Toward an Understanding of Decision Making in Severe Mental Illness
Abstract
A commonality of patients with major psychiatric disorders is their propensity to make poor decisions, which is intimately related to poor real-life outcomes. The authors reviewed the literature on decision making as applied to severe psychiatric disorders, with particular focus on advances in cognitive neuroscience. Deficits in reward sensitivity, avoidance learning, and temporal discounting are reported in depression. Besides abnormalities in hedonic capacity, other cognitive distortions required for flexible control of behavior occur in patients with bipolar disorder and schizophrenia. A conceptual framework of abnormal decision making in mental illness could generate targeted interventions to improve quality of life and clinical outcomes.
In this paper, a theoretical reconceptualization of some of the critical characteristics of severe mental illness is presented as an extension of previous conceptualizations that have focused on cognitive factors as central components of psychiatric disorders.1 Psychiatric disorders are defined by abnormalities of thought, affect, and impulse control. Arguably, the greatest functional impact of these illnesses on the lives of the mentally ill and society are not related to the symptoms of delusions, hallucinations, or depressed mood, but simply to making poor decisions. These decisions can contribute to behaviors such as nonadherence to medications or outpatient appointments, failing to exercise, poor diet, using drugs, or engaging in unprotected sex. Downstream consequences of poor decisions include worsening of symptoms, reduced life satisfaction, impaired everyday functioning, relapse and rehospitalization, clashes with law enforcement, poor physical health, and even more tragic outcomes such as accidental death, homicide, or suicide.
In many cases flawed decision making in mental illness is a product of the deficits in basic neuropsychological processes, including impaired attention, working memory, or response inhibition. Flawed decision making has a pervasive presence in clinical outcomes. For example, adherence rates for antidepressants, mood stabilizers, antipsychotics, or stimulants range between 30%−50%. More than half of the patients with severe mental illness are affected by substance use disorders. Forty percent of schizophrenic patients, regardless of medication regimen, suffer from metabolic syndrome. Thus, addressing impaired decision-making processes should provide a valuable strategy to improve everyday functioning through an alteration of health status and treatment outcomes in psychiatric patients. In this monograph, the concepts used to characterize decision making are reviewed followed by a review of the known neural substrates that mediate the various elements of decision making. Last, the application of a decision-making approach to understanding some of the morbidity associated with mental illness is described.
Defining Decision Making
A decision problem is defined by the actions or options among which one must choose, the possible outcomes or consequences of these actions, and the contingencies or conditional probabilities that relate outcomes to actions. Herbert Simon, a Nobel laureate, decomposed the task of rational decision making in three steps: (1) identification and delineation of all alternatives, (2) determination of the consequences of each alternative, and (3) a comparison of the accuracy and efficiency of each of these sets of consequences. Decision making is a constant process in modern life, that takes place from when we wake up until we decide to go to bed. Different disciplines, including psychology, psychiatry, neuroscience, economics, and neuroeconomics attempt to systematize the understanding of decision making.
Decision-making research paradigms range from electrophysiological to behavioral experiments (from insects to humans), lesion studies, and brain imaging. The paradigms used include monetary or nonmonetary positive- and negative-reinforcing stimuli (e.g., food, juice squirts, electric shocks) in order to assess an individual’s reactions and choices. These paradigms allow for the exploration and characterization of interactions between individuals using economic principles (Table 1).
Paradigm | Description | Neuropsychological target |
---|---|---|
Gamble/ lottery | Choice between possibilities associated with different outcomes | Calculation of expected utility and risk |
Risk can be dissociated from impulsivity | ||
Iowa gambling task | Selection of cards of four different stacks with different monetary gains and penalties | |
Cambridge gambling task | Probabilistic choice of finding a hidden token behind 10 boxes | Risk assessment without learning confounds |
Wheel of fortune | Two choice task with probabilistic monetary outcomes | Targets separately reward and punishment processing |
Ultimatum game | Two player game about how to split a pile of money. One makes an offer, and the other accepts or rejects it | Dissociation of profit from fairness expectations |
Trust game | Two player game about sharing one’s profits hoping for retribution from the other | Cooperation, trust, fairness, altruistic behavior |
Prisoner’s dilemma | Two player game about cooperating or defecting in a coordinated strategy | Cooperation, reciprocity, defection |
Stag hunt | Two player game about | Risk assessment, mentalization |
One of the many psychological theories focused on understanding behavior, drive theory, posits that organisms have needs, the primary needs related to survival, such as food and shelter, and secondary needs related to the organism’s well-being and comfort. The goal of satisfaction of these needs drives organisms to action and represents the underlying motivation for behavior. When a need is satisfied, the drive is reduced and the person returns to a state of homeostasis and relaxation. An opposing view is promulgated in Skinnerian models, which deny the existence of inner nonobservable phenomena, including motivation. Skinner defined operant conditioning and decision making as processes in which behavior is solely determined by its consequences, wherein organisms learn through experience to behave in ways in order to obtain rewards and avoid punishment. Thus, decision making is intrinsically related to previous experiences and their consequences.
Cognitive Control
Cognitive control refers to the ability to flexibly direct behavior in accordance with a variety of goals, and constitutes the ability to represent, maintain, and update the rules that guide behavior in a context-appropriate manner. Cognitive control functions include error detection and correction mechanisms, conflict resolution, response inhibition, task-switching, and emotion regulation. Although cognitive control functions were initially deemed to be a frontal cortex function, research in the last two decades has identified a “cognitive control network” that includes the dorsolateral prefrontal cortex (DLPFC), medial prefrontal cortex (mPFC), anterior cingulate cortex (ACC), parietal cortex, motor areas, and cerebellum.2–5 Recent meta analyses of a very large number of published functional magnetic resonance imaging (fMRI) datasets demonstrated that a superordinate frontal-cingulate-parietal-subcortical cognitive control system is engaged during the performance of a range of executive function tasks.6 Functional connectivity has revealed that coordinated temporal activation across the network of prefrontal and posterior brain regions is associated with better performance on cognitive control tasks.7 Impairments in the cognitive control system are seen in a variety of psychiatric disorders. For instance, deficits in emotion regulation are central to borderline personality and mood disorders, attention biases to negative stimuli are hallmark of anxiety disorders. Moreover, global impairments in cognitive control associated with deficits in attention, memory, language comprehension, and emotional processing are characteristic of schizophrenia, and responsible for this disorder’s most disheartening clinical challenges (e.g., disorganization, negative symptoms) and functional outcome.
Reward and Value
A considerable amount of neuroscience research has been performed using the learning paradigm classical conditioning. Classical conditioning, prototypically illustrated by Pavlov’s experiments, refers to the development of an automatic association between two co-occurring, previously independent stimuli. The classic example is a dog’s association of hearing a bell and the presentation of food thereby inducing salivation to subsequent sounding of the bell in the absence of the food stimulus. Seminal studies by Schultz et al. 8 examined brain responses during classical conditioning and showed increased phasic responses in the ventral tegmental area (VTA) dopaminergic neurons in primates when presented with an unexpected pleasurable stimulus or reward. These brain responses were subsequently evoked by a conditioned stimulus after pairings with the reward. Further, the activation of dopamine neurons decreased with extinction trials when the expected reward was omitted. Subsequent observations showed that these neurons actually fire in anticipation of future rewards. Thus, dopaminergic neurons seem to encode the likelihood of a rewarding outcome and generate a continuous update of its prediction accuracy. Dopamine is therefore believed to provide a teaching signal to parts of the brain responsible for acquiring new behaviors. Notably, serotonin closely interacts with dopamine along two different axes: reward (dopamine)-punishment (serotonin) and behavioral activation (dopamine) inhibition (serotonin).9 The role of serotonin seems to counteract impulsivity, possibly by enhancing aversion and increasing behavioral inhibition. Although dopamine promotes behavioral activation to seek rewards, serotonin serves to inhibit actions when punishment may occur.
Neuroimaging experiments have identified dopaminergic projection areas, the orbitofrontal cortex (OFC) and ventral striatum [including the nucleus accumbens (NAcc)], as sites active during the learning, retention, and extinction conditions during reward processing.10 These two structures constitute the brain reward valuation system, which has been posited to mediate representation of a common neural currency.11 Ventral striatal activation correlates with reward magnitude, whereas mPFC activation correlates with the probability of receiving a reward, reward anticipation, and saliency. However, the calculation of the different facets of reward and value are distributed along several brain regions, including the posterior cingulate cortex (PCC), ACC, the temporoparietal junction, including the inferior parietal lobule, superior temporal gyrus and anterior insula, and the left posterior parietal cortex.12
Risk, Uncertainty, and Ambiguity
Expected value, initially defined by Pascal, is the product of the payoff and estimated probability of occurrence of a possible choice, and represents a common internal currency that individuals use to compare and choose between different options to utilize resources (i.e., buying drugs versus groceries and medications).
The value of an item to an individual depends on the risk context. Thus, in an environment where the consequences of choices are certain and constant (Figure 1A, Figure 1B), the option with the highest value would be the preferred one.13 However, since this situation is rarely encountered, expected value alone is often a poor predictor of choice. Risk is present when multiple possible outcomes can occur with well-defined or estimable probabilities (Figure 1C, Table 2). In economic models, risk is considered a cost and has to be weighed against the expected value of a decision. Sensitivity to risk is evidenced as risk aversion or risk-seeking behaviors. The incorporation of risk with reward or payoff led Bernoulli to posit the idea of subjective value or utility. Utility is the subjective assessment of the value of a reward; it is dynamic and can be influenced by the physiologic state of the individual (hunger, thirst, or sleep) and the context (home, inpatient unit, or prison). For instance, the challenge of keeping sobriety and taking prescribed medications is quite different for patients receiving ambulatory or inpatient care.
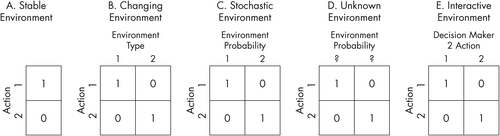
A) Stable environment; B) changing environment; C) stochastic environment; D) unknown environment; and E) interactive environment (Adapted From13)
Concept | Definition | Neural substrate |
---|---|---|
Expected value and expected utility | Calculation of the combination of payoff and probability of occurrence a possible choice | Ventral striatum, medial prefrontal cortex, and orbitofrontal cortex |
Magnitude | Ventral striatum | |
Probability | Medial prefrontal cortex | |
Uncertainty | Lack of knowledge of a decisions’ outcome | Dorsomedial prefrontal cortex and anterior insula |
Risk | Known unpredictable probability of an undesired outcome. | Dorsomedial prefrontal cortex, amygdala, and anterior insula |
Ambiguity | Unknown unpredictable probability of an undesired outcome. | Amygdala and insula |
Temporal discounting | Decrease in the subjective value of a reward as a function of the amount of and delay to receive the reward | Interaction between impulsive (ventral striatum) and executive (dorsolateral prefrontal cortex, posterior parietal cortex) drives. |
Regret | Reaction when a choice that resulted in a worse outcome than would have occurred with an alternative choice | Orbitofrontal cortex, superior frontal cortex, angular gyrus, and thalamus |
Rejoice | Reaction when the option chosen yields a more favorable outcome than an alternative decision | Rostral anterior cingulate, hippocampus, ventral striatum, and midbrain |
Dread | Reaction to waiting for a known future bad outcome | Caudal anterior cingulate and posterior insula |
A more accurate analysis of real life situations requires the consideration of the interaction of uncertainty × context. Uncertainty refers to the lack of knowledge about the outcome of a decision and it can be associated with risk or ambiguity (Table 2). Ambiguity occurs in the presence of multiple outcomes whose probabilities are unknown or not well-defined, hence, the expected value cannot be calculated (Figure 1D). Ambiguity is a more common real world situation and is even more aversion-provoking than risk. In the latter part of the last century, Kahneman and Tversky14 developed prospect theory, which explains how humans calculate value nonlinearly. This view, supported by findings of nonlinear striatum activity, explains phenomena such as larger aversive response to losses compared with favorable response to comparable gains, strong preference for certainty, and cognitive biases as the framing effect (i.e., inconsistent choices dependent on whether a question is presented in terms of gains or losses).14
Risky choice tasks, such as facing a large reward associated with an unlikely outcome, versus a small reward associated with a more probable outcome, lead to OFC activation.15 Additionally, increased insular activation occurs when choosing riskier outcomes over safer outcomes10,16 and amygdalar activation appears in anticipation of loss or punishment.17 Gambling tasks revealed expected risk to be proportional to activation in the insula, lateral OFC, and midbrain,18 and choices that maximized gains or minimized losses were predicted by activation in the ventromedial PFC (VMPFC) or anterior insula, respectively19 Of these regions, the insula and the dorsomedial PFC (DMPFC)20 have been shown to be predictive of uncertainty related to monetary21 and nonmonetary stimuli.22,23 In summary, the data support the view that the cognitive processing of uncertainty is related to the DMPFC, whereas the affective reaction is associated with the OFC and insula. Much of the data presented in this paper originates from fMRI. These data are usually obtained from the comparison of brain activation of a condition of interest and an adequate control, which is reflected in statistical maps that can be visualized as activation of particular brain regions or circuits. However, sensory perception, motor function, and cognition are the product of a coordinated action of several neural processes that occur below the temporal or spatial resolution of fMRI. Thus, it is important to avoid the temptation of assigning discrete mental operations to specific brain regions or circuits. Last, a practical example of abnormalities in uncertainty processing is the anticipatory anxiety found in panic disorder. Panic attacks can be such terrifying and helplessness-inducing experiences that patients live in fear of the next attack, uncertain when it will occur, thereby leading to progressively restricted lifestyles as futile attempts to prevent them.
Strategic Uncertainty
An even more realistic scenario when considering decision making in a social context is interaction with other individuals. Figure 1E shows the interaction between two individuals. In this type of interaction a decision maker needs to consider not only the inherent risk but also what the other person is likely to do, and how his/her own actions will affect others (strategic uncertainty). Strategic uncertainty can be studied with economic games that target the interaction between two or more people such as the prisoners’ dilemma, the stag hunt, or the ultimatum game (Table 1). Paranoia is a prime example of a mental state that strongly influences the view of others and their attributed intentions, and consequently the patient’s own decision making that frequently leads to violence or isolation.
Game Theory
Game theory attempts to predict the strategies that a group of decision makers will converge on as they try to maximize their own profits. It rests on two basic assumptions: first, individuals only seek to maximize their own profit; and second, to achieve this they behave rationally. Nash equilibrium refers to a set of strategies from which no individual player can increase his payoff unilaterally. However, results from experiments in both human and nonhuman primates have systematically violated predictions from the Nash equilibrium. In humans, decision making in social contexts is not purely driven by self-interest, but also by considerations about the well-being of other individuals. Therefore, to better characterize decision making in social contexts, socially oriented emotions such as spite, fairness, and altruism need to be considered. The relevance of decision making within social contexts cannot be overestimated. For example, guilt is frequently found in abusive relationships in depressed patients; grandiosity and recklessness explain many of the interpersonal conflicts during mania; and paranoia and auditory hallucinations found in psychosis can strain interpersonal interactions to the point of violence.
Temporal Discounting
An additional dimension in decision making is time. We do not assign the same subjective value to rewards obtained in the present or in the future. Temporal or delay discounting is an operational measure of delayed gratification and has been shown to be altered in a number of conditions marked by impulsivity. This refers to the decrease in the subjective value of a commodity as the product of its amount and the delay to receive it.24 For instance, if someone would prefer $80 now instead of $100 in 1 year, it could be said that the temporally distant $100 are discounted to an immediate value of $80. In a classical experiment, Mischel and collaborators presented preschool children the choice of one treat (cookie or candy) immediately, or having to wait several minutes for two treats.25 This task was remarkably predictive of the childrens future life achievements. Children who preferred delayed larger rewards tended to be more intelligent, better able to concentrate, and more tolerant to frustration; and as adolescents had higher social-emotional and cognitive function ratings, including academic performance.25 Later follow up showed that the ability to wait as a preschooler predicted higher education achievements, and less risk for crack cocaine use.26 This capacity to delay gratification has been associated with effective self-regulation through the use of abstract rather than consummatory strategies, as well as shifting of attention. Furthermore, in adolescents, a greater ability to delay gratification was associated with such characteristics as being responsible, productive, ethically consistent, interested in intellectual matters, overly controlled, and higher IQ, whereas those adolescents who were not able to delay gratification were characterized as rebellious, unpredictable, self-indulgent, or hostile.27
Temporal discounting is the outcome of opposing trends. The first, an instinctive response of craving immediate fulfillment of desires or needs, is linked to activation of medial OFC and subcortical structures, mainly the ventral striatum (VS).28 The second, executive function, paradigm of cold cognition, reasoning, and planning, is associated with the activity of the right DLPFC and posterior parietal cortex.29 The role of the PFC is further illustrated by the overwhelming preference for immediate rewards in patients with prefrontal damage in the so-called “environmental-dependency syndrome.”30 Activity in the mPFC, ventral striatum, and PCC are directly proportional to a reward magnitude, but inversely to the delay in time in which it is expected.31 An attempt to separate the risk component in temporal discounting showed frontal pole, PCC, and the parahippocampal gyri to be associated with purely temporal computation.32 Furthermore, when risky choices are dissociated from temporal ones, the former are associated with activation in DLPFC and posterior parietal cortex, whereas the later indicated activation of the PCC and the striatum.33 These results suggest that cognitive processing of temporal discounting needs to integrate an element of risk and visualization of the future.
Emotions and Decision Making
A traditional dichotomous view of hot emotion versus cold cognition has been refuted by research in neuroscience, psychology, and economics over the last decades. Emotion does not equate with irrationality, but is an altogether component of the decision-making process. Not surprisingly, the wide range of various emotions explains why is unrealistic to project all emotions into a one-dimensional category of pleasure versus pain.34 Loewenstein and Lerner construe emotions according to their place along the time course of the decision process, including deliberation about a choice and the posterior reaction to the outcome.35 They distinguish between anticipated and immediate emotions, with immediate emotions further classified into incidental and integrated emotions. Anticipated emotions are those believed to occur following a given decision outcome. This is also known as effective forecasting and typical examples are regret or disappointment. On the other hand, immediate emotions are those experienced while the individual is pondering a choice. Immediate emotions are either incidental emotions caused by factors, which are not related to the decision problem at hand, or integral emotions, which are caused by the decision problem itself.
On the other hand, Pfister and Bohm classify emotion according to its function emphasizing emotion’s role in decision-making processes. They consider four categories: information, speed, relevance, and commitment.36 The information function provides evaluative information which weighs in preference construction. Emotions such as joy or distress inform about the degree of (un)pleasantness of choices and consequences. They allow one to map a diversity of experiences on a one-dimensional scale of pleasure and pain. The speed function enables rapid choice and action under time pressure. Affect programs for negative emotions such as fear and disgust trigger immediate avoidance responses.37 These mechanisms are highly stimulus-specific and presumably have evolved under evolutionary selection pressure. The somatic-marker hypothesis38 maintains that these kinds of affective signals, originating in bodily states and acquired by learning from previous experiences, act as markers about the valence of current experiences. Somatic markers operate automatically and obligatorily, influencing behavior even before a conscious intention is generated. The relevance function focuses attention on particular aspects of potential relevance for the decision-maker. Emotions such as regret or envy constitute a particular appraisal, which implies particular evaluations as well as particular action tendencies. The commitment function enables social coordination by committing people to stick to decisions, even against their short-term self-interest. Guilt, altruism, love, or hate guide decision making in strategic choice situations. Overall, emotions do not have a uniform influence on decision making, but seem to be rather dependent on the context and in the individual circumstances. An example of the pervasive effect of emotion in mental illness is given by the abnormalities in reward processing found in anhedonia and depression (Table 3).39
Condition | Decision-making abnormalities | Neural substrate |
---|---|---|
Depression and anhedonia | Deficits in reward processing | Hypoactive ventral and dorsal striatum |
Hyperactive orbitofrontal cortex | ||
Drug addiction | Deficits in temporal discounting | Hyperactivity of mesolimbic system (orbitofrontal and ventral striatum) and/or hypoactive executive system (dorsolateral prefrontal cortex and posterior parietal cortex) |
Exaggerated risk taking behavior | ||
Schizophrenia | Context processing | Hypoactive dorsolateral prefrontal cortex |
Deficits in reward processing | ||
Deficits in temporal discounting | ||
Gambling | Illusion of personal control | Abnormal activity in ventromedial prefrontal cortex, ventral striatum anterior insula |
Near miss effect | ||
Loss chasing | ||
Anorexia nervosa | Abnormal reward processing (quantitatively and qualitatively) | Abnormal activity in hypoactive ventral striatum |
Unconscious Decision Making
Unconscious knowledge refers to that revealed by task performance alone, subjects being unaware that they are accessing it, whereas we speak of conscious knowledge when subjects are aware of possessing and accessing it.40 It ranges from basic perceptual processing to spontaneous problem solving. Even though attention to the unconscious had already been studied before Freud, his work had considerable impact on the 20th century’s research on unconscious knowledge.41 Unconscious or subliminal processing has been described in visual, auditory, somatosensory, and olfactory information. Experimental methods used to tap unconscious processes, include studying subjects who are unaware of the stimuli, because they are too weak, brief, complex, or are masked (i.e., subliminal perception). Other approaches examine states of complete unconsciousness (i.e., sleep, coma, and anesthesia), inability to be conscious of certain kinds of stimuli (i.e., blindsight, hemineglect, and prosopagnosia), or when attention has been diverted to another demanding task.
Examples of cognitive processes found to run at the unconscious level include task-set preparation, conflict detection/resolution, motivation, and error detection. (For detailed reviews see42,43.) Several “high-level” (prefrontal) cognitive functions, such as response inhibition and task-switching, space integration of multiple unconscious stimuli,44 ensemble statistics,45 and play recognition in expert chess players46 have been observed to be influenced and modulated by subliminal stimuli. Priming research has shown that subliminal information can affect behavior and brain activity for a considerable amount of time, even 24 hours.47 Last, although controversial, unconscious thought theory, with limitations and detractors, attempts to account for the existence of what it calls “the unconscious” in the empiric observations of people seeming to make better decisions when they leave it to “the unconscious” to do the job.48
Decision Making in Mental Illness
Psychiatric disorders encompass a wide variety of nosological presentations traditionally classified as disorders of thought, affect, or impulse control. The pathophysiology of the vast majority of psychiatric disorders is complex and not well understood. There is a trend in psychiatry to explain mental illness with common denominators across diagnostic boundaries. One common element in severe mental illness is pervasive bad decision making (i.e., not taking medications, paying heed to command hallucinations, or attempting suicide). Despite the ubiquity of decision making in daily life, it has been largely neglected in the diagnosis and management of mental illness. Individuals’ choices are intimately related to disease outcome (hospitalization, incarceration, or suicide), which have been largely unchanged despite decades of research in neuroscience, psychology, and psychiatry.
Depression
Major depressive disorder (MDD) is characterized by two primary affective symptoms: sustained negative affect and reduced positive affect.49 In general, decisions during depressed states are tainted by negative affect and distorted negative cognitions,50 although some research suggests that mild levels of depression may be associated with more realistic self-assessments even in psychotic patients.51 A number of studies have used monetary and nonmonetary paradigms to evaluate the reward system in depression. A consistent pattern of reduced activation of the ventral striatum, dorsal striatum, and VMPFC52–54 in response to positive reward stimuli has been reported, but see also for discrepant results.55 A real life consequence of altered reward processing in depression was demonstrated in a report of economic social interaction using the ultimatum game in which participants were asked to accept or reject a wide range of offers. Depressed patients exhibited a more negative emotional reaction to unfair offers, despite accepting more of these offers than controls.56 Thus, depression appears to have a dual effect on the processing of reward and value: induction of excessive emotional responses and reduced willingness to reject unfair offers.
There is considerable evidence that monoamine systems, including doparnine (DA) and serotonin, are altered in depression.57 Considerable monoamine loss is observed in high-risk states for depression.58 In particular, DA neurotransmission in major depressive disorder (MDD) seems to be diminished, either by decreased DA release or intracellular signaling processing. These DA-related disturbances improve by treatment with antidepressants, presumably by acting on serotonergic or noradrenergic circuits, which then affect DA function.57 Furthermore, DA receptor binding and amphetamine response in depression are correlated with altered brain activation in the ventrolateral PFC, OFC, caudate, and putamen.59 Overall, depression seems to be associated with an alteration of the prefrontal control over the striatum leading to a dysfunctional frontostriatal connectivity. The impact of the frontostriatal system dysfunction in depression can be further understood considering the role of two circuits: the medial prefrontal cortex-ventral striatum, which underlies motivation, and the OFC-ventromedial caudate, considered to intervene in affective processing.60,61
In addition of serotonin’s role in impulsive aggression,62–64 abnormal serotonin function has been linked to psychopathologies associated with negative affects such as depression and anxiety.65–68 In contrast to impulsivity, depression is characterized by reduced behavioral vigor and enhanced aversive processing, with increased sensitivity to negative stimuli.69 However, both impulsivity and depression have been associated with low serotonergic tone, based primarily on the therapeutic efficacy of selective serotonin reuptake inhibitors (SSRIs) and observations that central serotonin depletion through dietary manipulation can induce depressive relapse.70,71 Indeed, patients with depression show reduced tryptophan levels,72 abnormal serotonin receptor function,73 abnormal serotonin transporter function,74 and elevated brain serotonin turnover.75 However, the relationship between depression and serotonin is less clear-cut than that between impulsivity and serotonin. It is possible that the link between depression and serotonin might be indirect and mediated by associative learning76 and/or disinhibition of negative thoughts.77 Overall, a number of psychiatric disorders seem to disrupt the delicate balance between serotonin and dopamine, reflecting alterations in reward, risk assessment, and social interaction. This view is supported by the wide pharmacological profiles of the major psychotropics, such as antidepressants, antipsychotics, and even drugs of abuse.
Anhedonia, which is the loss of pleasure or interest in previously rewarding stimuli, is a key pathological element of MDD and predicts antidepressant response.78 Anhedonia is associated with disruption of the frontostriatal valuation system and reward processing (Figure 2). Anhedonic depressed patients exhibit reduced caudate52 and OFC volume.79 Decreased activation of the ventral striatum during reward selection, anticipation, and feedback are found in monetary tasks in nonmedicated anhedonic depressed patients.80 Exploration of anhedonia in healthy volunteers showed decreased striatal activation and overactivation of the VMPFC during reward processing.81

The bottom highlighted area shows the brain reward valuation system: ventral tegmental area (VTA) projections, ventral striatum (VS), and orbitofrontal cortex (OFC). The top highlighted area shows the substrates of executive function: dorsolateral prefrontal cortex (DLPFC) and posterior parietal cortex. The middle highlighted area shows two brain regions involved in emotion and intuition processing: amygdala and insula.
There is debate as to whether anhedonia in depressed patients represents the inability to experience pleasure and engage in rewarding activities, or the inability to sustain positive affect. Neuroimaging data support both hypotheses. Epstein et al.80 reported that unmedicated depressed patients exhibited decreased ventral striatum and DMPFC activation with positive stimuli. Additionally, the magnitude of ventral striatum deactivation correlated with decreased interest and pleasure during daily activities. In contrast, Heller et al., 39 using an emotion regulation task found that depressed individuals were able to up-regulate positive affect, but failed to sustain NAcc activity over time. This diminished capacity to maintain positive affect was associated with decreased connectivity between the NAcc and mPFC. This concatenation of results points to an altered valuation system (frontostriatal circuit) in anhedonia, likely at the PFC level. This would be consistent with the therapeutic effects of psychotherapy such as cognitive behavioral therapy (CBT), which aims to identify and change dysfunctional patterns of thought and behavior. Furthermore, different forms of psychotherapy (e.g., interpersonal, behavioral activation, and cognitive behavioral therapy), for depression have shown normalization of PFC and striatum activity during reward tasks.81,82
Development
Depressed adolescents exhibit similar alterations of the reward system as their adult counterparts. Children between 9 and 17 years old with MDD had reduced neural response than controls in the caudate, OFC, ACC, and amygdala, as well as higher neuroactivation in the DLPFC and frontal pole during decision and outcome phases in a monetary reward task.83 In addition, diminished caudate activation was correlated with lower subjective positive affect during follow-up.83 The same group also reported that pretreatment striatal and medial PFC reactivity during a monetary reward task were predictive of response to cognitive-behavioral therapy (CBT) or pharmacological treatment. Relative to control subjects, adult individuals exposed to childhood adversity reported elevated symptoms of anhedonia and depression, rated the reward cues less positively, and displayed a weaker response to reward cues in the left globus pallidus.84 Additionally, the combination of adverse early life experience and the short polymorphism of the serotonin transporter gene enhances negativity bias (perceiving more danger and risk than reward) and physiological reactivity to negative experiences.49
On the other hand, depression in older adults presents with unique cognitive characteristics. Individuals are guided by the same essential set of socioemotional goals throughout life, such as seeking novelty, feeling needed, and expanding one’s horizons. However, the relative priority of different sets of goals changes as a function of age. Typically, despite being at risk for loss of loved ones, function, finances, and status, older adults endorse better well-being than younger counterparts. This apparent paradox is explained by the Socioemotional Selectivity theory85, which states that according to the perception of time left to live, an individual’s perceived limitations on time lead to reorganizations of goals thereby prioritizing those with emotional meaning over those that maximize long-term payoffs in the distant future. This is believed to occur through more effective cognitive control over negative affect which reflects in effective emotion regulation mediated by the ventral PFC.86 However, in the less frequent cases when depression occurs in older adults, it is typically associated with vascular insults that affect the PFC function. Because late onset depression is usually secondary to the loss of coping cognitive mechanisms in the elderly, it tends to be treatment resistant and have poorer outcomes.
Suicide is the most catastrophic outcome of depression and other psychiatric disorders, and is associated with reduced serotonergic neurotransmission, particularly within the VMPFC, including increased expression of serotonin 1 and 2 receptors87 and binding of the presynaptic serotonin 2 receptor in the VMPFC88 (for review, see89). This dearth of serotonin is thought to impair executive function, predisposing patients to become more impulsive, rigid in their thinking, and poorer decision-makers. Deficits in executive function and problem-solving are greater in depressed individuals with a history of suicide attempts or even suicidal ideation compared with depressed controls.90 Impaired decision making, reflected in poor performance in the Iowa Gambling Task (IGT), which is designed to mimic complex and uncertain decision making, is found in individuals with a past history of suicide attempts,91 in particular, in those that used violent methods.92 Euthymic patients with a history of suicide attempts showed significant deficits in executive function: impaired visuospatial conceptualization, inhibition, and visual attention (or reading fluency) suggestive of generalized PFC dysfunction, both DLPFC and VMPFC.93 It is possible that executive function deficits may be more specific to suicidal behavior rather than to any specific psychiatric diagnosis because this observation holds true for suicidal patients with depression, bipolar disorder, and even temporal lobe epilepsy. Last, poor inhibition is found in suicide attempters when compared with patients with only suicidal ideation,94 and greater cognitive executive function impairments are found in depressed patients with suicidal ideation compared with those without it.95 However, individuals with a history of suicide attempts show poorer inhibition but better problem-solving ability than suicide ideators.94 There is evidence that at least a subgroup of suicide attempters have deficits in self-regulation and temporal discounting. Depressed suicide attempters 60 years or older showed deficits in probabilistic reversal learning suggesting that this population makes present focused decisions, ignoring past experiences.96 In a similar elderly sample, Dombrovski et al.97 showed decreased temporal discounting in high-lethality suicide attempters compared with low lethality ones.
Anxiety
Anxiety is the natural response to risk and uncertainty, both of which are frequently found in everyday life (see Figure 1). The amygdala plays a central role in mediating an anxiogenic response to unpredictability.98 Additionally, the insula is a key structure involved in the prediction of risk,18 and the DLPFC is positively correlated with risk aversion.99
Fear and anxiety are closely related, and share common cognitive and physiological properties.100 Fear response is evoked by specific stimuli and tends to be transient, decreasing once a threat has dissipated. Anxiety may be experienced in the absence of a direct threat and typically persists over a longer period of time. However, anxiety is commonly conceptualized as a state of sustained fear.101 Fear conditioning has been a most successful research model to understand the biology of anxiety. Animal and human research on fear conditioning has highlighted the central role of the amygdala in fear acquisition, storage, and expression.101–103 The amygdala’s projections to different parts of the brain have been associated with specific functions. For instance, projections to the brainstem and hypothalamus mediate autonomic fear expression, projections to the ventral striatum mediate the use of actions to cope with fear,102 hippocampal projections are involved in contextually dependent expression of fear,104 and connectivity to the VMPFC is required for inhibition or control of conditioned fear and storage of extinction memory5,104,105
Two principal information-processing biases are characteristic of anxiety: (1) a bias to attend toward threat-related information, and (2) a bias toward negative interpretation of ambiguous stimuli.106 Anxiety is associated with faster response times when detecting a threat or negative stimuli or identifying a target cued by a threat stimulus, and slower response times when detecting a neutral stimulus or reporting neutral information in the presence of a threat stimulus.107–109 This attentional bias reflects both facilitated detection of threat-related stimuli and difficulty in disengaging attention from negative stimuli,107 and seems to be related to both the engagement of preattentive amygdala-dependent threat evaluation processes110 and impaired prefrontal control mechanisms typically engaged during attentional competition and control.111 Consistent with this view, high trait anxiety is associated with increased amygdala activity to attended as well as unattended threat stimuli112 and decreased prefrontal activation under conditions of attention competition,111,112 even in the absence of threat-related stimuli.113
Anxious individuals unrealistically judge negative outcomes to be more likely than positive ones.114–116 Higher trait anxiety is associated with heightened amygdala blood-oxygen-level-dependent (BOLD)-responses during passive viewing of neutral faces117 and a tendency to interpret neutral faces more negatively.118 For instance, anxious individuals tend to interpret ambiguous emotional facial expressions,119 face-voice pairings,120 and homophones121 as more negative in valence than less-anxious individuals.
Anxiety as a trait has been amply studied in healthy subjects. Trait anxiety, worry, and social anxiety in healthy participants are predictive of heightened risk aversion.122–124 In turn, heightened arousal to risky choices or increased interoceptive awareness of arousal responses (or an interaction of the two) may lead anxious individuals to be more risk averse. Trait anxiety is also associated with greater susceptibility to the framing effect (i.e., reacting differently whether a choice is presented in terms of gain or loss).125
The circuitry involved in the learning and regulation of conditioned fear is altered in healthy individuals with the anxiety trait and in patients suffering from anxiety disorders. Trait anxiety is associated with heightened amygdala activation as well as elevated fear expression during fear acquisition.126,127 Anxiety also impairs extinction learning and retention126–128 as well as the regulation of emotional responses via intentional cognitive strategies.107,129 Patients with panic disorder show an increased generalization of conditioned fear to similar stimuli.130 Atrophy of the hippocampus in posttraumatic stress disorder patients suggests that contextual modulation of fear may also be altered in anxiety.131
Although clinical data are still limited,105 there is clear evidence of negative attentional biases in several anxiety disorders including generalized anxiety disorder, posttraumatic stress disorder, social and specific phobias, and obsessive-compulsive disorder (for review see107). Amygdala hyper-responsivity while attending to, evaluating, and anticipating negative stimuli may heighten the cognitive and affective responses to a potential threat in anxious individuals. Thus, the everyday decisions made by individuals suffering from anxiety disorders to avoid exaggerated perceived threats can have a profound impact on the ability to function adaptively.
Bipolar Disorder
Bipolar disorder and schizophrenia share impairments in similar cognitive areas including attention, processing speed, verbal memory, learning, and executive function, although bipolar disorder deficits are usually less severe.132 These cognitive impairments represent a substantial clinical problem in up to 60% of bipolar disorder patients133 and can be found in depressed, manic, and mixed episodes as well as in the euthymic state. This pervasive impairment in cognitive function in bipolar disorder suggests it may be a trait marker associated with genetic vulnerability.
A real life consequence of these cognitive deficits is functional impairment in several spheres including independent living, social relationships, and vocational success. For instance, 20% of patients with bipolar disorder are married in contrast to 60% of the general population; approximately 60% of bipolar patients are unemployed compared with 6% in the general population; and 19%−58% are not living independently.134 These functional impairments are present at the time of the first episode and persist over time.135
In addition to the pervasive alterations in the cognitive sphere, emotion processing is markedly disrupted in patients suffering from bipolar disorder. It has been proposed that central to bipolar disease is a heightened processing of positive emotion regardless of the context.136 A recent meta-analysis identified significant deficits in theory of mind and emotion processing in euthymic bipolar patients.137 Emotion processing in depressed bipolar patients appears to involve a partially overlapping neural network with that of major depression, but with distinct roles of the VLPFC and thalamus.138 Thus, bipolar patients are impaired in their ability to identify other individuals’ emotions and intentions, with a resultant impact on everyday functioning.
Despite these deficits in cognition and emotion processing, the findings on decision making are heterogeneous in bipolar disorder. For instance, manic or hypomanic patients tend to make suboptimal choices in the Cambridge Gambling Task,139 they are more sensitive to error processing during a two choice prediction task,140 they show steep temporal discounting,141 and deficits in response disinhibition and inattention (142, but see also143,144). These deficits are not exclusive to mania, since depressed bipolar patients evidence deficits in reward processing, short-term memory, and sensitivity to negative feedback.145 Moreover, euthymic patients with bipolar disorder also display moderate to severe deficits in a wide variety of executive function measures including category fluency, mental manipulation, verbal learning, abstraction, set-shifting, sustained attention, response inhibition, and psychomotor speed (146–148, but see also91,149,150). Furthermore, even first degree relatives of bipolar disorder patients show executive function deficits (i.e., attentional set shifting).151 In addition to moderate to severe neuropsychological impairments, there seems to be specific cognitive and decision making biases in bipolar patients including impulsivity, exaggerated positive emotion, and deficits in risk assessment and reward processing. The weights of these cognitive and decision-making impairments are evident in the somber functional outcomes of patients with bipolar disorder.
Schizophrenia
Schizophrenia is a severe psychiatric disorder that afflicts approximately 1% of the population worldwide. It is characterized by alterations in higher function including thought, perception, mood, and behavior. The majority of people with schizophrenia do not attain “normal” milestones in social functioning, productivity, residence, and self-care. For instance, less than 20% of schizophrenic patients are responsible for their housing, almost 80% are unemployed, and less than 15% are married or in stable relationships.152 This functional impairment occurs despite adequate symptom control that is attained by 30%−70% of patients. A large body of evidence has demonstrated significant cognitive dysfunction in schizophrenia, which is associated with disorganization, negative symptoms, and impaired functional outcome.153 Information-processing deficits in schizophrenia are described in attention, working memory, inhibition, and context processing.154 Context-processing deficits are associated with working memory impairments and dopaminergic tone in the PFC (Table 3).155 Moreover, both cognitive control and social cognition (e.g., theory of mind) deficits are disease outcome predictors and suitable candidates for therapeutic interventions.152,156 It is no surprise that this faulty information processing in schizophrenic patients translates into impaired risk assessment,75,76 reward processing,157 and temporal discounting.158,159 The latter are correlated with working memory deficits.159,160
A pervasive clinical challenge in schizophrenia is the elevated comorbidity with substance abuse disorders. Approximately half of schizophrenic patients present a lifetime history of substance abuse disorders,161 and 75%−90% are current smokers.162,163 These extraordinary high rates of substance-use comorbidity may be explained by disrupted reward processing.164 Thus, patients with schizophrenia who are smokers display a stronger subjective response and intensity of demands to smoking than the general population smokers.165,166 In sum, patients with schizophrenia exhibit global cognitive control dysfunction that is reflected in specific deficits in risk assessment, reward processing, and temporal discounting. These impairments are translated in the devastating functional toll of this disorder.
Possible Interventions
There are already successful therapeutic approaches focused on improving cognition and decision-making capabilities of psychiatric patients: cognitive remediation and variations of cognitive-behavioral therapy (CBT), which aim to enhance emotional cognitive control and decision-making skills. Cognitive remediation is based on enhancing specific cognitive processes in order to modify the course and morbidities of disease. Recent advances in cognitive remediation strategies and delivery methods have notably increased the success of this intervention.167 These advances include (1) the development of multidimensional tests of executive functioning that drill and practice with low-level cognitive demands, (2) the dynamic adjustment of difficulty in order to maintain a constant difficulty level, and (3) the development of understanding the minimum effective dose and optimal spacing of practice trials. Successful examples are found in areas as diverse as schizophrenia,168 bipolar disorder,169 attention deficit hyperactivity disorder (ADHD),170 dyslexia,171 traumatic brain injury,172 and stroke.173 In these studies, cognitive remediation was associated with improvement in hyperactivity, organizational skills, affect, sustained attention, and temporal discounting.174 Moreover, improvements induced by cognitive remediation correlate with discrete changes in neural activity,175 noneffortful auditory evoked potentials,176 peripheral biomarkers as brain-derived neurotrophic factor (BDNF),177 as well as improvements in everyday functioning. Furthermore, considering the close association between cognitive impairments and poor functional outcome, cognitive remediation aimed at social cognitive impairments has proven effective in enhancing social functioning. Social cognitive remediation programs are either targeted to a specific social cognitive domain (e.g., emotion perception), or broad-based, combining a variety of psychosocial approaches, including cognitive remediation, social skills training, and social cognitive skill building.178,179 Both approaches have proved effective in improving social cognitive processes in schizophrenia.180,181
Two examples of the successful adaptation of cognitive-behavioral therapy to enhance decision making are dialectical behavior therapy and problem-solving therapy. Dialectical behavior therapy (DBT) was the first psychological treatment for borderline personality disorder tested in a clinical trial.182 It is specifically designed to target the emotion dysregulation (mood instability) and to reduce impulsive behaviors (in other words, enhancing emotional cognitive control). It applies behavioral analysis to incidents leading to self-injury and overdoses, teaching patients alternative ways to handle dysphoric emotions. DBT has been used successfully in patients with borderline personality disorder, eating disorders, and parasuicidal behavior. Problem-solving therapy (PST) is a cognitive-behavioral intervention that focuses on training in adaptive problem-solving attitudes and skills.183 It aims to reduce and prevent psychopathology and enhance positive well-being by helping individuals cope more effectively with stressful problems in living. PST is based on a relational/problem-solving model of stress and well-being (psychological, social, and health functioning) in which social problem solving (i.e., real-life problem solving) is assumed to play an important role as a mediator and a moderator of the relationship between stressful life events (major negative events as well as daily problems) and well-being. It has proven effective for the treatment of depression.184
Conclusions
It is not surprising that severe mental illness affects patients’ lives not only because of the cardinal disease symptoms, but also by impairing their ability to make functional and healthy decisions. This impairment may be related to disorganization or global deficits in attention, working memory, and language, such as those observed in schizophrenia and bipolar disorder. However, it can be subtler and lead to abnormal risk assessment or reward processing as found in depression. These pervasive deficits translate into medication and medical appointment nonadherence, poor diet and exercise, and drug use, which in turn leads to poor quality of life and clinical outcomes such as relapse, hospitalization, death, and incarceration.
A multidisciplinary approach to how the brain makes decisions may provide a new theory of thought with quantifiable parameters that can increase understanding of mental processes (decision making) in health and disease. The study of two particular constructs, reward and temporal discounting, has shed light on our understanding of severe mental illness. The utilization of additional paradigms can further lead to interventions to address every day situations that patients with mental illness face. The consideration of decision-making patterns in the assessment and management of patients with mental illness might yield evidence-based interventions with real life impact such as cognitive remediation.
1 : Neurocognitive deficits and functional outcome in schizophrenia: are we measuring the “right stuff”? Schizophr Bull 2000; 26:119–136Crossref, Medline, Google Scholar
2 : Cerebellar involvement in executive control. Cerebellum 2007; 6:184–192Crossref, Medline, Google Scholar
3 : From cognitive to neural models of working memory. Philos Trans R Soc Lond B Biol Sci 2007; 362:761–772Crossref, Medline, Google Scholar
4 : Frontal lobe and cognitive development. J Neurocytol 2002; 31:373–385Crossref, Medline, Google Scholar
5 : Functional imaging studies of emotion regulation: a synthetic review and evolving model of the cognitive control of emotion. Ann N Y Acad Sci 2012; 1251:E1–E24Crossref, Medline, Google Scholar
6 : Meta-analytic evidence for a superordinate cognitive control network subserving diverse executive functions. Cogn Affect Behav Neurosci 2012; 12:241–268Crossref, Medline, Google Scholar
7 : General and specific functional connectivity disturbances in first-episode schizophrenia during cognitive control performance. Biol Psychiatry 2011; 70:64–72Crossref, Medline, Google Scholar
8 : Explicit neural signals reflecting reward uncertainty. Philos Trans R Soc Lond B Biol Sci 2008; 363:3801–3811Crossref, Medline, Google Scholar
9 . Opponency revisited: competition and cooperation between dopamine and serotonin. Neuropsychopharmacology 2011; 36:74–97Google Scholar
10 Neural antecedents of financial decisions. J Neurosci 2007; 27:8174–8177Google Scholar
11 : Neural economics and the biological substrates of valuation. Neuron 2002; 36:265–284Crossref, Medline, Google Scholar
12 : Anterior cingulate activity modulates nonlinear decision weight function of uncertain prospects. Neuroimage 2006; 30:668–677Crossref, Medline, Google Scholar
13 : Probing human and monkey anterior cingulate cortex in variable environments. Cogn Affect Behav Neurosci 2007; 7:413–422Crossref, Medline, Google Scholar
14 : Prospect theory: an analysis of decisions under risk. Econometrica 1979; 47:313–327Crossref, Google Scholar
15 Choosing between small, likely rewards and large, unlikely rewards activates inferior and orbital prefrontal cortex. J Neurosci 1999; 19:9029–9038Google Scholar
16 : The neural basis of financial risk taking. Neuron 2005; 47:763–770Crossref, Medline, Google Scholar
17 . Dissociable systems for gain- and loss-related value predictions and errors of prediction in the human brain. J Neurosci 2006; 26:9530–9537Google Scholar
18 . Human insula activation reflects risk prediction errors as well as risk. J Neurosci 2008; 28:2745–2752Google Scholar
19 : Separate neural mechanisms underlie choices and strategic preferences in risky decision making. Neuron 2009; 62:593–602Crossref, Medline, Google Scholar
20 : Why am I unsure? Internal and external attributions of uncertainty dissociated by fMRI. Neuroimage 2004; 21:848–857Crossref, Medline, Google Scholar
21 : Neural activity in the human brain relating to uncertainty and arousal during anticipation. Neuron 2001; 29:537–545Crossref, Medline, Google Scholar
22 . Decisions under uncertainty: probabilistic context influences activation of prefrontal and parietal cortices. J Neurosci 2005; 25:3304–3311Google Scholar
23 : A neural representation of categorization uncertainty in the human brain. Neuron 2006; 49:757–763Crossref, Medline, Google Scholar
24 : Breakdown of Will. Cambridge, Cambridge University Press, 2008Crossref, Google Scholar
25 : Willpower in a cognitive-affective processing system, in Handbook of Self-Regulation: Research, Theory, and Applications. Edited by Baumeister FVohs KD. New York, Guildford Press, 1989, pp 99–129Google Scholar
26 : Regulating the interpersonal self: strategic self-regulation for coping with rejection sensitivity. J Pers Soc Psychol 2000; 79:776–792Crossref, Medline, Google Scholar
27 : The role of ego-control, ego-resiliency, and IQ in delay of gratification in adolescence. J Pers Soc Psychol 1989; 57:1041–1050Crossref, Medline, Google Scholar
28 : Separate neural systems value immediate and delayed monetary rewards. Science 2004; 306:503–507Crossref, Medline, Google Scholar
29 : Prediction of immediate and future rewards differentially recruits cortico-basal ganglia loops. Nat Neurosci 2004; 7:887–893Crossref, Medline, Google Scholar
30 : Human autonomy and the frontal lobes. Part II: patient behavior in complex and social situations: the “environmental dependency syndrome.” Ann Neurol 1986; 19:335–343Crossref, Medline, Google Scholar
31 : The neural correlates of subjective value during intertemporal choice. Nat Neurosci 2007; 10:1625–1633Crossref, Medline, Google Scholar
32 . Neural dissociation of delay and uncertainty in intertemporal choice. J Neurosci 2008; 28:14459–14466Google Scholar
33 : The neural substrates of probabilistic and intertemporal decision making. Brain Res 2008; 1234:104–115Crossref, Medline, Google Scholar
34 : Role of affect in decision making. Prog Brain Res 2013; 202:37–53Crossref, Medline, Google Scholar
35 : The role of affect in decision making, in Handbook of Affective Science. Edited by Dawson RJScherer KRGoldsmith HH. Oxford, Oxford University Press, 2003, pp 619–642Google Scholar
36 : The multiplicity of emotions: A framework of emotional functions in decision making. Judgm Decis Mak 2008; 3:5–17Google Scholar
37 : Affective neuroscience: the foundations of human and animal emotions. New York, Oxford University Press, 2004Google Scholar
38 : The Iowa Gambling Task and the somatic marker hypothesis: some questions and answers. Trends Cogn Sci 2005; 9:159–162, discussion 162–164Crossref, Medline, Google Scholar
39 : Reduced capacity to sustain positive emotion in major depression reflects diminished maintenance of fronto-striatal brain activation. Proc Natl Acad Sci USA 2009; 106:22445–22450Crossref, Medline, Google Scholar
40 : Implicit knowledge: new perspectives on unconscious processes. Proc Natl Acad Sci USA 1992; 89:11113–11117Crossref, Medline, Google Scholar
41 : The Discovery of the Unconscious; the History and Evolution of Dynamic Psychiatry. New York, Basic Books, 1970Google Scholar
42 . Unconscious knowledge: a survey. Adv Cogn Psychol 2010; 6:116–141Google Scholar
43 . The role of consciousness in cognitive control and decision making. Front Hum Neurosci 2012; 6:121Google Scholar
44 : Integration without awareness: expanding the limits of unconscious processing. Psychol Sci 2011; 22:764–770Crossref, Medline, Google Scholar
45 : Rapid parallel semantic processing of numbers without awareness. Cognition 2011; 120:136–147Crossref, Medline, Google Scholar
46 : Playing chess unconsciously. J Exp Psychol Learn Mem Cogn 2009; 35:292–298Crossref, Medline, Google Scholar
47 : Long-lasting effects of subliminal affective priming from facial expressions. Conscious Cogn 2009; 18:929–938Crossref, Medline, Google Scholar
48 : On making the right choice: the deliberation-without-attention effect. Science 2006; 311:1005–1007Crossref, Medline, Google Scholar
49 : ‘Negativity bias’ in risk for depression and anxiety: brain-body fear circuitry correlates, 5-HTT-LPR and early life stress. Neuroimage 2009; 47:804–814Crossref, Medline, Google Scholar
50 : Is depression associated with dysfunction of the central reward system? Biochem Soc Trans 2009; 37:313–317Crossref, Medline, Google Scholar
51 : Self-assessment of functional status in schizophrenia. J Psychiatr Res 2007; 41:1012–1018Crossref, Medline, Google Scholar
52 : Reduced caudate and nucleus accumbens response to rewards in unmedicated individuals with major depressive disorder. Am J Psychiatry 2009; 166:702–710Crossref, Medline, Google Scholar
53 : A double dissociation of ventromedial prefrontal cortical responses to sad and happy stimuli in depressed and healthy individuals. Biol Psychiatry 2005; 58:495–503Crossref, Medline, Google Scholar
54 : Abnormal neural response to feedback on planning and guessing tasks in patients with unipolar depression. Psychol Med 1998; 28:559–571Crossref, Medline, Google Scholar
55 : Neural responses to monetary incentives in major depression. Biol Psychiatry 2008; 63:686–692Crossref, Medline, Google Scholar
56 : The impact of depression on social economic decision making. J Abnorm Psychol 2010; 119:440–446Crossref, Medline, Google Scholar
57 : The role of dopamine in the pathophysiology of depression. Arch Gen Psychiatry 2007; 64:327–337Crossref, Medline, Google Scholar
58 : Neuroimaging markers of cellular function in major depressive disorder: implications for therapeutics, personalized medicine, and prevention. Clin Pharmacol Ther 2012; 91:201–214Crossref, Medline, Google Scholar
59 . Dopamine type-1 receptor binding in major depressive disorder assessed using positron emission tomography and [11C]NNC-112. Neuropsychopharmacology 2009; 34:1277–1287Google Scholar
60 : Functional architecture of basal ganglia circuits: neural substrates of parallel processing. Trends Neurosci 1990; 13:266–271Crossref, Medline, Google Scholar
61 : Basal ganglia functional connectivity based on a meta-analysis of 126 positron emission tomography and functional magnetic resonance imaging publications. Cereb Cortex 2006; 16:1508–1521Crossref, Medline, Google Scholar
62 : Brain serotonin synthesis in adult males characterized by physical aggression during childhood: a 21-year longitudinal study. PLoS ONE 2010; 5:e11255Crossref, Medline, Google Scholar
63 : Aggression in humans correlates with cerebrospinal fluid amine metabolites. Psychiatry Res 1979; 1:131–139Crossref, Medline, Google Scholar
64 . Aggression, suicidality, and intermittent explosive disorder: serotonergic correlates in personality disorder and healthy control subjects. Neuropsychopharmacology 2010; 35:435–444Google Scholar
65 : Serotonin and emotional processing: does it help explain antidepressant drug action? Neuropharmacology 2008; 55:1023–1028Crossref, Medline, Google Scholar
66 : 5-HT and mechanisms of defense. J Psychopharmacol 1991; 5:305–315Crossref, Medline, Google Scholar
67 : Serotoninergic regulation of emotional and behavioural control processes. Trends Cogn Sci 2008; 12:31–40Crossref, Medline, Google Scholar
68 : Reward and punishment processing in depression. Biol Psychiatry 2010; 68:118–124Crossref, Medline, Google Scholar
69 : Neurocognitive mechanisms in depression: implications for treatment. Annu Rev Neurosci 2009; 32:57–74Crossref, Medline, Google Scholar
70 : Serotonin function and the mechanism of antidepressant action. Reversal of antidepressant-induced remission by rapid depletion of plasma tryptophan. Arch Gen Psychiatry 1990; 47:411–418Crossref, Medline, Google Scholar
71 : Relapse of depression after rapid depletion of tryptophan. Lancet 1997; 349:915–919Crossref, Medline, Google Scholar
72 : Decreased plasma tryptophan levels in major depression. J Affect Disord 1989; 16:27–31Crossref, Medline, Google Scholar
73 : PET imaging of serotonin 1A receptor binding in depression. Biol Psychiatry 1999; 46:1375–1387Crossref, Medline, Google Scholar
74 : Imaging of the serotonergic system: interactions of neuroanatomical and functional abnormalities of depression. Biol Psychiatry 1998; 44:534–549Crossref, Medline, Google Scholar
75 : Elevated brain serotonin turnover in patients with depression: effect of genotype and therapy. Arch Gen Psychiatry 2008; 65:38–46Crossref, Medline, Google Scholar
76 : Recurrence in major depressive disorder: a neurocognitive perspective. Psychol Med 2008; 38:315–318Crossref, Medline, Google Scholar
77 : Serotonin, inhibition, and negative mood. PLOS Comput Biol 2008; 4:e4Crossref, Medline, Google Scholar
78 : Diminished neural processing of aversive and rewarding stimuli during selective serotonin reuptake inhibitor treatment. Biol Psychiatry 2010; 67:439–445Crossref, Medline, Google Scholar
79 : Anatomic evaluation of the orbitofrontal cortex in major depressive disorder. Biol Psychiatry 2004; 55:353–358Crossref, Medline, Google Scholar
80 : Lack of ventral striatal response to positive stimuli in depressed versus normal subjects. Am J Psychiatry 2006; 163:1784–1790Link, Google Scholar
81 : The effects of psychotherapy on neural responses to rewards in major depression. Biol Psychiatry 2009; 66:886–897Crossref, Medline, Google Scholar
82 : Regional brain metabolic changes in patients with major depression treated with either paroxetine or interpersonal therapy: preliminary findings. Arch Gen Psychiatry 2001; 58:631–640Crossref, Medline, Google Scholar
83 : Altered striatal activation predicting real-world positive affect in adolescent major depressive disorder. Am J Psychiatry 2009; 166:64–73Crossref, Medline, Google Scholar
84 : Childhood adversity is associated with left basal ganglia dysfunction during reward anticipation in adulthood. Biol Psychiatry 2009; 66:206–213Crossref, Medline, Google Scholar
85 : Socioemotional selectivity theory, aging, and health: the increasingly delicate balance between regulating emotions and making tough choices. J Pers 2004; 72:1395–1424Crossref, Medline, Google Scholar
86 : The Role of Cognitive Control in Older Adults' Emotional Well-Being, in Handbook of Theories of Aging, 2nd ed. Edited by Bengtson VGans DPutney NSilverstein M. New York, Springer, 2009, 790 pp.Google Scholar
87 : Higher expression of serotonin 5-HT(2A) receptors in the postmortem brains of teenage suicide victims. Am J Psychiatry 2002; 159:419–429Crossref, Medline, Google Scholar
88 : Increased serotonin-2 binding sites in frontal cortex of suicide victims. Lancet 1983; 1:214–216Crossref, Medline, Google Scholar
89 : Neurobiology of suicidal behaviour. Nat Rev Neurosci 2003; 4:819–828Crossref, Medline, Google Scholar
90 : Executive performance of depressed suicide attempters: the role of suicidal ideation. Eur Arch Psychiatry Clin Neurosci 2008; 258:414–421Crossref, Medline, Google Scholar
91 : Decision making in euthymic bipolar I and bipolar II disorders. Psychol Med 2011; 41:1319–1327Crossref, Medline, Google Scholar
92 : Impaired decision making in suicide attempters. Am J Psychiatry 2005; 162:304–310Crossref, Medline, Google Scholar
93 : Prefrontal cortex dysfunction in patients with suicidal behavior. Psychol Med 2007; 37:411–419Crossref, Medline, Google Scholar
94 : Differential effects of executive functioning on suicide attempts. J Neuropsychiatry Clin Neurosci 2011; 23:173–179Link, Google Scholar
95 : Executive functioning in depressed patients with suicidal ideation. Acta Psychiatr Scand 2005; 112:294–301Crossref, Medline, Google Scholar
96 : Reward/Punishment reversal learning in older suicide attempters. Am J Psychiatry 2010; 167:699–707Crossref, Medline, Google Scholar
97 : Lethal forethought: delayed reward discounting differentiates high- and low-lethality suicide attempts in old age. Biol Psychiatry 2011; 70:138–144Crossref, Medline, Google Scholar
98 Processing of temporal unpredictability in human and animal amygdala. J Neurosci 2007; 27:5958–5966Google Scholar
99 . Neural correlates of value, risk, and risk aversion contributing to decision making under risk. J Neurosci 2009; 29:12574–12583Google Scholar
100 : Differences between trait fear and trait anxiety: implications for psychopathology. Clin Psychol Rev 2011; 31:122–137Crossref, Medline, Google Scholar
101 . Phasic vs sustained fear in rats and humans: role of the extended amygdala in fear vs anxiety. Neuropsychopharmacology 2010; 35:105–135Google Scholar
102 The amygdala. Curr Biol 2007; 17:R868–R874Google Scholar
103 : Extending animal models of fear conditioning to humans. Biol Psychol 2006; 73:39–48Crossref, Medline, Google Scholar
104 : Contextual fear, gestalt memories, and the hippocampus. Behav Brain Res 2000; 110:73–81Crossref, Medline, Google Scholar
105 . Changing fear: the neurocircuitry of emotion regulation. Neuropsychopharmacology 2010; 35:136–146Google Scholar
106 : Cognitive vulnerability to emotional disorders. Annu Rev Clin Psychol 2005; 1:167–195Crossref, Medline, Google Scholar
107 : Mechanisms of attentional biases towards threat in anxiety disorders: an integrative review. Clin Psychol Rev 2010; 30:203–216Crossref, Medline, Google Scholar
108 : A cognitive-motivational analysis of anxiety. Behav Res Ther 1998; 36:809–848Crossref, Medline, Google Scholar
109 : Threat-related attentional bias in anxious and nonanxious individuals: a meta-analytic study. Psychol Bull 2007; 133:1–24Crossref, Medline, Google Scholar
110 : A cognitive model of selective processing in anxiety. Cognit Ther Res 1998; 22:539–560Crossref, Google Scholar
111 : Neural processing of fearful faces: effects of anxiety are gated by perceptual capacity limitations. Cereb Cortex 2007; 17:1595–1603Crossref, Medline, Google Scholar
112 . State anxiety modulation of the amygdala response to unattended threat-related stimuli. J Neurosci 2004; 24:10364–10368Google Scholar
113 : Trait anxiety and impoverished prefrontal control of attention. Nat Neurosci 2009; 12:92–98Crossref, Medline, Google Scholar
114 : Anticipatory anxiety and risk perception. Cognit Ther Res 1987; 11:551–565Crossref, Google Scholar
115 : Worry is reasonable: the role of explanations in pessimism about future personal events. J Abnorm Psychol 1991; 100:478–486Crossref, Medline, Google Scholar
116 : Anxiety and cognitive performance: attentional control theory. Emotion 2007; 7:336–353Crossref, Medline, Google Scholar
117 : Human amygdala responses during presentation of happy and neutral faces: correlations with state anxiety. Biol Psychiatry 2004; 55:897–903Crossref, Medline, Google Scholar
118 : Interpreting neutral faces as threatening is a default mode for socially anxious individuals. J Abnorm Psychol 2008; 117:680–685Crossref, Medline, Google Scholar
119 : Anxiety-related bias in the classification of emotionally ambiguous facial expressions. Emotion 2002; 2:273–287Crossref, Medline, Google Scholar
120 The effects of anxiety on the interpretation of emotion in the face-voice pairs. Exp Brain Res 2011; 213:275–282Google Scholar
121 : Cognitive functioning and anxiety. Psychol Res 1987; 49:189–195Crossref, Medline, Google Scholar
122 : Who takes risks? Daring and caution in foreign policy making. J Conflict Resolut 1997; 41:611–637Crossref, Google Scholar
123 : All negative moods are not equal: motivational influences of anxiety and sadness on decision making. Organ Behav Hum Decis Process 1999; 79:56–77Crossref, Medline, Google Scholar
124 : Dispositional anxiety and risk-avoidant decision-making. Pers Individ Dif 2007; 42:665–675Crossref, Google Scholar
125 : Personality traits and risky decision making in a controlled experimental task: an exploratory study. Pers Ind Diff 2001; 31:215–226Crossref, Google Scholar
126 : Classical fear conditioning in the anxiety disorders: a meta-analysis. Behav Res Ther 2005; 43:1391–1424Crossref, Medline, Google Scholar
127 : Fear-conditioning mechanisms associated with trait vulnerability to anxiety in humans. Neuron 2011; 69:563–571Crossref, Medline, Google Scholar
128 : Orbitofrontal thickness, retention of fear extinction, and extraversion. Neuroreport 2005; 16:1909–1912Crossref, Medline, Google Scholar
129 : Emotion regulation and anxiety disorders. J Anxiety Disord 2008; 22:211–221Crossref, Medline, Google Scholar
130 : Overgeneralization of conditioned fear as a pathogenic marker of panic disorder. Am J Psychiatry 2010; 167:47–55Crossref, Medline, Google Scholar
131 : MRI-based measurement of hippocampal volume in patients with combat-related posttraumatic stress disorder. Am J Psychiatry 1995; 152:973–981Crossref, Medline, Google Scholar
132 : Cognition and disability in bipolar disorder: lessons from schizophrenia research. Bipolar Disord 2010; 12:364–375Crossref, Medline, Google Scholar
133 : Heterogeneity in cognitive functioning among patients with bipolar disorder. J Affect Disord 2008; 109:149–156Crossref, Medline, Google Scholar
134 : Disability and its treatment in bipolar disorder patients. Bipolar Disord 2007; 9:183–196Crossref, Medline, Google Scholar
135 : The McLean-Harvard First-Episode Mania Study: prediction of recovery and first recurrence. Am J Psychiatry 2003; 160:2099–2107Crossref, Medline, Google Scholar
136 : A review and synthesis of positive emotion and reward disturbance in bipolar disorder. Clin Psychol Psychother 2011; 18:356–365Crossref, Medline, Google Scholar
137 : Social cognition in euthymic bipolar disorder: systematic review and meta-analytic approach. Acta Psychiatr Scand 2012; 125:266–280Crossref, Medline, Google Scholar
138 : Common and distinct neural correlates of emotional processing in bipolar disorder and major depressive disorder: a voxel-based meta-analysis of functional magnetic resonance imaging studies. Eur Neuropsychopharmacol 2012; 22:100–113Crossref, Medline, Google Scholar
139 : Decision-making cognition in mania and depression. Psychol Med 2001; 31:679–693Crossref, Medline, Google Scholar
140 : Increased sensitivity to error during decision-making in bipolar disorder patients with acute mania. J Affect Disord 2004; 82:203–208Crossref, Medline, Google Scholar
141 : I want it now! Neural correlates of hypersensitivity to immediate reward in hypomania. Biol Psychiatry 2012; 71:530–537Crossref, Medline, Google Scholar
142 : Characterizing impulsivity in mania. Bipolar Disord 2009; 11:41–51Crossref, Medline, Google Scholar
143 : A neuropsychological investigation of prefrontal cortex involvement in acute mania. Am J Psychiatry 2001; 158:1605–1611Crossref, Medline, Google Scholar
144 : Decision-making in mania: a PET study. Brain 2001; 124:2550–2563Crossref, Medline, Google Scholar
145 : Hot and cold cognition in unmedicated depressed subjects with bipolar disorder. Bipolar Disord 2009; 11:178–189Crossref, Medline, Google Scholar
146 : A meta-analysis of cognitive deficits in euthymic patients with bipolar disorder. J Affect Disord 2006; 93:105–115Crossref, Medline, Google Scholar
147 Neurocognitive impairment in euthymic patients with bipolar affective disorder. Br J Psychiatry 2005; 186:32–40Google Scholar
148 : Neuropsychological functioning in euthymic bipolar disorder: a meta-analysis. Acta Psychiatr Scand Suppl 2007; 434:17–26Crossref, Medline, Google Scholar
149 : Processing of differentially valued rewards and punishments in youths with bipolar disorder or severe mood dysregulation. J Child Adolesc Psychopharmacol 2008; 18:185–196Crossref, Medline, Google Scholar
150 : Reward-related processes in pediatric bipolar disorder: a pilot study. J Affect Disord 2004; 82(Suppl 1):S89–S101Crossref, Medline, Google Scholar
151 : Impairment of executive function but not memory in first-degree relatives of patients with bipolar I disorder and in euthymic patients with unipolar depression. Am J Psychiatry 2005; 162:1980–1982Crossref, Medline, Google Scholar
152 : Performance-based measurement of functional disability in schizophrenia: a cross-national study in the United States and Sweden. Am J Psychiatry 2009; 166:821–827Crossref, Medline, Google Scholar
153 . Cognitive control deficits in schizophrenia: mechanisms and meaning. Neuropsychopharmacology 2011; 36:316–338Google Scholar
154 : Context-processing deficits in schizophrenia: converging evidence from three theoretically motivated cognitive tasks. J Abnorm Psychol 1999; 108:120–133Crossref, Medline, Google Scholar
155 : Updating of context in working memory: an event-related potential study. Cogn Affect Behav Neurosci 2010; 10:298–315Crossref, Medline, Google Scholar
156 : Social cognition: the key factor predicting social outcome in people with schizophrenia? Psychiatry (Edgmont) 2010; 7:41–44Medline, Google Scholar
157 : Anticipated, on-line and remembered positive experience in schizophrenia. Schizophr Res 2010; 122:199–205Crossref, Medline, Google Scholar
158 : Risk/reward decision-making in schizophrenia: a preliminary examination of the influence of tobacco smoking and relationship to Wisconsin Card Sorting Task performance. Schizophr Res 2009; 110:156–164Crossref, Medline, Google Scholar
159 : Temporal discounting of rewards in patients with bipolar disorder and schizophrenia. J Abnorm Psychol 2011; 120:911–921Crossref, Medline, Google Scholar
160 : Imagining the future: degraded representations of future rewards and events in schizophrenia. J Abnorm Psychol 2011; 120:483–489Crossref, Medline, Google Scholar
161 : Substance use disorders in schizophrenia—clinical implications of comorbidity. Schizophr Bull 2009; 35:469–472Crossref, Medline, Google Scholar
162 : Cigarette smoking in schizophrenia: relationship to psychopathology and medication side effects. Am J Psychiatry 1992; 149:1189–1194Crossref, Medline, Google Scholar
163 : Schizophrenia and smoking: an epidemiological survey in a state hospital. Am J Psychiatry 1995; 152:453–455Crossref, Medline, Google Scholar
164 : The vulnerability to alcohol and substance abuse in individuals diagnosed with schizophrenia. Neurotox Res 2006; 10:235–252Crossref, Medline, Google Scholar
165 : Cigarette demand and delayed reward discounting in nicotine-dependent individuals with schizophrenia and controls: an initial study. Psychopharmacology (Berl) 2011; 216:91–99Crossref, Medline, Google Scholar
166 : Reward value of cigarette smoking for comparably heavy smoking schizophrenic, depressed, and nonpatient smokers. Am J Psychiatry 2003; 160:316–322Crossref, Medline, Google Scholar
167 : A meta-analysis of cognitive remediation in schizophrenia. Am J Psychiatry 2007; 164:1791–1802Crossref, Medline, Google Scholar
168 : Cognitive training and supported employment for persons with severe mental illness: one-year results from a randomized controlled trial. Schizophr Bull 2005; 31:898–909Crossref, Medline, Google Scholar
169 : RESEARCH: Cognitive rehabilitation for bipolar disorder: an open trial for employed patients with residual depressive symptoms. CNS Neurosci Ther 2010; 16:298–307Crossref, Medline, Google Scholar
170 : Cognitive remediation in ADHD: effects of periodic non-contingent alerts on sustained attention to response. Neuropsychol Rehabil 2006; 16:653–665Crossref, Medline, Google Scholar
171 : Altering cortical connectivity: remediation-induced changes in the white matter of poor readers. Neuron 2009; 64:624–631Crossref, Medline, Google Scholar
172 : A trial of neuropsychologic rehabilitation in mild-spectrum traumatic brain injury. Arch Phys Med Rehabil 2005; 86:1565–1574Crossref, Medline, Google Scholar
173 : Cognitive rehabilitation of memory following stroke. Theory, practice, and outcome. Adv Neurol 2003; 92:415–421Medline, Google Scholar
174 : Remember the future: working memory training decreases delay discounting among stimulant addicts. Biol Psychiatry 2011; 69:260–265Crossref, Medline, Google Scholar
175 : How can cognitive remediation therapy modulate brain activations in schizophrenia? An fMRI study. Psychiatry Res 2011; 192:160–166Crossref, Medline, Google Scholar
176 : Using neuroplasticity-based auditory training to improve verbal memory in schizophrenia. Am J Psychiatry 2009; 166:805–811Crossref, Medline, Google Scholar
177 : Is serum brain-derived neurotrophic factor a biomarker for cognitive enhancement in schizophrenia? Biol Psychiatry 2009; 66:549–553Crossref, Medline, Google Scholar
178 : Remediation of facial affect recognition impairments in patients with schizophrenia: a new training program. Psychiatry Res 2003; 117:281–284Crossref, Medline, Google Scholar
179 : Modification of affect perception deficits in schizophrenia. Schizophr Res 2000; 46:217–229Crossref, Medline, Google Scholar
180 : Treatment of cognitive dysfunctions and behavioral deficits in schizophrenia. Schizophr Bull 1992; 18:21–26Crossref, Medline, Google Scholar
181 : Cognitive enhancement therapy for schizophrenia: effects of a 2-year randomized trial on cognition and behavior. Arch Gen Psychiatry 2004; 61:866–876Crossref, Medline, Google Scholar
182 : Cognitive-Behavioral Treatment of Borderline Personality Disorder. New York, Guilford Press, 1993Google Scholar
183 : Problem solving and behavior modification. J Abnorm Psychol 1971; 78:107–126Crossref, Medline, Google Scholar
184 : Problem-solving therapy for depression: a meta-analysis. Clin Psychol Rev 2009; 29:348–353Crossref, Medline, Google Scholar