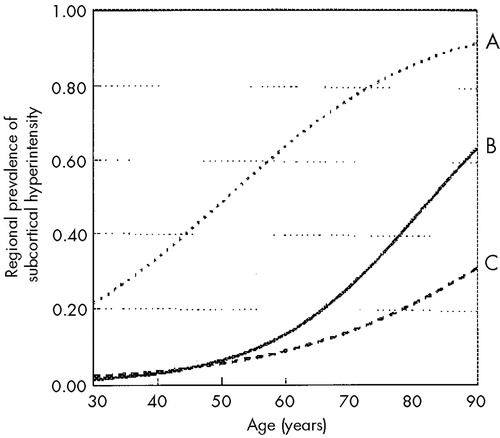
FIGURE 3. Increase in the regional prevalence of subcortical hyperintensity (SH) with ageLogistic regression models indicated that the risk increased significantly with age in the deep white matter (line A) and pons (line B), but not in the periventricular white matter or basal ganglia (line C). The odds of SH increased by approximately 6.3% per year in the deep white matter and by 8.1% in the pons. Adapted from Coffey et al. 1992.34
![]() |
![]() |
![]() |
![]() |
![]() |
![]() |
![]() |
![]() |
![]() |
![]() |
![]() |
![]() |
![]() |
![]() |
1 Cummings JL, Coffey CE: Neurobiological basis of behavior, in Textbook of Geriatric Neuropsychiatry, 2nd edition, edited by Coffey CE, Cummings JL. Washington, DC, American Psychiatric Press, 2000, pp 81-105Google Scholar
2 MacLean PD: The triune brain, emotion and scientific bias, in The Neurosciences: Second Study Program, edited by Schmitt FO. New York, Rockefeller University Press, 1970, pp 336-349Google Scholar
3 Alexander GE, DeLong MR, Strick PL: Parallel organization of functionally segregated circuits linking basal ganglia and cortex. Annu Rev Neurosci 1986; 9:357-381Crossref, Medline, Google Scholar
4 Mega MS, Cummings JL: Frontal-subcortical circuits and neuropsychiatric disorders. J Neuropsychiatry Clin Neurosci 1994; 6:358-370Link, Google Scholar
5 Hauw JJ: Leuko-ariosis: the brain interstitial atrophy of Durand-Fardel (letter). Arch Neurol 1988; 45:140Google Scholar
6 Blass JP, Hoyer S, Nitsch R: A translation of Otto Binswanger's article “The Delineation of the Generalized Progressive Paralyses.” Arch Neurol 1991; 48:961-972Crossref, Medline, Google Scholar
7 Alzheimer A: Die Seelenstorungen auf arteriosclerotischer Grundlage. Allegemeine Z Psychiatrie Psychischger Med 1902; 59:695-711Google Scholar
8 Olszewski J: Subcortical arteriosclerotic encephalopathy. World Neurol 1965; 3:359-374Google Scholar
9 Babikian V, Ropper AH: Binswanger's disease: a review. Stroke 1987; 18:2-12Crossref, Medline, Google Scholar
10 Roman GC: Senile dementia of the Binswanger's type: a vascular form of dementia in the elderly. JAMA 1987; 258:1782-1788Google Scholar
11 Caplan LR, Schoene WC: Clinical features of subcortical arteriosclerotic encephalopathy (Binswanger disease). Neurology 1978; 28:1206-1215Google Scholar
12 Binswanger's encephalopathy (editorial). Lancet 1981; i:923Google Scholar
13 Rosenberg GA: Binswanger's disease (letter). Arch Neurol 1986; 43:641Crossref, Medline, Google Scholar
14 Coffey CE, Hinkle PE, Weiner RD, et al: Electroconvulsive therapy of depression in patients with white matter hyperintensity. Biol Psychiatry 1987; 22:629-636Crossref, Medline, Google Scholar
15 Coffey CE, Figiel GS, Djang WT, et al: Leukoencephalopathy in elderly depressed patients referred for ECT. Biol Psychiatry 1988; 24:143-161Crossref, Medline, Google Scholar
16 Kertesz A, Black SE, Tokar G, et al: Periventricular and subcortical hyperintensities on magnetic resonance imaging. Arch Neurol 1988; 45:404-408Crossref, Medline, Google Scholar
17 Coffey CE, Figiel GS, Djang WT, et al: White matter hyperintensity on magnetic resonance imaging: clinical and neuroanatomic correlates in the depressed elderly. J Neuropsychiatry Clin Neurosci 1989; 1:135-144Link, Google Scholar
18 Hachinski VC, Potter P, Merskey H: Leuko-ariosis. Arch Neurol 1987; 44:21-23Crossref, Medline, Google Scholar
19 Awad IA, Johnson PC, Spetzler RF: Incidental subcortical lesions identified on magnetic resonance imaging in the elderly, II: post mortem correlations. Stroke 1986; 17:1090-1097Google Scholar
20 Solomon A, Yeates AE, Burger PC: Subcortical arteriosclerotic encephalopathy: brain stem findings with MR imaging. Radiology 1987; 165:625-629Crossref, Medline, Google Scholar
21 Coffey CE, Figiel GS, Djang WT, et al: Effects of ECT upon brain structure: a pilot prospective magnetic resonance imaging study. Am J Psychiatry 1988; 145:701-706Crossref, Medline, Google Scholar
22 Holland BA: Diseases of the white matter, in Magnetic Resonance Imaging of the Central Nervous System, edited by Brandt-Zawadzki M. New York, Raven, 1987, pp 259-277Google Scholar
23 Roullet E, Gray FF, Dubas F: Leuko-ariosis in severe amyloid angiopathy (letter). Arch Neurol 1988; 45:140-141Google Scholar
24 Sze G, DeArmond SJ, Brandt-Zawadzki, et al: Foci of MRI signal (pseudolesions) anterior to the frontal horns: histologic correlations of a normal finding. Am J Radiol 1986; 147:331-337Google Scholar
25 Fazekas F, Schmidt R, Scheltens P: Pathophysiologic mechanisms in the development of age-related white matter changes of the brain. Dement Geriatr Cogn Disord 1998; 9(suppl 1):2-5Google Scholar
26 Braffman BH, Zimmerman RA, Trojanowski JQ, et al: Brain MR: pathologic correlation with gross and histopathology, II: hyperintense white matter foci in the elderly. Am J Neuroradiol 1988; 9:629-636Google Scholar
27 Pantonini L, Garcia JH: Pathogenesis of leukoaraiosis: a review. Stroke 28:652-659, 1997Crossref, Medline, Google Scholar
28 Kirkpatrick JB, Heyman LA: White matter lesions in MRI imaging of clinically healthy brains of elderly subjects: possible pathologic basis. Radiology 1987; 162:509-511Crossref, Medline, Google Scholar
29 Coffey CE: Anatomic imaging of the aging human brain, in Textbook of Geriatric Neuropsychiatry, edited by Coffey CE, Cummings JL. Washington, DC, American Psychiatric Press, 1994, pp 159-194Google Scholar
30 Coffey CE: Anatomic imaging of the aging human brain: computed tomography and magnetic resonance imaging, in Textbook of Geriatric Neuropsychiatry, 2nd edition, edited by Coffey CE, Cummings JL. Washington, DC, American Psychiatric Press, 2000, pp 181-238Google Scholar
31 Guttmann CRG, Jolesz FA, Kikinis R, et al: White matter changes with normal aging. Neurology 1998; 50:972-978Crossref, Medline, Google Scholar
32 Liao D, Cooper L, Cai J, et al: The prevalence and severity of white matter lesions, their relationship with age, ethnicity, gender, and cardiovascular risk factors: the ARIC study. Neuroepidemiology 1997; 16:149-162Crossref, Medline, Google Scholar
33 Yue NC, Arnold AM, Longstreth WT, et al: Sulcal, ventricular, and white matter changes at MR imaging in the aging brain: data from the cardiovascular health study. Radiology 1997; 2:33-39Crossref, Google Scholar
34 Coffey CE, Wilkinson WE, Parashos IA, et al: Quantitative cerebral anatomy of the aging human brain: a cross-sectional study using magnetic resonance imaging. Neurology 1992; 42:527-536Crossref, Medline, Google Scholar
35 Roob G, Schmidt R, Kapeller P, et al: MRI evidence of past cerebral microbleeds in a healthy elderly population. Neurology 1999; 52:991-994Crossref, Medline, Google Scholar
36 Carmelli D, Swan GE, Reed T, et al: Midlife cardiovascular risk factors and brain morphology in identical older male twins. Neurology 1999; 52:1119-1124Google Scholar
37 Schmidt R, Fazekas F, Kapeller P, et al: MRI white matter hyperintensities: three-year follow-up of the Austrian Stroke Prevention Study. Neurology 1999; 53:132-139Crossref, Medline, Google Scholar
38 Veldink JH, Scheltens P, Jonker C, et al: Progression of cerebral white matter hyperintensities on MRI is related to diastolic blood pressure. Neurology 1998; 51:319-320Crossref, Medline, Google Scholar
39 Wahlund LO, Almkvist O, Basun H, et al: MRI in successful aging: a 5-year follow-up study from the eighth to ninth decade of life. Magn Reson Imaging 1996; 14:601-608Crossref, Medline, Google Scholar
40 Holschneider DP, Leuchter AF: Quantitative electroencephalography: neurophysiological alterations in normal aging and geriatric neuropsychiatric disorders, in Textbook of Geriatric Neuropsychiatry, 2nd edition, edited by Coffey CE, Cummings JL. Washington, DC, American Psychiatric Press, 2000, pp 285-310Google Scholar
41 Yamashita K, Kobayashi S, Fukuda H, et al: Leukoaraiosis and event-related potentials (P300) in normal aged subjects. Gerontology 1992; 38:233-240Crossref, Medline, Google Scholar
42 DeCarli C, Murphy DG, Tranh M, et al: The effect of white matter hyperintensity on brain structure, cognitive performance, and cerebral metabolism of glucose in 51 healthy adults. Neurology 1995; 45:2077-2084Google Scholar
43 Fazekas F: Magnetic resonance signal abnormalities in asymptomatic individuals: their incidence and functional correlates. Eur Neurol 1989; 29:164-168Crossref, Medline, Google Scholar
44 Kobari M, Meyer JS, Ichijo M: Leukoaraiosis, cerebral atrophy, and cerebral perfusion in normal aging. Arch Neurol 1990; 47:161-165Crossref, Medline, Google Scholar
45 George MS, McConnell KA, Lorberbaum JP, et al: Functional brain imaging: functional magnetic resonance imaging and magnetic resonance spectroscopy, in Textbook of Geriatric Neuropsychiatry, 2nd edition, edited by Coffey CE, Cummings JL. Washington, DC, American Psychiatric Press, 2000, pp 267-284Google Scholar
46 Sappey-Marinier D, Deicken FR, Fein G, et al: Alterations in brain phosphorous metabolite concentrations associated with areas of high signal intensity in white matter at MR imaging. Radiology 1992; 183:247-256Crossref, Medline, Google Scholar
47 Sappey-Marinier D, Calabrese G, Hetherington HP, et al: Proton magnetic resonance spectroscopy of human brain: applications to normal white matter, chronic infarction, and MRI white matter signal hyperintensities. Magn Reson Med 1992; 26:313-327Crossref, Medline, Google Scholar
48 Inzitari D, Cadelo M, Marranci ML, et al: Vascular deaths in elderly neurological patients with leukoaraiosis. J Neurol Neurosurg Psychiatry 1997; 62:177-181Crossref, Medline, Google Scholar
49 Inzitari D, Giordano GP, Ancona AL et al: Leukoaraiosis, intracerebral hemorrhage, and arterial hypertension. Stroke 1990; 21:1419-1423Google Scholar
50 The Stroke Prevention in Reversible Ischemia Trial (SPIRIT) Study Group: A randomized trial of anticoagulants versus aspirin after cerebral ischemia of presumed arterial origin. Ann Neurol 1997; 42:857-865Crossref, Medline, Google Scholar
51 Balon RW, Yue Q, Socotch TM, et al: White matter lesions and disequilibrium in older people, I: case-control comparison. Arch Neurol 1995; 52:970-974Crossref, Medline, Google Scholar
52 Kinkel WR, Jacobs L, Polachini I, et al: Subcortical arteriosclerotic encephalopathy (Binswanger's disease). Arch Neurol 1985; 42:951-959Crossref, Medline, Google Scholar
53 Masdeu JC, Wolfson L, Lantos G, et al: Brain white-matter changes in the elderly prone to falling. Arch Neurol 1989; 46:1292-1296Google Scholar
54 Camicioli R, Moore MM, Sexton G, et al: Age-related brain changes associated with motor function in healthy older people. J Am Geriatr Soc 1999; 47:330-334Crossref, Medline, Google Scholar
55 Guttman CRG, Benson R, Warfield SK, et al: White matter abnormalities in mobility-impaired older persons. Neurology 2000; 54:1277-1283Google Scholar
56 Tell GS, Lefkowitz DS, Diehr P, et al: Relationship between balance and abnormalities in cerebral magnetic resonance imaging in older adults. Arch Neurol 1998; 55:73-79Crossref, Medline, Google Scholar
57 Austrom MG, Thompson RF, Hendrie HC, et al: Foci of increased T2 signal intensity in MR images of healthy elderly subjects: a follow-up study. J Am Geriatr Soc 1990; 38:1133-1138Google Scholar
58 Harrell LE, Duvall E, Folks DG, et al: The relationship of high-intensity signals on magnetic resonance images to cognitive and psychiatric state in Alzheimer's disease. Arch Neurol 1991; 48:1136-1140Google Scholar
59 Hendrie HC, Farlow MR, Austrom MG, et al: Foci of increased T2 signal intensity on brain MR scans of healthy elderly subjects. AJNR Am J Neuroradiol 1989; 10:703-707Medline, Google Scholar
60 Mirsen TR, Lee DH, Wong CJ, et al: Clinical correlates of white-matter changes on magnetic resonance imaging scans of the brain. Arch Neurol 1991; 48:1015-1021Google Scholar
61 Steingart A, Hachinski VC, Lau C, et al: Cognitive and neurologic findings in subjects with diffuse white matter lucencies on computed tomography scan (leuko-araiosis). Arch Neurol 1987; 44:32-35Crossref, Medline, Google Scholar
62 Matsubayashi K, Shimada K, Kawamoto A, et al: Incidental brain lesions on magnetic resonance imaging and neurobehavioral functions in the apparently healthy elderly. Stroke 1992; 23:175-180Crossref, Medline, Google Scholar
63 Swan GE, DeCarli C, Miller BL, et al: Biobehavioral characteristics of nondemented older adults with subclinical brain atrophy. Neurology 2000; 54:2108-2114Google Scholar
64 Boone KB, Miller BL, Lesser IM, et al: Neuropsychological correlates of white-matter lesions in healthy elderly subjects. Arch Neurol 1992; 49:549-554Crossref, Medline, Google Scholar
65 DeGroot JC, de Leeuw FE, Oudkerk M, at al: Cerebral white matter lesions and cognitive function: the Rotterdam scan study. Ann Neurol 2000; 47:145-151Crossref, Medline, Google Scholar
66 Rao SM, Mittenberg W, Bernardin L, et al: Neuropsychological test findings in subjects with leukoaraiosis. Arch Neurol 1989; 46:40-44Crossref, Medline, Google Scholar
67 Schmidt R, Fazekas F, Offenbacher H, et al: Neuropsychological correlates of MRI white matter hyperintensities: a study of 150 normal volunteers. Neurology 1993; 43:2490-2494Google Scholar
68 No reference.Google Scholar
69 Ylikoski R, Ylikoski A, Erkinjuntti T, et al: White matter changes in healthy elderly persons correlate with attention and speed of mental processing. Arch Neurol 1993; 50:818-824Crossref, Medline, Google Scholar
70 Garde E, Mortensen EL, Krabbe K, et al: Relation between age-related decline in intelligence and cerebral white-matter hyperintensities in healthy octogenarians: a longitudinal study. Lancet 2000; 356:628-634Crossref, Medline, Google Scholar
71 Tupler LA, Coffey CE, Logue PE, et al: Neuropsychological importance of subcortical white matter hyperintensity. Arch Neurol 1992; 49:1248-1252Google Scholar
72 Benton AL, Hamsher KS, Varney NR, et al: Contributions to Neuropsychological Assessment: A Clinical Manual. New York, Oxford University Press, 1983Google Scholar
73 Wechsler D: Wechsler Adult Intelligence Scale-Revised Manual. New York, Psychological Corporation, 1981Google Scholar
74 Almkvist O, Wahlund LO, Andersson-Lundman G, et al: White-matter hyperintensity and neuropsychological functions in dementia and healthy aging. Arch Neurol 1992; 49:626-632Crossref, Medline, Google Scholar
75 Aharon-Peretz J, Cummings JL, Hill MA: Vascular dementia and dementia of the Alzheimer type: cognition, ventricular size, and leukoariosis. Arch Neurol 1988; 45:719-721Crossref, Medline, Google Scholar
76 Erkinjuntti T, Ketonen L, Sulkava R, et al: Do white matter changes on MRI and CT differentiate vascular dementia from Alzheimer's disease? J Neurol Neurosurg Psychiatry 1987; 50:37-42Crossref, Medline, Google Scholar
77 London E, deLeon MJ, George AE, et al: Periventricular lucencies in the CT scans of aged and demented patients. Biol Psychiatry 1986; 21:960-962Crossref, Medline, Google Scholar
78 Radue EW, DeBoulay GH, Harrison MJG, et al: Comparison of angiographic and CT findings between patients with multi-infarct dementia and those with dementia due to primary neuronal degeneration. Neuroradiology 1978; 16:113-115Crossref, Medline, Google Scholar
79 Glatt SL, Lantos G, Danziger A, et al: Efficacy of CT in the diagnoses of vascular dementia. Am J Neuroradiology 1983; 4:703-705Medline, Google Scholar
80 George AE, deLeon MJ, Kalnin A, et al: Leukoencephalopathy in normal and pathologic aging, II: MRI of brain lucencies. Am J Neuroradiol 1986; 7:567-570Medline, Google Scholar
81 Fazekas F, Chawluk JB, Alavi A, et al: MR signal abnormalities at 1.5T in Alzheimer's dementia and normal aging. Am J Neuroradiol 1987; 8:421-426Google Scholar
82 Hershey LA, Modic MT, Greenough PG, et al: Magnetic resonance imaging in vascular dementia. Neurology 1987; 37:29-36Crossref, Medline, Google Scholar
83 Rezek DL, Morris JC, Fulling KH, et al: Periventricular white matter lucencies in senile dementia of the Alzheimer type and in normal aging. Neurology 1987; 37:1365-1368Google Scholar
84 Waldemar G, Christiansen P, Larsson HBW, et al: White matter magnetic resonance hyperintensities in dementia of the Alzheimer type: morphological and regional cerebral blood flow correlates. J Neurol Neurosurg Psychiatry 1994; 57:1458-1465Google Scholar
85 Fazekas F, Kapeller P, Schmidt R, et al: The relation of cerebral magnetic resonance signal hyperintensities to Alzheimer's disease. J Neurol Sci 1996; 142:121-125Crossref, Medline, Google Scholar
86 McDonald WM, Ranga K, Krishnan R, et al: Magnetic resonance findings in patients with early onset Alzheimer's disease. Biol Psychiatry 1991; 29:799-810Crossref, Medline, Google Scholar
87 Fazekas F, Alavi A, Chawluk JB, et al: Comparison of CT, MRI, and PET in Alzheimer's dementia and normal aging. J Nucl Med 1989; 30:1607-1615Google Scholar
88 Kumar A, Yousem D, Souder E, et al: High-intensity signals in Alzheimer's disease without cerebrovascular risk factors: a magnetic resonance imaging evaluation. Am J Psychiatry 1992; 149:248-250Crossref, Medline, Google Scholar
89 Leys D, Soetaert G, Petit H, et al: Perivantricular and white matter hyperintensities do not differ between Alzheimer's disease and normal aging. Arch Neurol 1990; 47:524-527Crossref, Medline, Google Scholar
90 Erkinjuntti T, Gao F, Lee DH, et al: Lack of differentiation in brain hyperintensities between patients with early Alzheimer's disease and control subjects. Arch Neurol 1994; 51:260-268Crossref, Medline, Google Scholar
91 Kozachuk WE, DeCarli C, Shapiro MB, et al: White matter hyperintensities in dementia of the Alzheimer's type and in healthy subjects without cerebrovascular risk factors. Arch Neurol 1990; 47:1306-1310Google Scholar
92 Bowen B, Barker W, Loewenstein D, et al: MR signal abnormalities in memory disorder and dementia. Am J Neuroradiol 1990; 11:283-290Medline, Google Scholar
93 Scheltens P, Barkhof F, Valk J, et al: White matter lesions on magnetic resonance imaging in clinically diagnosed Alzheimer's disease. Brain 1992; 115:735-748Crossref, Medline, Google Scholar
94 Barber R, Scheltens P, Gholkar A, et al: White matter lesions on magnetic resonance imaging in dementia with Lewy bodies, Alzheimer's disease, vascular dementia, and normal aging. J Neurol Neurosurg Psychiatry 1999; 67:66-72Crossref, Medline, Google Scholar
95 Barber R, Gholkar A, Scheltens P, et al: Apolipoprotein E ε4 allele, temporal lobe atrophy, and white matter lesions in late life dementia. Arch Neurol 1999; 56:961-965Crossref, Medline, Google Scholar
96 Skoog I, Hesse C, Aevarsson O, et al: A population study of apo E genotype at the age of 85: relation to dementia, cerebrovascular disease, and mortality. J Neurol Neurosurg Psychiatry 1998; 64:37-43Crossref, Medline, Google Scholar
97 Marder K, Richards M, Bello J, et al: Clinical correlates of Alzheimer's disease with and without silent radiographic abnormalities. Arch Neurol 1995; 52:146-151Crossref, Medline, Google Scholar
98 Coffey CE, Figiel GS: Neuropsychiatric significance of SH, in Psychopathology and the Brain, edited by Carroll BJ, Barrett JE. New York, Raven, 1991, pp 243-264Google Scholar
99 Soares JC, Mann JJ: The anatomy of mood disorders - review of structural neuroimaging studies. Biol Psychiatry 1997; 41:86-106Crossref, Medline, Google Scholar
100 Starkstein S, Sabe L, Vasquez S, et al: Neuropsychological, psychiatric, and cerebral perfusion correlates of leukoariosis in Alzheimer's disease. J Neurol Neurosurg Psychiatry 1997; 63:66-73Crossref, Medline, Google Scholar
101 Bondareff W, Raval J, Woo B, et al: Magnetic resonance imaging and severity of dementia in older adults. Arch Gen Psychiatry 1990; 47:47-51Crossref, Medline, Google Scholar
102 Bondareff W, Raval J, Colletti P, et al: Quantitative magnetic resonance imaging (MRI) and the severity of dementia in Alzheimer's disease. Am J Psychiatry 1988; 145:853-858Crossref, Medline, Google Scholar
103 Diaz J, Merskey H, Hachinski V, et al: Improved recognition of leukoariosis and cognitive impairment in Alzheimer's disease. Arch Neurol 1991; 48:1022-1025Google Scholar
104 Snowdon DA, Greiner LH, Mortimer JA, et al: Brain infarction and the clinical expression of Alzheimer disease: the Nun Study. JAMA 1997; 277:813-817Crossref, Medline, Google Scholar
105 Gupta S, Naheedy M, Young J, et al: Periventricular white matter changes and dementia: clinical, neuropsychological, radiological, and pathological correlation. Arch Neurol 1988; 45:637-641Crossref, Medline, Google Scholar
106 Keretsz A, Polk M, Carr T: Cognition and white matter changes on magnetic resonance imaging in dementia. Arch Neurol 1990; 47:387-391Crossref, Medline, Google Scholar
107 Albert M, Naeses MA, Levine HL, et al: Ventricular size in patients with presenile dementia of the Alzheimer type. Arch Neurol 1984; 41:1258-1263Google Scholar
108 Drayer BP, Heyman A, Wilkinson W, et al: Early onset Alzheimer's disease: an analysis of CT findings. Ann Neurol 1985; 17:407-410Crossref, Medline, Google Scholar
109 Loeb C, Gandolfo C, Bino G: Intellectual impairment and cerebral lesions in multiple cerebral infarcts. Stroke 1988; 19:560-565Crossref, Medline, Google Scholar
110 Salat D, Kaye J, Janowsky J: Prefrontal gray and white matter volumes in healthy aging and Alzheimer's disease. Arch Neurol 1999; 56:338-344Crossref, Medline, Google Scholar
111 Ott BR, Faberman RS, Rogg JM, et al: A SPECT imaging study of MRI white matter hyperintensity in patients with degenerative dementia. Dement Geriatr Cogn Disord 1997; 8:348-354Crossref, Medline, Google Scholar
112 Yamaji S, Ishii K, Sasaki M, et al: Changes in cerebral blood flow and oxygen metabolism related to magnetic resonance imaging white matter hyperintensities in Alzheimer's disease. J Nucl Med 1997; 38:1471-1474Google Scholar
113 Fazekas F, Niederkorn K, Schmidt R, et al: White matter signal abnormalities in normal individuals: correlation with carotid ultrasonography, cerebral blood flow measurements, and cerebrovascular risk factors. Stroke 1988; 19:1285-1288Google Scholar
114 Hirono N, Kitagaki H, Kazui H, et al: Impact of white matter changes on clinical manifestation of Alzheimer's disease: a quantitative study. Stroke 2000; 31:2182-2188Google Scholar
115 Lopez O, Becker J, Rezek D, et al: Neuropsychiatric correlates of cerebral white matter radiolucencies in probable Alzheimer's disease. Arch Neurol 1992; 49:828-834Crossref, Medline, Google Scholar
116 Figiel GS, Coffey CE, Weiner RD: Brain magnetic resonance imaging in elderly depressed patients receiving electroconvulsive therapy. Convulsive Therapy 1989; 5:26-34Medline, Google Scholar
117 Dupont RM, Jernigan TL, Gillin JC, et al: Subcortical signal hyperintensities in bipolar patients detected by MRI (letter). Psychiatry Res 1987; 21:357-358Crossref, Medline, Google Scholar
118 Coffey CE, Figiel GS, Djang WT, et al: Subcortical hyperintensity on magnetic resonance imaging: a comparison of normal and depressed elderly subjects. Am J Psychiatry 1990; 147:187-189Crossref, Medline, Google Scholar
119 Zubenko GS, Sullivan P, Nelson JP, et al: Brain imaging abnormalities in mental disorders of late life. Arch Neurol 1990; 47:1107-1111Google Scholar
120 Rabins PV, Pearlson GD, Aylward E, et al: Cortical magnetic resonance imaging changes in elderly inpatients with major depression. Am J Psychiatry 1991; 148:617-620Crossref, Medline, Google Scholar
121 Guze BH, Szuba MP: Leukoencephalopathy and major depression: a preliminary report. Psychiatry Research: Neuroimaging 1992; 45:169-175Crossref, Medline, Google Scholar
122 Coffey CE, Wilkinson WE, Weiner RD, et al: Quantitative cerebral anatomy in depression: a controlled magnetic resonance imaging study. Arch Gen Psychiatry 1993; 50:7-16Crossref, Medline, Google Scholar
123 Howard RJ, Beats B, Forstl H, et al: White matter changes in late onset depression: a magnetic resonance imaging study. Int J Geriatr Psychiatry 1993; 8:183-185Crossref, Google Scholar
124 Krishnan KRR: Neuroanatomical substrates of depression in the elderly. J Geriatr Psychiatry Neurol 1993; 6:39-51Crossref, Medline, Google Scholar
125 Greenwald BS, Kramer-Ginsberg E, Krishnan KRR, et al: MRI signal hyperintensities in geriatric depression. Am J Psychiatry 1996; 153:1212-1215Google Scholar
126 Iadaka T, Nakajima T, Kawamoto K, et al: Signal hyperintensities on brain magnetic resonance imaging in elderly depressed patients. Eur Neurol 1996; 36:293-299Crossref, Medline, Google Scholar
127 Lesser IM, Miller BL, Boone KB, et al: Brain injury and cognitive function in late-onset psychotic depression. J Neuropsychiatry Clin Neurosci 1991; 3:33-40Link, Google Scholar
128 Miller BL, Lesser IM, Boone K, et al: Brain white-matter lesions and psychosis. Br J Psychiatry 1989; 155:73-78Crossref, Medline, Google Scholar
129 O'Brien J, Desmond P, Ames A, et al: A magnetic resonance imaging study of white matter lesions in depression and Alzheimer's disease. Br J Psychiatry 1996; 168:477-485Crossref, Medline, Google Scholar
130 Lenze E, Cross S, McKeel D, et al: White matter hyperintensities and gray matter lesions in physically healthy depressed subjects. Am J Psychiatry 1999; 156:1602-1607Google Scholar
131 Steffens DC, Helms MJ, Krishnan KRR, et al: Cerebrovascular disease and depression symptoms in the Cardiovascular health study. Stroke 1999; 30:2159-2166Google Scholar
132 Kumar A, Bilker W, Jin A, et al: Age of onset of depression and quantitative neuroanatomic measures: absence of specific correlates. Psychiatry Res 1999; 91:101-110Crossref, Medline, Google Scholar
133 Kumar A, Bilker W, Jin A, et al: Atrophy and high intensity lesions: complementary neurobiological mechanisms in late-life major depression. Neuropsychopharmacology 2000; 22:264-274Crossref, Medline, Google Scholar
134 Dupont RM, Jernigan TL, Butters N: Subcortical abnormalities detected in bipolar affective disorder using magnetic resonance imaging: clinical and neuropsychological significance. Arch Gen Psychiatry 1990; 47:55-59Crossref, Medline, Google Scholar
135 Swayze VW, Andreason NC, Alliger RJ, et al: Structural brain abnormalities in bipolar affective disorder. Arch Gen Psychiatry 1990; 47:1054-1059Google Scholar
136 Brown FW, Lewine RJ, Hudgins PA, et al: White matter hyperintensity signals in psychiatric and nonpsychiatric subjects. Am J Psychiatry 1992; 149:620-625Crossref, Medline, Google Scholar
137 Aylward EH, Roberts-Twillie JV, Barta PE, et al: Basal ganglia volumes and white matter hyperintensities in patients with bipolar disorder. Am J Psychiatry 1994; 151:687-693Crossref, Medline, Google Scholar
138 Altshuler LL, Curran JG, Hauser P, et al: T2 hyperintensities in bipolar disorder: magnetic resonance imaging comparison and literature meta-analysis. Am J Psychiatry 1995; 152:1139-1144Google Scholar
139 Botteron KN, Vannier MW, Geller B, et al: Preliminary study of magnetic resonance imaging characteristics in 8- to 16-year-olds with mania. J Am Acad Child Adolesc Psychiatry 1995; 34:742-749Crossref, Medline, Google Scholar
140 Dupont RM, Jernigan TL, Heindel W, et al: Magnetic resonance imaging and mood disorders: localization of white matter and other subcortical abnormalities. Arch Gen Psychiatry 1995; 52:747-755Crossref, Medline, Google Scholar
141 Woods BT, Brennan S, Yurgelun-Todd D, et al: MR imaging abnormalities in major psychiatric disorders: an exploratory comparative study. J Neuropsychiatry Clin Neurosci 1995; 7:49-53Link, Google Scholar
142 Strakowski SM, Woods BT, Tohen M, et al: MR imaging subcortical signal hyperintensities in mania at first hospitalization. Biol Psychiatry 1993; 33:204-206Crossref, Medline, Google Scholar
143 Woods BT, Yurgelun-Todd D, Mikulis D, et al: Age-related MRI abnormalities in bipolar illness: a clinical study. Biol Psychiatry 1995; 38:846-847Crossref, Medline, Google Scholar
144 McDonald WM, Tupler LA, Marsteller FA, et al: Hyperintense lesions on magnetic resonance images in bipolar disorder. Biol Psychiatry 1999; 45:965-971Crossref, Medline, Google Scholar
145 Figiel GS, Krishnan KRR, Rao VP, et al: Subcortical hyperintensities on brain magnetic resonance imaging: a comparison of normal and bipolar subjects. J Neuropsychiatry Clin Neurosci 1991; 3:18-22Link, Google Scholar
146 McDonald WM, Krishnan KRR, Doraiswamy PM, et al: Occurrence of subcortical hyperintensities in elderly subjects with mania. Psychiatry Res: Neuroimaging 1991; 40:211-220Crossref, Medline, Google Scholar
147 Krabbendam L, Honig A, Wiersma J, et al: Cognitive dysfunctions and white matter lesions in patients with bipolar disorder in remission. Acta Psychiatr Scand 2000; 101:274-280Medline, Google Scholar
148 Videbech P: MRI findings in patients with affective disorder: a meta-analysis. Acta Psychiatr Scand 1997; 96:157-168Crossref, Medline, Google Scholar
149 Ballard C, McKeith I, O'Brien J, et al: Neuropathological substrates of dementia and depression in vascular dementia, with a particular focus on cases with small infarct volumes. Dement Geriatr Cogn Disord 2000; 11:59-65Crossref, Medline, Google Scholar
150 Coffey CE, Weiner RD, Djang WT, et al: Brain anatomic effects of electroconvulsive therapy: a prospective magnetic resonance imaging study. Arch Gen Psychiatry 1991; 48:1013-1021Google Scholar
151 Kalin NH, Davidson RJ, Irwin W, et al: Functional magnetic resonance imaging studies of emotional processing in normal and depressed patients: effects of venlafaxine. J Clin Psychiatry 1997; 58(suppl 16):32-29Google Scholar
152 Kato T, Inubushi T, Kato N: Magnetic resonance spectroscopy in affective disorders. J Neuropsychiatry Clin Neurosci 1998; 10:133-147Link, Google Scholar
153 Ebmeier K, Glabus M, Prentice N, et al: A voxel-based analysis of cerebral perfusion in dementia and depression of old age. Neuroimage 1998; 7:199-208Crossref, Medline, Google Scholar
154 Erwin CW, Coffey CE, Marsh GR: Polysomnographic findings in elderly depressed patients with unsuspected MRI abnormalities of the pons (abstract). Sleep Res 1989; 18:174Google Scholar
155 Hickie I, Scott E, Mitchell P, et al: Subcortical hyperintensities on magnetic resonance imaging: clinical correlates and prognostic significance in patients with severe depression. Biol Psychiatry 1995; 37:151-160Crossref, Medline, Google Scholar
156 Lesser IM, Boone KB, Mehringer CM, et al: Cognition and white matter hyperintensities in older depressed patients. Am J Psychiatry 1996; 153:1280-1287Google Scholar
157 Churchill CM, Priolo CV, Nemeroff CB, et al: Occult subcortical magnetic resonance findings in elderly depressives. Int J Geriatr Psychiatry 1991; 6:213-216Crossref, Google Scholar
158 Dahabra S, Ashton CH, Bahrainian M, et al: Structural and functional abnormalities in elderly patients clinically recovered from early and late-onset depression. Biol Psychiatry 1998; 44:34-46Crossref, Medline, Google Scholar
159 Figiel GS, Krishnan KRR, Doraiswamy PM, et al: Subcortical hyperintensities on brain magnetic resonance imaging: a comparison between late age onset and early onset elderly depressed subjects. Neurobiol Aging 1991; 26:245-247Crossref, Google Scholar
160 Krishnan KRR, McDonald WM, Doraiswamy PM, et al: Neuroanatomical substrates of depression in the elderly. Eur Arch Psychiatry Clin Neurosci 1993; 243:41-46Crossref, Medline, Google Scholar
161 Salloway S, Malloy P, Kohn R, et al: MRI and neuropsychological differences in early and late-life-onset geriatric depression. Neurology 1996; 46:1567-1574Google Scholar
162 Krishnan KRR, Hays JC, Blazer DG: MRI-defined vascular depression. Am J Psychiatry 1997; 154:497-501Crossref, Medline, Google Scholar
163 Kramer-Ginsberg E, Greenwald BS, Krishnan KRR, et al: Neuropsychological functioning and MRI signal hyperintensities in geriatric depression. Am J Psychiatry 1999; 156:438-444Medline, Google Scholar
164 Figiel GS, Krishnan KRR, Breitner JC, et al: Radiologic correlates of antidepressant-induced delirium: the possible significance of basal-ganglia lesions. J Neuropsychiatry Clin Neurosci 1989; 1:188-190Link, Google Scholar
165 Figiel GS, Krishnan KRR, Doraiswamy PM, et al: Caudate hyperintensities in elderly depressed patients with neuroleptic-induced parkinsonism. J Geriatr Psychiatry Neurol 1991; 4:86-89Crossref, Medline, Google Scholar
166 Fujikawa T, Yokota N, Muraoka M, et al: Response of patients with major depression and silent cerebral infarction to antidepressant drug therapy, with emphasis on central nervous system adverse reactions. Stroke 1996; 27:2040-2042Google Scholar
167 Hickie I, Scott E, Wilhelm K, et al: Subcortical hyperintensities on magnetic resonance imaging in patients with severe depression: a longitudinal evaluation. Biol Psychiatry 1997; 42:367-374Crossref, Medline, Google Scholar
168 Alexopoulos GS, Meyers BS, Young RC, et al: Clinically defined vascular depression. Am J Psychiatry 1997; 154:562-565Crossref, Medline, Google Scholar
169 Steffens DC, Krishnan KRR: Structural neuroimaging and mood disorders: recent findings, implications for classification, and future directions. Biol Psychiatry 1998; 43:705-712Crossref, Medline, Google Scholar
170 Duffy, JD, Coffey CE: The neurobiology of depression, in Contemporary Behavioral Neurology, edited by Trimble MR, Cummings JL. Boston, Butterworth-Heinemann, 1997, pp 275-288Google Scholar
171 Mayberg HS: Limbic-cortical dysregulation: a proposed model of depression. J Neuropsychiatry Clin Neurosci 1997; 9:471-481Link, Google Scholar
172 Husain MM, Knight DL, Doraiswamy PM, et al: Platelet [3H]-imipramine binding and leukoencephalopathy in geriatric depression. Biol Psychiatry 1991; 29:665-670Crossref, Medline, Google Scholar
173 Musselman DL, Evans DL, Nemeroff CB: The relationship of depression to cardiovascular disease. Arch Gen Psychiatry 1998; 55:580-592Crossref, Medline, Google Scholar
174 Markovitz JH, Shuster JL, Chitwood WS, et al: Platelet activation in depression and effects of sertraline treatment: an open-label study. Am J Psychiatry 2000; 157:1006-1008Google Scholar
175 Jonas BS, Mussolino ME: Symptoms of depression as a prospective risk factor for stroke. Psychosom Med 2000; 62:463-471Crossref, Medline, Google Scholar
176 Everson SA, Roberts RE, Goldberg DE, et al: Depressive symptoms and increased risk of stroke mortality over a 29-year period. Arch Intern Med. 1998; 158:1133-1138Google Scholar
177 Simonsick EM, Wallace RB, Blazer DG, et al: Depressive symptomology and hypertension-associated morbidity and mortality in older adults. Psychosom Med 1995; 57:427-435Crossref, Medline, Google Scholar
178 Kelsoe TR, Cadet JL, Pickar D, et al: Quantitative neuroanatomy in schizophrenia. Arch Gen Psychiatry 1988; 45:533-541Crossref, Medline, Google Scholar
179 Jeste DV, Cullum M, Jernigan T, et al: Late-onset schizophrenia: neuropsychology and MRI. Presented at the American Psychiatric Association Annual Meeting, Montreal, Canada, May 10, 1988Google Scholar
180 Sachdev P, Brodaty H: Quantitative study of signal hyperintensities on T2-weighted magnetic resonance imaging in late-onset schizophrenia. Am J Psychiatry 1999; 156:1958-1967Google Scholar
181 Lesser IM, Miller BL, Boone KB, et al: Neuro-imaging and late life psychosis. Presented at the American Psychiatric Association Annual Meeting, Montreal, Canada, May 10, 1988Google Scholar
182 Skoog I, Berg S, Johansson B, et al: The influence of white matter lesions on neuropsychological functioning in demented and non-demented 85-year-olds. Acta Neurol Scand 1996; 96:142-148Google Scholar
183 Brant-Zawadzki M, Fein G, Van Dyke C, et al: MR imaging of the aging brain: patchy white-matter lesions and dementia. Am J Neuroradiol 1985; 6:675-682Medline, Google Scholar
184 Hunt AL, Orrison WW, Yeo RA, et al: Clinical significance of MRI white matter lesions in the elderly. Neurology 1989; 39:1470-1474Google Scholar
185 Schmidt R, Fazekas F, Offenbacher H, et al: Magnetic resonance imaging white matter lesions and cognitive impairment in hypertensive individuals. Arch Neurol 1991; 48:417-420Crossref, Medline, Google Scholar
186 Lewine R, Hudgins P, Risch SC, et al: Lowered attention capacity in young, medically healthy men with magnetic resonance brain hyperintensity signals. Neuropsychiatry Neuropsychol Behav Neurol 1993; 6:38-42Google Scholar
187 O'Brien JT, Desmond P, Ames D, et al: Magnetic resonance imaging correlates of memory impairment in the healthy elderly: association with medial temporal lobe atrophy but not white matter lesions. Int J Geriatr Psychiatry 1997; 12:369-374Crossref, Medline, Google Scholar
188 DeGroot JC, de Leeuw F, Oudkerk M, et al: Cerebral white matter lesions and depressive symptoms in elderly adults. Arch Gen Psychiatry 2000; 57:1071-1076Google Scholar
189 Schmidt R: Comparison of magnetic resonance imaging in Alzheimer's disease, vascular dementia and normal aging. Eur Neurol 1992; 32:164-169Crossref, Medline, Google Scholar