Increased P50 Gating but Intact Prepulse Inhibition in Borderline Personality Disorder
Neurobiological research suggests that a fronto-limbic network is related to borderline personality disorder symptoms, causing a weakening of prefrontal inhibitory control and amygdala hyperactivity. 2 Next to affective dysregulation, several research groups recently found evidence for cognitive deficits in borderline personality disorder. 3 , 4 In a recent meta-analysis, Ruocco 5 concluded that borderline patients generally perform more poorly than healthy comparison subjects on global dimensions of attention, cognitive flexibility, learning, and memory. But looking in more detail, the few neurocognitive studies undertaken have led to a broad but inconsistent pattern of impairments. While some authors report deficits in almost all classical neuropsychological tests, others found no differences between borderline patients and healthy comparison subjects. 6 – 8
The goal of the present study is to investigate if patients with borderline personality disorder show deficits in sensory gating, a normal preattentive inhibitory mechanism that prevents the brain from overflooding with sensory input. This research approach has led to deeper understanding of the underlying neural pathophysiology in schizophrenia. Decreased sensory gating in schizophrenia patients and their first degree relatives has been found repeatedly. 9 , 10
Two paradigms are widely used for establishing sensory gating: P50 gating and prepulse inhibition of the startle response (PPI). In P50 gating, auditory evoked potentials are measured in a double click paradigm, with a 500 msec interstimulus interval. Sensory gating occurs when the auditory evoked potentials amplitude elicited by the second click is suppressed when compared to the auditory evoked potentials waveform elicited by the first click. The inhibitory process can be detected after just 50 msec, suggesting that gating does not depend on any effortful control. Moreover, it is an automatic process, not controlled by sleep or attention. 11 , 12 The neural circuitry underlying P50 suppression is not precisely understood, but is known to involve hippocampal and frontal structures as well as cholinergic systems. 13 , 14 P50 gating abnormalities have been found in patients with schizophrenia and schizotypal personality disorder, 15 , 16 but also in psychotic patients with bipolar disorder and posttraumatic stress disorder. 12 , 17 , 18 Recently, decreased sensory gating in the mid-latency auditory evoked potentials N100 and P200 has also been found in patients with schizophrenia. 19
In the PPI paradigm, a motor startle reflex elicited by a loud noise is reduced when it is preceded by a weaker nonstartling stimulus, the prepulse. Animal and human studies suggest that the startle response is mediated by a neuronal circuit in the lower brainstem and that the startle response can be attenuated by several structures such as the prefrontal cortex. 20 , 21 A reduction of PPI, similar to P50 gating, has been demonstrated in patients with schizophrenia—and their siblings—and patients with schizotypal personality disorder. 9 , 22 In addition, effects of second generation antipsychotics on the PPI deficit have been found. 23 , 24
Although P50 gating, N100 gating, and PPI are conceptually linked, previous research suggests different underlying neural circuitries: concurrent assessment revealed no strong correlations in either humans or rats and there are important methodological differences. 14 , 25 – 27 Therefore, a combined use of the paradigms allows a broader range of inhibitory information processes to be investigated.
There are three reasons to examine sensory gating in borderline personality disorder. First, both borderline and schizophrenia spectrum patients share the vulnerability to develop psychotic symptoms, although these symptoms are transient, stress-related, and much less prominent in borderline personality disorder. In addition, clinical response on antipsychotics in borderline patients supports a relationship with psychotic disorders. 28 Second, animal research has revealed that early-life social events in rats, such as early handling, social isolation, and maternal deprivation, have important effects on PPI and P50 gating in adult life. 25 Since damaging early-life events are particularly common in borderline patients, 29 deficit inhibitory mechanisms could be a chain link in pathophysiology. Third, previous research using event-related potentials showed similar aberrations in schizophrenia and borderline personality disorder, 30 although the literature is limited. Event-related potentials research in borderline personality disorder particularly revealed lower amplitudes and longer latencies of the P300. 31 , 32 Drake et al. 33 found prolonged latencies and decreased amplitudes in mid-latency and long-latency auditory evoked potentials. Recently, diminished action monitoring investigated by measuring the error-related negativity was found by two different groups. 34 , 35
To date, only one study, by Herpertz and Koetting, 36 has addressed sensory motor gating in borderline personality disorder and did not find any differences in prepulse inhibition between borderline patients and comparison subjects. However, sensory (P50) gating in borderline personality disorder has not been investigated yet. We hypothesized that sensory gating and PPI would be reduced in borderline patients.
METHODS
Participants
Patients with the primary (DSM-IV) diagnosis borderline personality disorder were recruited from two Dutch psychiatry departments (inpatient clinic for personality disorders and outpatient clinic for dialectical behavior therapy). A trained physician (KG) or psychiatrist 1 confirmed the diagnostic criteria for borderline personality disorder using SCID-II. 37 A SCID-I screening 38 was performed to exclude current comorbid alcohol or substance dependence, axis I psychotic and mood disorders (except for dysthymic disorder), and neurological, ophthalmologic, and vestibular disorders. We recruited age-matched healthy comparison subjects who had no prior history of any major psychiatric or neurological disorders and who did not have first- or second-degree family members with psychotic disorders.
All subjects were instructed to abstain from coffee and nicotine 12 hours prior to testing to prevent confounding by the acute effects of these compounds. 39 , 40 In the week prior to testing, the use of cannabis or any other illicit drug or alcohol consumption exceeding more than three units per day was reason for exclusion as well. Further, on the morning of the experiment, no subjects had used any psychotropic drug.
The demographic data of the patients with borderline personality disorder and matched comparison subjects are shown in Table 1 . We performed two separate experiments, one on sensory gating and one on PPI, and applied the same inclusion and exclusion criteria to the participants. The participants gave written informed consent after complete description of the study and received financial compensation for their participation. The study was approved by the local ethics committee.
![]() |
Experiment 1: Sensory Gating
Recording Procedures
EEG recordings were made with Ag/AgCl electrodes mounted in an elastic electrode cap at the frontal region (Fz) and central region (Cz) (Rembrandt 5.2 acquisition software). For the detection of eye blinks and eye movement artifacts, horizontal electro-oculogram (EOG) recordings were placed at the outer canthus of each eye and vertical EOG recordings infraorbital and supraorbital to the left eye. All electrodes were referenced to the left mastoid electrode. A ground electrode was attached to the left temporal-parietal scalp. The impedance for the EEG electrodes was kept below 3.5 kΩ, while the impedance for the EOG and mastoid electrodes was kept below 5 kΩ. The electrical signals were recorded with band pass filters set at 0–70 Hz and digitized with a 204.8 Hz sample rate.
We seated each subject in a comfortable armchair and instructed him or her to stare at a fixed point. Auditory stimuli were presented through headphones and consisted of repeated presentation of two identical clicks: a conditioning stimulus (S1) and a test stimulus (S2) with a fixed interstimulus interval of 500 msec. The interval between the pairs of clicks varied randomly between 8 and 12 seconds. Each subject received 60 trials.
Auditory Evoked Potentials Analysis
The EEG and EOG signals were analyzed with Brain Vision Analyzer software. First, the raw signals were offline-referenced to linked ears. Artifact rejection was done by both visual inspection and semiautomatic rejection (amplitudes >100 μV; alpha theta activity). The data were baseline-corrected. To decrease the noise-to-signal ratio, the EEG signals were band-pass filtered between 10 and 50 Hz for examination of the P50 and between 1 and 40 for examination of the N100 and the P200. 19 The auditory evoked potentials waveforms had to meet the following criteria: the P50 was identified as the second major positive component between 30 and 80 msec poststimulation and is preceded by the photoacoustic wave in the 15 to 40 msec range. The N100 is the largest negative deflection between 80 and 150 msec, while the largest positive deflection between 150 and 250 msec is identified as the P200. Amplitudes of a waveform were measured from its peak to the preceding peak of opposite deflection (“peak-to-peak”).
Waveform measurements were made from both Cz and Fz, the channels where gating is known to be most pronounced. 41
Statistical Analysis
For each waveform (P50, N100, P200), analyses of variance (ANOVAs) were performed with the amplitudes of the P50, N100, and P200 as dependent variables, patient group as the between-subjects factor (borderline personality disorder, comparison subjects), and stimulus (S1, S2) and electrode position (Fz, Cz) as within-subjects factors.
In the literature, both difference scores (S1−S2) and suppression ratio scores (S2/S1) are used to measure sensory gating. 42 , 43 As previous research has shown that the difference score may be more reliable in repeated testing 42 and possibly more sensitive in detecting abnormalities, 19 we decided to report both scores but primarily discuss the difference scores. The difference score (S1−S2) of the amplitudes was analyzed with a paired t test. Differences in gating between borderline patients and comparison subjects were tested with ANOVAs, with the gating scores of the P50, N100, and P200 as dependent variables and electrode position as the within-subjects factor (Fz, Cz). The significance level was set at p<0.05 (two-tailed).
Experiment 2: Prepulse Inhibition of the Startle Response
Recording Procedures
Electrodes were placed beneath and at the site of the right eye, as well as a ground electrode on the forehead (impedance <5 kΩ). Subjects were seated in a comfortable armchair and instructed to stare at a fixed point while the auditory stimuli were presented through a headphone (MONACOR 2000). Electromyogram (EMG) activity was band-pass filtered (100–500 Hz) and recorded with sampling rate 2000 per second (Windaq Aquisiation Software, 50 Hz notch filter). After a 30 second acclimatization period that consisted of 50 dB continuous white noise, the session began with habituation trials of 15 (pulse alone) 40 msec pulses (106 dB) with random inter-stimulus intervals between 8 and 11 seconds.
Subsequently, the PPI part of the session consisted of 36 trials including six conditions in a pseudorandom order: 106 dB pulse alone, 90 dB pulse alone, 65 dB/90 dB prepulse-pulse trial, 75 dB/90 dB prepulse-pulse trial, 65 dB/106 dB prepulse-pulse trial, and 75 dB/106 dB prepulse-pulse trial (all with 100 msec prepulse-pulse interval).
All recordings were visually screened and onset time, peak latency, and peak amplitude were measured. Trials were rejected when the startle was a nonpolyphasic signal, had a peak amplitude <0.1875 microvolt, or had a peak onset latency time >95 msec. The filter window for response onset was 21–140 msec.
Statistical Analysis
An ANOVA was performed with amplitude of the startle reflex as the dependent variable and with prepulse levels (no prepulse, 65 dB, 75 dB) and pulse levels (90 dB, 106 dB) as within-subject factors. PPI was calculated with the formula: % PPI =100×[1− (amplitude on prepulse-pulse trials/amplitude on pulse-alone)]. Group differences (a between-subjects factor) for PPI were tested in a multivariate analysis of variance (MANOVA) with prepulse levels (65dB, 75 dB) and pulse levels (90 dB, 106 dB) as within-subject factors and percentage PPI as the dependent variable. The significance level was set at p<0.05 (two-tailed).
RESULTS
Sensory Gating
The grand averages of the auditory event-related potentials are presented in Figure 1 . The ANOVA with P50 amplitude as the dependent variable revealed significant main effects for electrode position (F=17.1, df=30, 1, p<0.001) and stimulus (F=38.6, df=30, 1, p<0.001). There was no main group effect (F=0.51, df=30, 1, p=0.48), but the significant group×stimulus interaction (F=4.60, df=30, 1, p<0.05) indicated different gating patterns between the groups. The group×electrode position was not significant (F=3.25, df=30, 1, p=0.08). As shown in Table 2 , further analysis for electrode position and amplitude indicated that the amplitudes of borderline patients were higher compared to healthy comparison subjects on S1, whereas there were no differences on S2.
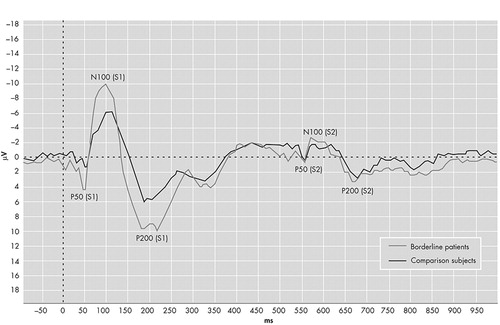
The borderline patients have significantly higher amplitudes on S1.
![]() |
The ANOVA for N100 and P200 revealed similar results. In both ANOVAs there were significant main effects for group (N100: F=4.84, df=30, 1, p<0.05; P200: F=6.86, df=30, 1, p<0.01) and stimulus (N100: F=163, df=30, 1, p<0.001; P200: F=111, df=30, 1, p<0.001). The main effect for electrode position was only significant for N100 (F=10.1, df=30, 1, p<0.01; P200: F=2.34, df=30, 1, p=0.14). Further analyses revealed the same pattern with higher amplitudes on S1 and equal amplitudes on S2. The data are presented in Table 2 .
The paired t tests revealed that gating occurred in both groups on all three waveforms in both Fz and Cz (borderline patients all waveforms: p<0.001; comparison subjects p<0.01 for P50, p<0.001 for N100 and P200). Additional tests revealed no statistical differences in latencies between the two groups ( Table 3 ).
![]() |
The results of our primary outcome measures, the P50, N100, and P200 gating scores, are shown in Table 2 . The ANOVA with P50 gating as dependent variable and electrode position as the within-subject factor revealed a significant group effect (F=4.60, df=30, 1, p<0.05) indicating that borderline patients had higher P50 gating than comparison subjects. The effect of electrode position was not significant (F=1.27, df=30, 1, p=0.27).
The separate ANOVAs with N100 gating and P200 gating as the dependent variables and electrode position as the within-subject factor revealed similar effects: there was a significant group effect (N100: F=5.03, df=30, 1, p<0.03; P200: F=6.86, df=30, 1, p<0.01) indicating that borderline patients also had higher mid-latency gating. The effect of electrode position was also significant (N100: F=4.35, df=30, 1, p<0.05; P200: F=110.6, df=30, 1, p<0.001).
We obtained essentially the same results while restricting the analyses to female patients and to patients without antipsychotic treatment. In addition, we performed additional analyses with ratio scores as dependent variables ( Table 2 ). Only the P50 ratio score on Fz was significantly different, with borderline patients showing more sensory gating.
Prepulse Inhibition of the Startle Response
The percentages PPI of the startle responses are displayed in Table 4 . A significant effect of the intensity of the pulse (F=71.32, df=1, 60, p<0.001) and intensity of the prepulse (F=51.82, df=2, 59, p<0.001) was found for the amplitude of the startle reflex, indicating that the intensity of both the prepulse and the pulse determined the amplitude of the startle response. There were no group differences in amplitudes (F=2.38, df=1, 60, p=0.13; Figure 2 ).
![]() |

Group differences were not statistically significant.
On the ANOVA with PPI as dependent variable, significant effects were found for the intensity of the prepulse (F=0.74.2, df=1, 59, p<0.001) and intensity of the pulse (F=9.54, df=1, 59, p<0.01). There was no group difference in PPI (F=0.69, df=1, 59, p=0.79). The results are presented in Table 3 .
The proportion of men to women differed significantly between borderline patients and comparison subjects ( Table 1 ) and since PPI exhibits gender differences, 44 additional tests were performed by excluding all male participants or setting sex as an extra between-subject factor. This additional analysis, however, revealed similar results for PPI to the whole sample. A rerun of these analyses that excluded all patients using antipsychotics also yielded similar results.
DISCUSSION
We conducted two experiments to investigate sensory (motor) gating in patients with borderline personality disorder and comparison subjects.
In the auditory evoked potentials experiment we expected to find a gating deficit such as described in schizophrenia. In contrast to our hypothesis, we found more gating of the P50, N100, and P200. The difference is caused by significantly higher S1 amplitudes in the borderline personality disorder group compared to the comparison group, rather than differences in S2 amplitudes.
The N100 and P200 waves are thought to reflect the allocation of early attention to a stimulus, whereas the P50 is thought to be “preattentive.” 11 , 14 In addition, gating of P50, N100, and P200 reflect different mechanisms and are only partially correlated. 45 The data suggest that borderline patients differ from comparison subjects in both early attentive as well as preattentive information processing and do not exhibit the same deficits as patients with schizophrenia.
The increased amplitudes in the early auditory evoked potentials waves on S1 may indicate that borderline patients have a higher response tendency to new auditory stimuli than comparison subjects. 46 It is generally accepted that the amount of synchronization of firing pyramidal cells is the main contributor to the amplitude of a cortical recorded evoked potential. The number of cells that fire depends on the firing mode of cortical pyramidal neurons. These cells fire either in the tonic or bursting mode. Generally, tonic firing is typical for alert wakefulness; burst firing is characteristic of drowsiness. It is easier to recruit a larger number of cells when cells are in the bursting mode than in the tonic firing mode. 47 Therefore, the larger amplitude may be due to more bursting cells, and perhaps to a reduced arousal level.
Elevated mid-latency event-related potentials have also been found in subjects with sensation-seeking and thrill-seeking behavior, characteristics that are common in borderline patients. 48 , 49 Our data may therefore support the current hypotheses on impulsivity in borderline personality disorder. 50 In line with this, we also found impulsive response style in a Flankers task and saccadic eye movements in a similar patient sample. 34 , 51 The present data suggest that borderline patients have an increased physiological predisposition to respond to new stimuli. However, the “compensatory” gating mechanism, which prevents the individual from being flooded by sensory input, is intact in borderline patients. In fact, the present data suggest that it might be even more efficient than in comparison subjects.
There are two possible methodological limitations to the auditory evoked potentials experiment. First, there may be a type II error due to the small sample size, which in some cases prevented us from demonstrating genuine significance effects instead of tendencies (e.g., the N100 at Fz). In addition, the number of trials was relatively small. Second, the paradigm we used was specifically designed for early preattentive sensory gating and not the “odd-ball” paradigm, in which subjects need to respond to target stimuli that occur infrequently and irregularly within a series of standard stimuli. 52 Therefore, a relation between the present increased neuronal reaction to new sensory stimuli and attention deficits should be drawn with caution.
The second experiment replicated previous work by Herpertz and Koetting 36 and showed that there is no evidence for abnormalities in PPI of the startle response in patients with borderline personality disorder. Their patient sample did not have similar inclusion criteria to ours; our patients had no comorbid major depression and had abstained from nicotine, whereas their patients were unmedicated.
Taking both experiments together, it is important to address two methodological issues. First, we included patients without comorbid psychotic or depressive disorder and the data were not corrupted by the acute effects of medication, illicit drugs, or nicotine. However, some of the patients used psychotropic medication, but not on the day of the study. In post hoc analyses we found no differences between medicated and nonmedicated patients. Second, one should take into account that our participants were predominantly female. Since women are known to exhibit less PPI and P50 gating than men, it needs to be established whether increased P50 gating and amplitudes of P50, N100, and P200 might be present in male borderline personality disorder patients as well. 53 , 54
In conclusion, patients with borderline personality disorder do not show sensory (motor) gating deficits such as those described in other major psychiatric disorders such as schizophrenia and schizotypal personality disorder. In contrast, our data showed borderline patients to have even higher amplitudes on S1 than comparison subjects, which may suggest differences in early preattentive information processes. The latter finding merits further exploration in larger patient samples in order to demonstrate if this is specific for borderline personality disorder pathology.
1 . Skodol AE, Gunderson JG, Pfohl B, et al: The borderline diagnosis, I: psychopathology, comorbidity, and personality structure. Biol Psychiatry 2002; 51:936–950Google Scholar
2 . Lieb K, Zanarini MC, Schmahl C, et al: Borderline personality disorder. Lancet 2004; 364:453–461Google Scholar
3 . Nigg JT, Silk KR, Stavro G, et al: Disinhibition and borderline personality disorder. Dev Psychopathol 2005; 17:1129–1149Google Scholar
4 . LeGris J, van Reekum R: The neuropsychological correlates of borderline personality disorder and suicidal behaviour. Can J Psychiatry 2006; 51:131–142Google Scholar
5 . Ruocco AC: The neuropsychology of borderline personality disorder: a meta-analysis and review. Psychiatry Res 2005; 137:191–202Google Scholar
6 . Cornelius JR, Soloff PH, George AWA, et al: An evaluation of the significance of selected neuropsychiatric abnormalities in the etiology of borderline personality disorders. J Personal Disord 1989; 3:19–25Google Scholar
7 . Sprock J, Rader TJ, Kendall JP, et al: Neuropsychological functioning in patients with borderline personality disorder. J Clin Psychol 2000; 56:1587–1600Google Scholar
8 . Kunert HJ, Druecke HW, Sass H, et al: Frontal lobe dysfunctions in borderline personality disorder? Neuropsychological findings. J Personal Disord 2003; 17:497–509Google Scholar
9 . Braff DL, Geyer MA, Swerdlow NR: Human studies of prepulse inhibition of startle: normal subjects, patient groups, and pharmacological studies. Psychopharmacology (Berl) 2001; 156:234–258Google Scholar
10 . Adler LE, Freedman R, Ross RG, et al: Elementary phenotypes in the neurobiological and genetic study of schizophrenia. Biol Psychiatry 1999; 46:8–18Google Scholar
11 . Kho KH, Verkes RJ, Eling P, et al: P50 gating is not affected by selective attention. J Psychophysiology 2003; 17:23–29Google Scholar
12 . Braff DL, Light GA: Preattentional and attentional cognitive deficits as targets for treating schizophrenia. Psychopharmacology (Berl) 2004; 174:75–85Google Scholar
13 . Adler LE, Olincy A, Waldo M, et al: Schizophrenia, sensory gating, and nicotinic receptors. Schizophr Bull 1998; 24:189–202Google Scholar
14 . Oranje B, Geyer MA, Bocker KB, et al: Prepulse inhibition and P50 suppression: commonalities and dissociations. Psychiatry Res 2006; 143:147–158Google Scholar
15 . Croft RJ, Lee A, Bertolot J, Gruzelier JH: Associations of P50 suppression and desensitization with perceptual and cognitive features of “unreality” in schizotypy. Biol Psychiatry 2001; 50:441–446Google Scholar
16 . Cadenhead KS, Light GA, Geyer MA, et al: Sensory gating deficits assessed by the P50 event-related potential in subjects with schizotypal personality disorder. Am J Psychiatry 2000; 157:55–59Google Scholar
17 . Olincy A, Martin L: Diminished suppression of the P50 auditory evoked potential in bipolar disorder subjects with a history of psychosis. Am J Psychiatry 2005; 162:43–49Google Scholar
18 . Karl A, Malta LS, Maercker A: Meta-analytic review of event-related potential studies in post-traumatic stress disorder. Biol Psychol 2006; 71:123–147Google Scholar
19 . Boutros NN, Korzyukov O, Jansen B, et al: Sensory gating deficits during the mid-latency phase of information processing in medicated schizophrenia patients. Psychiatry Res 2004; 126:203–215Google Scholar
20 . Koch M: The neurobiology of startle. Prog Neurobiol 1999; 59:107–128Google Scholar
21 . Swerdlow NR, Geyer MA, Braff DL: Neural circuit regulation of prepulse inhibition of startle in the rat: current knowledge and future challenges. Psychopharmacology (Berl) 2001; 156:194–215Google Scholar
22 . Hazlett EA, Levine J, Buchsbaum MS, et al: Deficient attentional modulation of the startle response in patients with schizotypal personality disorder. Am J Psychiatry 2003; 160:1621–1626Google Scholar
23 . Kumari V, Sharma T: Effects of typical and atypical antipsychotics on prepulse inhibition in schizophrenia: a critical evaluation of current evidence and directions for future research. Psychopharmacology (Berl) 2002; 162:97–101Google Scholar
24 . Geyer MA, Krebs-Thomson K, Braff DL, et al: Pharmacological studies of prepulse inhibition models of sensorimotor gating deficits in schizophrenia: a decade in review. Psychopharmacology (Berl) 2001; 156:117–154Google Scholar
25 . Ellenbroek BA: Pre-attentive processing and schizophrenia: animal studies. Psychopharmacology (Berl) 2004; 174:65–74Google Scholar
26 . Budd TW, Barry RJ, Gordon E, et al: Decrement of the N1 auditory event-related potential with stimulus repetition: habituation vs. refractoriness. Int J Psychophysiol 1998; 31:51–68Google Scholar
27 . van Luijtelaar EL, Miller CA, Coenen AM, et al: Differential effects of non-REM and REM sleep on sensory gating in rats. Acta Neurobiol Exp (Wars) 1998; 58:263–270Google Scholar
28 . Grootens KP, Verkes RJ: Emerging evidence for the use of atypical antipsychotics in borderline personality disorder. Pharmacopsychiatry 2005; 38:20–23Google Scholar
29 . Battle CL, Shea MT, Johnson DM, et al: Childhood maltreatment associated with adult personality disorders: findings from the collaborative longitudinal personality disorders study. J Personal Disord 2004; 18:193–211Google Scholar
30 . Boutros NN, Torello M, McGlashan TH: Electrophysiological aberrations in borderline personality disorder: state of the evidence. J Neuropsychiatry Clin Neurosci 2003; 15:145–154Google Scholar
31 . Blackwood DH, St-Clair DM, Kutcher SP: P300 event-related potential abnormalities in borderline personality disorder. Biol Psychiatry 1986; 21:560–564Google Scholar
32 . Kutcher SP, Blackwood DH, St-Clair D, et al: Auditory P300 in borderline personality disorder and schizophrenia. Arch Gen Psychiatry 1987; 44:645–650Google Scholar
33 . Drake ME, Phillips BB, Pakalnis A: Auditory evoked potentials in borderline personality disorder. Clin Electroencephalogr 1991; 22:188–192Google Scholar
34 . de Bruijn ER, Grootens KP, Verkes RJ, et al: Neural correlates of impulsive responding in borderline personality disorder: ERP evidence for reduced action monitoring. J Psychiatr Res 2006; 40:428–437Google Scholar
35 . Ruchsow M, Walter H, Buchheim A, et al: Electrophysiological correlates of error processing in borderline personality disorder. Biol Psychol 2006; 72:133–140Google Scholar
36 . Herpertz SC, Koetting K: Startle response in inpatients with borderline personality disorder vs. healthy controls. J Neural Transm 2005; 112:1097–1106Google Scholar
37 . First MB, Spitzer RL, Gibbon M, et al: Structured Clinical Interview for DSM-IV Axis II Personality Disorders (SCID-II). Washington, DC, American Psychiatric Press, 1997Google Scholar
38 . Spitzer RL, Williams JB, Gibbon M, et al: The Structured Clinical Interview for DSM-III-R (SCID), I: history, rationale, and description. Arch Gen Psychiatry 1992; 49:624–629Google Scholar
39 . Croft RJ, Dimoska A, Gonsalvez CJ, et al: Suppression of P50 evoked potential component, schizotypal beliefs and smoking. Psychiatry Res 2004; 128:53–62Google Scholar
40 . Adler LE, Hoffer LD, Wiser A, et al: Normalization of auditory physiology by cigarette smoking in schizophrenic patients. Am J Psychiatry 1993; 150:1856–1861Google Scholar
41 . Muller MM, Keil A, Kissler J, et al: Suppression of the auditory middle-latency response and evoked gamma-band response in a paired-click paradigm. Exp Brain Res 2001; 136:474–479Google Scholar
42 . Smith DA, Boutros NN, Schwarzkopf SB: Reliability of P50 auditory event-related potential indices of sensory gating. Psychophysiology 1994; 31:495–502Google Scholar
43 . Potter D, Summerfelt A, Gold J, et al: Review of clinical correlates of P50 sensory gating abnormalities in patients with schizophrenia. Schizophr Bull 2006; 32:692–700Google Scholar
44 . Aasen I, Kolli L, Kumari V: Sex effects in prepulse inhibition and facilitation of the acoustic startle response: implications for pharmacological and treatment studies. J Psychopharmacol 2005; 19:39–45Google Scholar
45 . Oranje B, van Berckel BN, Kemner C, et al: P50 suppression and prepulse inhibition of the startle reflex in humans: a correlational study. Biol Psychiatry 1999; 45:883–890Google Scholar
46 . Coull JT: Neural correlates of attention and arousal: insights from electrophysiology, functional neuroimaging and psychopharmacology. Prog Neurobiol 1998; 55:343–361Google Scholar
47 . Coenen AM: Neuronal activities underlying the electroencephalogram and evoked potentials of sleeping and waking: implications for information processing. Neurosci Biobehav Rev 1995; 19:447–463Google Scholar
48 . Wang W, Wang YH: Sensation seeking correlates of passive auditory P3 to a single stimulus. Neuropsychologia 2001; 39:1188–1193Google Scholar
49 . Pierson A, Le HJ, Fossaert A, et al: Frontal reactivity and sensation seeking an ERP study in skydivers. Prog Neuropsychopharmacol Biol Psychiatry 1999; 23:447–463Google Scholar
50 . Skodol AE, Siever LJ, Livesley WJ, et al: The borderline diagnosis, II: biology, genetics, and clinical course. Biol Psychiatry 2002; 51:951–963Google Scholar
51 . Grootens KP, van Luijtelaar G, Buitelaar J, et al: Inhibition errors in borderline personality disorder with psychotic-like symptoms: implications for a prefrontal cortex dysfunction hypothesis. Prog Neuropsychopharmacol Biol Psychiatry 2008; 32:267–73Google Scholar
52 . Huettel SA, McCarthy G: What is odd in the oddball task? Prefrontal cortex is activated by dynamic changes in response strategy. Neuropsychologia 2004; 42:379–386Google Scholar
53 . Swerdlow NR, Auerbach P, Monroe SM, et al: Men are more inhibited than women by weak prepulses. Biol Psychiatry 1993; 34:253–260Google Scholar
54 . Hetrick WP, Sandman CA, Bunney WE Jr, et al: Gender differences in gating of the auditory evoked potential in normal subjects. Biol Psychiatry 1996; 39:51–58Google Scholar