Saccadic Impairment Associated With Remote History of Mild Traumatic Brain Injury
Abstract
Evidence suggests that mild traumatic brain injury (TBI) is associated with long-term changes in brain function, but conventional neurocognitive tools are often insensitive to deficits after 90 days. Eye movements have been proposed as a means to identify more chronic forms of impairment. In this study, saccadic, manual, and conventional neuropsychological measures were compared between participants with remote mild TBI and well-matched control participants. Saccadic impairment was more frequent within the mild TBI group, and a history of multiple injuries or high symptom burden appeared to compound this risk. However, other neurocognitive measures did not differ by group, number of injuries, or symptom severity. These results suggest that saccadic impairment may reflect chronic effects of mild TBI that conventional measures are unable to detect.
Although computerized neurocognitive assessment tools (NCATs) may be useful for identifying cognitive impairments in the acute stage after mild traumatic brain injury (TBI),1 the sensitivity of existing NCATs is greatly diminished within 7–10 days.2–4 More comprehensive forms of neuropsychological evaluation may retain sensitivity to impairment up to 90 days after mild TBI, after which neuropsychological performance is expected to return to baseline.5,6 Normalization of impairments on neuropsychological testing during this time frame may be interpreted to reflect “full recovery” after mild TBI. However, laboratory findings such as axonal injury and altered neural activation have been shown to extend significantly beyond the 90-day time frame,7–10 and some individuals sustaining a mild TBI may also report clinically significant neurobehavioral symptoms into the chronic phase.10–12 In addition, a history of head injury may increase the risk for the development of late-life cognitive decline or dementia.13–15 In sum, current measures may overlook important forms of chronic morbidity. Therefore, novel approaches are needed to identify residual impairments associated with a history of mild TBI.
Examination of eye movements may provide a means to accomplish this goal. Eye movement abnormalities have been identified in a number of neurological conditions, including mild TBI.16–19 Potential mechanisms of impaired eye movement in mild TBI include injuries to midbrain structures involved in basic oculomotor control, as well as diffuse axonal injuries impacting frontoparietal circuits involved in attention.20,21 One study conducted in the acute phase of mild TBI found that concussed individuals had normal oculomotor performance on basic (reflexive) saccades and oculomotor smooth pursuit, but they exhibited deficits on more challenging or cognitively loaded saccadic tasks, such as self-paced saccades, antisaccades, and memory-guided saccades.22 Concussed participants also demonstrated a pattern of increased task-related activation on fMRI suggestive of reduced processing efficiency for these brain functions. These acute oculomotor deficits improved across time; however, some abnormalities in more complex saccadic eye movements persisted at 30 days postinjury, along with increased task-related brain activation.23 Evidence has also been found for impairment in saccades and smooth pursuit eye movements in more chronic mild TBI (i.e., ≥3 months postinjury)18,24–26 and among individuals with persistent postconcussive symptoms in comparison to those with good recovery after mild TBI.19 Oculomotor impairment in these studies has been linked to indicators of white matter integrity on diffusion tensor imaging (DTI)18 and abnormal beta activity on magnetoencephalography in the parietal, frontal, and temporal areas and in the caudate.25
Each of these studies suggests that, beyond assessment of basic oculomotor functions, eye movements may also provide advantages as a modality for the assessment of higher-level visual attention. The neural circuitry underlying visual attention overlaps considerably with networks involved in programming eye movements.27–31 Recent research conducted in our laboratory has demonstrated that attention processes such as tonic vigilance, phasic alerting, performance consistency, response inhibition, and attention control may be reliably assessed using saccadic responses modulated by preparatory cues.32 Furthermore, this work suggests that these saccadic measures are able to capture different aspects of attention processing than conventional approaches to assessment. However, the impact of chronic mild TBI on these measures has not yet been examined. We conducted this study to examine the impact of remote mild TBI on saccadic attention metrics. We hypothesized that a history of remote mild TBI would be more strongly associated with saccadic impairment than with impairment on conventional neuropsychological measures.
Methods
Participants
Participants with mild TBI and healthy control participants were recruited using advertisements in medical and research facilities and public areas within the Washington, D.C., metropolitan area. Participation took place in a nonclinical laboratory setting, and participants were informed that data would be used for research purposes only. The mild TBI group consisted of adults (≥18 years old) with a history of one or more mild TBIs, defined according to ACRM criteria as a head injury accompanied by loss of consciousness (LOC) ≤30 minutes in duration and/or posttraumatic amnesia (PTA) ≤24 hours in duration.33 The control group consisted of adults with a negative history of TBI or other neurological conditions. Information about head injury severity and characteristics was obtained through the use of a detailed, custom-designed structured interview and verified by a consensus team including two licensed providers with postdoctoral training in assessment of brain injury. The structured interview included questions about medical history as well as detailed questions regarding circumstances and mechanism of injury, length of LOC, PTA, and other alterations of consciousness.
We examined 110 individuals for eligibility. Exclusion criteria consisted of a positive history of moderate to severe TBI (mild TBI: N=12), the presence of other medical/neurological conditions expected to affect cognitive function (control: N=5, mild TBI: N=2), presence of uncorrected visual impairment (N=0), difficulty identifying test stimuli (N=0), failure on two or more measures of response validity/effort (control: N=2), and failure to follow task instructions (mild TBI: N=1, control: N=2). Individuals with a history of head injury without LOC or PTA were also excluded (mild TBI: N=3, control: N=1) in order to minimize ambiguity in distinguishing signs of neurotrauma from other injury-related factors. In addition, one individual from the mild TBI group was excluded due to technical difficulties preventing acquisition of eye movement data.
Procedures
All research methods and procedures were approved by the Institutional Review Board at the Uniformed Services University of the Health Sciences in Bethesda, MD. Assessment procedures were supervised by a licensed clinical neuropsychologist. After providing written informed consent, participants completed a comprehensive structured interview, the Bethesda Eye & Attention Measure (BEAM) v.34,32 the Neurobehavioral Symptom Inventory (NSI),34 and a fixed battery of neuropsychological measures.
Measures
BEAM.
A detailed description of BEAM has been provided previously.32 In brief, BEAM is a computerized tool for the assessment of visual attention and neuromotor performance using saccadic and manual (button-press) responses to target stimuli. BEAM evaluates examinees’ ability to efficiently use preparatory attention cues in response to visual targets. As depicted in Figure 1, the BEAM configuration used for this study included a remote (contactless) eye tracker positioned 24 inches from the examinee beneath an LCD monitor, with a response pad used to collect manual responses. Examinees are instructed, “At the beginning of each trial, look at the cross in the center of the screen. A target circle will appear above, below, left, or right of the cross. When the target circle appears, look at it and press the button as fast as you can. However, if you see a red arrow, do not look at the target circle and do not press the button.” For each of 24 practice trials and 192 test trials, circular targets are presented 7.5° above, below, to the left, or to the right of the center of the screen. On each trial, the target is preceded immediately by one of six possible cues designed to elicit preparatory attention processing. An example trial is shown in Figure 2. “Nondirectional” cues (white diamonds) provide temporal information about the imminent appearance of a target, whereas “Directional” cues (white arrows pointing toward the impending target) provide valid spatial information. “Misdirectional” cues (white arrows pointing away from the impending target) provide invalid spatial information, requiring resolution of attentional interference in order to reorient to the correct target location. In “Uncued” trials, the central fixation cross persists through the appearance of the target. “Gap” cues (a blank image replacing the fixation cross) serve to disengage attention prior to the appearance of the target. “No-go” cues (red arrows pointing toward the impending target) provide a signal that the participant should not respond to that target. Different cue types are intermixed across test trials in an unpredictable manner. Completion of BEAM (including instructions and calibration) is typically completed in less than 15 minutes. No-go trials register saccadic and manual inhibition errors (i.e., when an examinee fails to withhold a response), whereas nondirectional, directional, misdirectional, gap, and uncued trials measure saccadic and manual response time (RT). Saccadic and manual RT median (latency) and variability (intraindividual standard deviation of RTs) are then aggregated for each trial type. In total, BEAM saccadic and manual performance are each represented by five RT latency scores, five RT variability scores, and one inhibition error score.
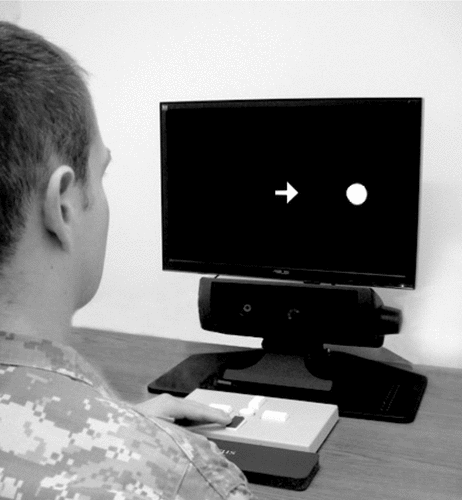
FIGURE 1. Bethesda Eye & Attention Measure v.34 (BEAM) Prototype System With Simulated Examineea
a Eye movements are recorded using a remote eye tracker positioned beneath the monitor. Manual responses are recorded using a response pad.
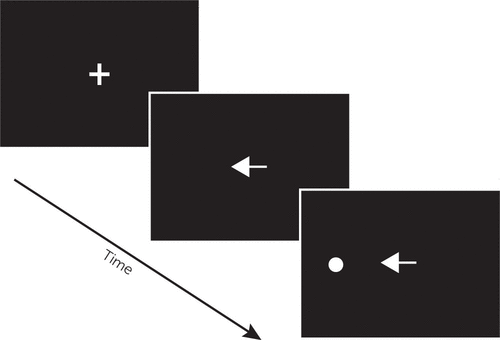
FIGURE 2. Bethesda Eye & Attention Measure v.34 (BEAM) Example Triala
a Directional cue is shown. Examinees are instructed to look at the circular target and press the button as quickly as possible. Stimuli presented here are not to scale.
NSI.
The NSI34 is a widely used, 22-item self-report questionnaire that assesses the postconcussive symptoms as defined by the ICD-10. Participants are asked to rate the severity of each affective, cognitive, somatic, or sensory symptom on a 5-point scale (“None” to “Very Severe”). Participants scoring ≥16 points on the NSI were classified into a “High NSI” subgroup indicative of clinically significant levels of symptoms; all others were classified as “Low NSI.”
Neuropsychological evaluation.
A battery of standardized neuropsychological tests was administered, including measures of attention, information processing speed, motor performance, learning/memory, and executive functions. Premorbid verbal intelligence was estimated using the Wechsler Test of Adult Reading35 (WTAR). Neuropsychological measures and variables included the Wechsler Adult Intelligence Scale IV36,37 (WAIS-IV Digit Span Forward, Digit Span Backward, Symbol Search Total Score), Conners’ Continuous Performance Test–II38 (CPT-II Hit RT, Hit RT SE, Omissions, and Commissions), the Delis-Kaplan Executive Function System39 (Color Word Interference Test Trials 1, 2, 3, and 4 time), Trail Making Test40 (TMT Parts A and B time), Grooved Pegboard41 (Dominant and Nondominant Hand Score), and the California Verbal Learning Test–II42 (CVLT-II Trial 1–5 Total, Short Delay Free Recall, Long Delay Free Recall). In total, the conventional neuropsychological battery included 18 metrics. In addition, embedded metrics from the WAIS-IV Digit Span, CPT-II, TMT, and CVLT-II were used to evaluate performance validity (i.e., test-taking effort) using previously validated cutoffs for each measure.42,43
Data Analysis
The SPSS 22.0 statistical package was used for all data analyses. Descriptive statistics were conducted on all variables to summarize the data. Each of the BEAM RT latency, RT variability, and inhibition error scores were standardized to the overall performance of the control group as Z scores, with better performance yielding higher scores. Raw scores from the conventional neuropsychological battery were converted to Z scores based on published normative data, with better performance yielding higher scores.
On the basis of previous research regarding the heterogeneity of mild TBI as a clinical condition, we expected that individual participants may manifest impairment in somewhat different ways.21,44 In these situations, group averages on individual test metrics may mask heterogeneous forms of impairment across tests. Therefore, similar to methods used previously for neuropsychological and eye movement data,19,45 an unweighted algorithm was applied to identify impairment across each test category. Participants’ performance on BEAM saccadic measures, BEAM manual measures, or the conventional neuropsychological battery was flagged as “impaired” if they obtained one or more scores ≥2 SD below the mean of the control group or the normative sample. This approach was intended to distill straightforward, clinically relevant findings from a large number of test metrics, while minimizing the risk for spurious results (i.e., family-wise type I error).
Data were complete for all primary variables within the analytic sample. Binary logistic regression models were used to evaluate the potential impact of mild TBI history on the risk for impairment. Additional binary logistic regression models were examined including participant age, estimated premorbid verbal intelligence, or NSI total score to account for the potential influence of these factors on primary results. Potential impacts of time since injury were evaluated using Spearman correlations. Impairment indexes were compared across number of mild TBIs (0, 1, or 2+) using the gamma statistic to explore potentially cumulative impacts of injury. Impairment indexes were also compared across NSI subgroups (Control/Low NSI, Control/High NSI, Mild TBI/Low NSI, and Mild TBI/High NSI) using the likelihood ratio to examine correspondence of neural impairment with severity of self-reported neurobehavioral symptoms. Planned post hoc analyses were conducted using independent-samples t tests and Kolmogorov-Smirnov tests to compare individual BEAM and neuropsychological metrics across the control group and mild TBI group.
Results
Sample Characteristics
Participant characteristics are presented in Table 1. The final sample included 54 individuals with no history of TBI and 27 individuals with a history of mild TBI sustained a median of 6.91 years (interquartile range=2.75–21.86) previously. Only one participant had been involved in litigation related to their injury. Participants in the mild TBI group reported significantly greater NSI symptoms (M=13.70, SD=11.89) than those in the control group (M=7.80, SD=8.63), p=0.03. However, the mild TBI group and the control group did not differ significantly in age, education, gender, ethnicity, estimated premorbid verbal intelligence, or performance on the conventional neuropsychological battery. The majority of individuals in the mild TBI group (N=20, 74.07%) sustained a single mild TBI, whereas 7 (25.93%) sustained two or more mild TBIs (M=2.86, SD=1.22). Primary injury mechanisms included sports/recreation (40.7%), accidental fall (25.9%), motor vehicle accidents (22.2%), assault (3.7%), explosive blast (3.7%), and other blunt trauma (3.7%).
Control Group (N=54) | Mild TBI Group (N=27) | pa | |
---|---|---|---|
Female, # (%) | 29 (53.7%) | 16 (59.3%) | 0.64 |
Age in years, M (SD) | 33.17 (11.42) | 34.93 (12.59) | 0.53 |
Years of education, M (SD) | 16.20 (2.63) | 16.04 (2.16) | 0.78 |
Estimated premorbid IQ, M (SD) | 108.59 (11.23) | 110.15 (9.21) | 0.54 |
Global cognitive T score, M (SD) | 52.34 (4.56) | 53.23 (4.87) | 0.42 |
Neurobehavioral Symptom Inventory score, M (SD) | 7.80 (8.63) | 13.70 (11.89) | 0.03 |
Race/ethnicity | 0.83 | ||
White, # (%) | 30 (55.6) | 18 (66.7) | |
Hispanic/Latino, # (%) | 3 (5.6) | 2 (7.4) | |
Asian, # (%) | 4 (7.4) | 1 (3.7) | |
Black, # (%) | 15 (27.8) | 5 (18.5) | |
Other, # (%) | 2 (3.7) | 1 (3.7) |
TABLE 1. Participant Characteristics: Control and Mild TBI Groups
Impairment Associated With History of Mild TBI
Binary logistic regression models demonstrated that a positive history of mild TBI was associated with a greater risk for BEAM saccadic impairment (Wald=5.81, OR=3.52, p=0.02), but not for BEAM manual impairment (Wald=0.04, OR=1.12, p=0.85) or impairment on the conventional neuropsychological evaluation (Wald=1.65, OR=0.35, p=0.20). Saccadic impairment was present for a total of 18.5% of participants in the control group and 44.4% of participants in the mild TBI group, compared with 20.4% of control participants and 22.2% of mild TBI participants with manual impairment, and 18.5% of control participants and 7.4% of mild TBI participants with one or more impaired scores on the neuropsychological battery. Inclusion of NSI total score, participant age, or estimated premorbid verbal intelligence as predictors did not affect the significance of a history of mild TBI in any models. In addition, time since injury was not significantly associated with BEAM saccadic impairment (rs=0.30, p=0.13), BEAM manual impairment (rs=0.07, p=0.73), or impairment on the neuropsychological battery (rs=–0.25, p=0.20).
Greater number of mild TBIs was significantly associated with higher rates of BEAM saccadic impairment (gamma=0.57, T=2.52, p=0.01, see Figure 3). Participants with two or more mild TBIs demonstrated the highest rate of impairment (71.4%) compared with 35.0% of those with a single mild TBI and 18.5% of control participants. Rates of BEAM manual impairment (gamma=0.07, T=0.27, p=0.79) and impairment on the conventional neuropsychological battery (gamma=−0.41, T=–1.31, p=0.19) did not differ significantly by the number of mild TBIs.
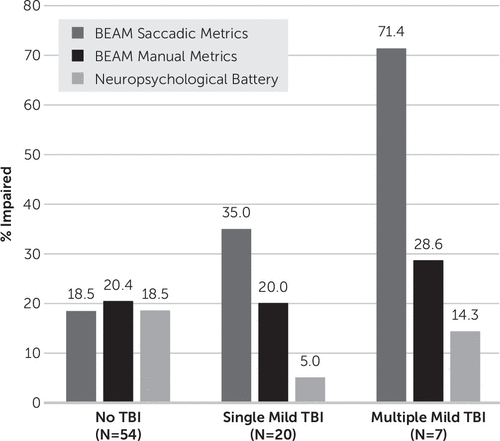
FIGURE 3. Frequency of Impairment by Number of Mild Traumatic Brain Injuries (TBIs)a
a Impairment was defined as one or more impaired scores within the metric. BEAM saccadic and manual impairment each include 12 component scores. The Neuropsychological Battery includes 20 component scores. Greater number of mild TBIs was significantly associated with higher rates of BEAM saccadic impairment, gamma=0.57, T=2.52, p=0.01.
NSI subgroup was also significantly associated with rates of BEAM saccadic impairment, LR(3)=10.02, p=0.02 (Figure 4). The “Mild TBI/High NSI” subgroup demonstrated the highest rate of impairment (66.7%), compared with 33.3% in the “Mild TBI/Low NSI” and “Control/High NSI” subgroups and 15.6% in the “Control/Low NSI” subgroup. Rates of BEAM manual impairment (LR(3)=4.73, p=0.16) and impairment on the conventional neuropsychological battery (LR(3)=2.03, p=0.57) did not significantly differ by NSI subgroup. It is important to note that rates of BEAM saccadic impairment were similar among participants with a single mild TBI and low NSI (23.1%), as compared with the full control group (18.5%) and the subgroup of control participants with low NSI (15.6%).
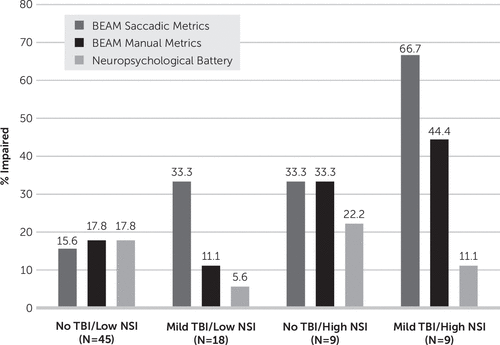
FIGURE 4. Frequency of Impairment by History of Traumatic Brain Injury (TBI) and Neurobehavioral Symptomsa
a Impairment was defined as one or more impaired scores within the metric. BEAM saccadic and manual impairment each include 12 component scores. The Neuropsychological Battery includes 20 component scores. NSI subgroup was significantly associated with rates of BEAM saccadic impairment, LR(3)=10.02, p=0.02.
Planned post hoc analyses demonstrated that the mild TBI group demonstrated less consistency in saccadic RT (i.e., more variability) than the control group in response to misdirectional cues (t79=–3.15, p=0.002). Mean performance of the control group and the mild TBI group did not differ significantly on any other individual metrics from BEAM or the neuropsychological battery (Table 2 and Table 3). However, patterns of impairment (i.e., frequency of scores ≥2 SD below the comparison group) differed by test category. On average, the mild TBI group was impaired 3.37 times more frequently than the control group on individual BEAM saccadic metrics, 0.86 times more frequently on individual BEAM manual metrics, and 1.06 times more frequently on individual metrics from the conventional neuropsychological battery.
Control Group (N=54) | Mild TBI Group (N=27) | pb | Impairment Ratioc | |||||
---|---|---|---|---|---|---|---|---|
M | SD | % Impaireda | M | SD | % Impaireda | |||
Saccadic RT latency (ms) | ||||||||
Directional cue | 266.47 | 39.33 | 3.70% | 280.94 | 44.60 | 3.70% | 0.14 | 1.00 |
Nondirectional cue | 323.67 | 43.31 | 3.70% | 328.15 | 43.68 | 3.70% | 0.66 | 1.00 |
Misdirectional cue | 349.57 | 48.21 | 1.85% | 370.76 | 49.50 | 11.11% | 0.07 | 6.00 |
Gap cue | 304.56 | 37.13 | 0.00% | 307.06 | 42.16 | 7.41% | 0.79 | 7.41 |
Uncued | 379.84 | 61.23 | 1.85% | 398.81 | 59.03 | 3.70% | 0.19 | 2.00 |
Saccadic RT variabilityd | ||||||||
Directional cue | 87.23 | 31.43 | 5.56% | 90.40 | 24.69 | 3.70% | 0.65 | 0.67 |
Nondirectional cue | 79.95 | 30.00 | 1.85% | 89.02 | 37.42 | 11.11% | 0.24 | 6.00 |
Misdirectional cue | 85.05 | 30.36 | 1.85% | 107.87 | 31.48 | 11.11% | <0.01 | 6.00 |
Gap cue | 84.78 | 28.04 | 1.85% | 89.26 | 28.77 | 3.70% | 0.50 | 2.00 |
Uncued | 108.46 | 27.21 | 3.70% | 119.10 | 35.09 | 11.11% | 0.14 | 3.00 |
Saccadic inhibition errors† | 13.69 | 13.14 | 5.56% | 16.70 | 16.37 | 11.11% | 0.70 | 2.00 |
Manual RT latency (ms) | ||||||||
Directional cue | 455.31 | 104.86 | 5.56% | 420.46 | 124.34 | 7.41% | 0.19 | 1.33 |
Nondirectional cue | 493.11 | 97.12 | 5.56% | 469.02 | 124.67 | 7.41% | 0.34 | 1.33 |
Misdirectional cue | 528.23 | 103.70 | 5.56% | 483.44 | 132.76 | 3.70% | 0.10 | 0.67 |
Gap cue | 534.19 | 92.24 | 3.70% | 511.89 | 117.30 | 7.41% | 0.35 | 2.00 |
Uncued | 572.44 | 94.66 | 3.70% | 546.54 | 111.87 | 7.41% | 0.28 | 2.00 |
Manual RT variabilityd | ||||||||
Directional cue | 88.62 | 30.45 | 5.56% | 85.38 | 26.50 | 0.00% | 0.64 | 0.18 |
Nondirectional cue | 81.65 | 31.14 | 5.56% | 75.07 | 26.42 | 0.00% | 0.35 | 0.18 |
Misdirectional cue | 87.41 | 31.43 | 3.70% | 82.12 | 24.64 | 0.00% | 0.45 | 0.27 |
Gap cue | 86.04 | 29.45 | 1.85% | 84.41 | 27.86 | 3.70% | 0.81 | 2.00 |
Uncued | 93.64 | 23.27 | 1.85% | 87.68 | 25.95 | 0.00% | 0.30 | 0.54 |
Manual inhibition errors† | 0.67 | 2.02 | 3.70% | 2.29 | 4.75 | 14.81% | 0.44 | 4.00 |
TABLE 2. BEAM Saccadic and Manual Performance in Control and Mild TBI Groups
Control Group (N=54) | Mild TBI Group (N=27) | pb | Impairment Ratioc | |||||
---|---|---|---|---|---|---|---|---|
M | SD | % Impaireda | M | SD | % Impaireda | |||
Grooved pegboard, dominant hand (sec)† | 61.15 | 9.47 | 0.00% | 57.45 | 6.95 | 0.00% | 0.11 | 1.00 |
Grooved pegboard, nondominant hand (sec)† | 67.37 | 12.48 | 1.85% | 62.72 | 6.17 | 0.00% | 0.07 | 0.54 |
Digit span forward raw score | 11.06 | 2.19 | 0.00% | 11.19 | 2.43 | 0.00% | 0.81 | 1.00 |
Digit span backward raw score | 9.39 | 2.54 | 0.00% | 9.85 | 2.33 | 0.00% | 0.42 | 1.00 |
Trail Making Test A time (sec) | 24.08 | 8.82 | 1.85% | 20.50 | 7.12 | 0.00% | 0.07 | 0.54 |
Trail Making Test B time (sec)† | 52.58 | 21.60 | 0.00% | 49.78 | 16.41 | 0.00% | 0.97 | 1.00 |
CWIT condition 1 total time (sec) | 28.34 | 4.25 | 1.85% | 28.56 | 5.59 | 3.70% | 0.85 | 2.00 |
CWIT condition 2 total time (sec) | 21.35 | 3.88 | 1.85% | 21.33 | 3.43 | 0.00% | 0.99 | 0.54 |
CWIT condition 3 total time (sec) | 48.73 | 10.04 | 0.00% | 50.07 | 11.81 | 0.00% | 0.60 | 1.00 |
CWIT condition 4 total time (sec)† | 54.69 | 12.12 | 0.00% | 59.11 | 13.30 | 0.00% | 0.09 | 1.00 |
Symbol search raw score | 36.20 | 7.46 | 0.00% | 37.22 | 7.74 | 0.00% | 0.57 | 1.00 |
CVLT-II trials 1–5 total | 56.69 | 10.91 | 1.85% | 56.15 | 11.38 | 0.00% | 0.84 | 0.54 |
CVLT-II short delay free recall | 12.00 | 2.97 | 1.85% | 12.22 | 3.24 | 0.00% | 0.76 | 0.54 |
CVLT-II long delay free recall | 12.24 | 3.19 | 1.85% | 12.78 | 2.90 | 0.00% | 0.46 | 0.54 |
CPT-II hit RT (ms) | 393.65 | 47.22 | 1.85% | 389.74 | 41.83 | 0.00% | 0.72 | 0.54 |
CPT-II omissions† | 1.20 | 1.86 | 0.00% | 1.36 | 1.58 | 0.00% | 0.97 | 1.00 |
CPT-II commissions† | 11.43 | 6.61 | 3.70% | 14.88 | 5.41 | 3.70% | 0.08 | 1.00 |
CPT-II hit RT standard error† | 4.80 | 1.59 | 3.70% | 4.52 | 1.33 | 0.00% | 0.78 | 0.27 |
TABLE 3. Neuropsychological Performance in Control and Mild TBI Groups
Discussion
In this study, we compared rates of impairment on saccadic, manual, and neuropsychological measures between participants with remote mild TBI and a group of well-matched control participants. Participants with a history of mild TBI were more likely to be impaired on saccadic measures. Furthermore, those with a history of multiple mild TBIs or high levels of neurobehavioral symptoms demonstrated the highest risk for saccadic impairment. However, other neurocognitive measures did not differ by group, number of injuries, or symptom severity.
Similar to previous studies,24,26 the risk for saccadic impairment in this study was much greater among those with a history of remote mild TBI. This effect was independent of age, estimated premorbid intelligence, or severity of neurobehavioral symptoms. Impairment on individual saccadic metrics was, on average, more than three times more likely to be seen in participants with mild TBI than in those in the control group. In particular, those with mild TBI were more susceptible to attentional interference (i.e., increased RT variability for the misdirectional cue), with six times the risk for impairment on this metric relative to those in the control group. Other saccadic metrics that were most likely to be impaired in the mild TBI group (RT latency for misdirectional and gap cues, RT variability for nondirectional cues and uncued trials) are suggestive of difficulties with sustained attention, attentional interference, and temporal anticipation of visual targets. This pattern of results is consistent with previous research suggesting that eye movements requiring prediction or anticipatory control of attention are most susceptible to impact from mild TBI.18,25 Although previous studies have most commonly used smooth pursuit eye movements to measure performance variability, prediction, and other anticipatory elements of attention, results from this study suggest that cued saccadic measures may also provide value in assessment of these neurocognitive processes after mild TBI.
Additional analyses examined rates of impairment by number of mild TBIs and severity of neurobehavioral symptoms. Each of the approaches to testing (BEAM saccadic metrics, BEAM manual metrics, and conventional neuropsychological tests) yielded comparable base rates of impairment in the non-TBI control group (19%−20%). However, rates of saccadic impairment escalated to 35% among those with a single mild TBI, and further to 71% among those with a history of two or more mild TBIs. In a similar manner, 67% of those with a history of mild TBI and high levels of neurobehavioral symptoms were impaired on saccadic metrics, compared with 16% impairment among those with no history of mild TBI and low levels of symptoms. Although individuals with high levels of neurobehavioral symptoms have been shown previously to be have increased susceptibility to saccadic impairment in chronic mild TBI,19 this is the first known study to evaluate saccadic impairment as it relates to the number of mild TBIs. Considering the relatively small number of participants with multiple mild TBIs in this study, results from these subgroup analyses should be considered preliminary. Nevertheless, these findings suggest that individuals with more complex histories of mild TBI (i.e., recurrent injuries or chronic postconcussive symptoms) may be particularly likely to demonstrate saccadic impairment. This contrasts with rates of impairment among individuals with a single remote mild TBI and low levels of symptoms, for whom the rate of saccadic impairment was comparable to that of control participants.
Conventional neuropsychological measures and BEAM manual metrics were not significantly associated with a history of mild TBI in this sample. In a similar manner, rates of impairment on the conventional neuropsychological battery and BEAM manual metrics did not vary significantly by the number of injuries or severity of neurobehavioral symptoms. Although the lack of sensitivity of these measures is consistent with previous research examining effects of mild TBI after 3 months,5,6 these findings highlight differences between the current study and previous other studies of eye movements after mild TBI. Most studies in this area have obtained mild TBI samples with prominent deficits on conventional neuropsychological measures19,25 or did not provide these measures for comparison.18,22–24 Therefore, this study is among the first to demonstrate that oculomotor measures may detect impairment among patients with mild TBI who are unimpaired on conventional assessment tools.
It is noteworthy that robust differences in the rates of saccadic impairment were demonstrated between the mild TBI group and the control group despite the modest mean differences in individual saccadic metrics. This pattern of results was expected based on the known heterogeneity in neuropathology, deficits, and symptoms related to mild TBI,21,44 reflecting findings that impaired scores in the mild TBI group were distributed across individual saccadic metrics. These results suggest that an impairment index approach may be more sensitive than comparison of aggregate means in smaller samples or for clinical use with individual patients.
Results of this study must be interpreted with consideration of its limitations. As with many studies of chronic mild TBI, injuries were characterized retrospectively using a structured interview. Retrospective methods are likely to be less accurate than methods permitting the collection of detailed information at or near the time of injury. In addition, direct measurements of brain structure or neural activity were not available, preventing examination of neural mechanisms of impairment. However, our nonclinical sampling methods produced highly equivalent groups for comparison, producing profiles of neuropsychological results that mirror expectations from the literature. Also, because the study presented no opportunity for secondary gain, results are unlikely to have been biased by differences in performance validity or test-taking effort between the mild TBI group and the control group. This study was conducted in a nonclinical laboratory setting, and participants were informed that data would be used for research purposes only. This is supported by the low rate of participant failure on tests of performance validity/effort, which were comparable between the groups.
Additional research will be needed to replicate and extend these findings in a larger sample of participants, including greater representation of those with a history of multiple mild TBIs and chronic neurobehavioral symptoms. Successful replication of findings from this study could pave the way for clinical detection of cumulative effects of neurotrauma that have previously been demonstrated only using advanced neuroimaging and other laboratory procedures.7–10,46 Larger samples would also provide additional statistical power to examine subcomponents of saccadic performance that may be most relevant to neuropathology or clinical outcome after mild TBI. Cross-validation of cued saccadic measures with objective measures of neuropathology and abnormal neural activation, such as DTI, EEG, and fMRI, may also provide valuable information regarding potential mechanisms for saccadic impairment. Further investigation is also warranted into the potential effects of affective conditions or symptoms on saccadic performance. Finally, prospective research will be particularly valuable to evaluate the effects of acute mild TBI on saccadic measures of attention and to chart recovery in performance across time.
Conclusions
These findings provide additional evidence that mild TBI may be associated with chronic oculomotor impairment. In particular, this study demonstrated that saccadic measures of attention may identify forms of chronic impairment that may not be detected using conventional approaches to assessment. These impairments appear to be most common among those with a history of multiple mild TBIs and those reporting high levels of chronic symptoms. However, specific forms of saccadic impairment may differ among individual patients. Methods of saccadic assessment incorporating evaluation of multiple attention processes may be best able to identify impairment after mild TBI. Additional research will be needed to identify neural and cognitive mechanisms of saccadic impairment and trajectories of recovery after injury.
1 : Magnitudes of decline on Automated Neuropsychological Assessment Metrics subtest scores relative to predeployment baseline performance among service members evaluated for traumatic brain injury in Iraq. J Head Trauma Rehabil 2012; 27:45–54.Crossref, Medline, Google Scholar
2 : Duration of cognitive impairment after sports concussion. Neurosurgery 2004; 54:1073–1078, discussion 1078–1080Crossref, Medline, Google Scholar
3 : The ANAM lacks utility as a diagnostic or screening tool for concussion more than 10 days following injury. Mil Med 2012; 177:179–183Crossref, Medline, Google Scholar
4 : A computerized test battery sensitive to mild and severe brain injury. Medscape J Med 2008; 10:90Medline, Google Scholar
5 : A meta-analysis of neuropsychological outcome after mild traumatic brain injury: re-analyses and reconsiderations of Binder et al. (1997), Frencham et al. (2005), and Pertab et al. (2009). Clin Neuropsychol 2011; 25:608–623Crossref, Medline, Google Scholar
6 : Neuropsychological performance following a history of multiple self-reported concussions: a meta-analysis. J Int Neuropsychol Soc 2010; 16:262–267Crossref, Medline, Google Scholar
7 : Are functional deficits in concussed individuals consistent with white matter structural alterations: combined FMRI & DTI study. Exp Brain Res 2010; 204:57–70Crossref, Medline, Google Scholar
8 : A prospective diffusion tensor imaging study in mild traumatic brain injury. Neurology 2010; 74:643–650Crossref, Medline, Google Scholar
9 : Mechanisms of working memory dysfunction after mild and moderate TBI: evidence from functional MRI and neurogenetics. J Neurotrauma 2006; 23:1450–1467Crossref, Medline, Google Scholar
10 : Incidence, clinical course, and predictors of prolonged recovery time following sport-related concussion in high school and college athletes. J Int Neuropsychol Soc 2013; 19:22–33Crossref, Medline, Google Scholar
11 : Epidemiology and predictors of post-concussive syndrome after minor head injury in an emergency population. Brain Inj 1999; 13:173–189Crossref, Medline, Google Scholar
12 : Quantification of post-concussion symptoms 3 months after minor head injury in 100 consecutive patients. J Neurol 1998; 245:609–612Crossref, Medline, Google Scholar
13 : Association between recurrent concussion and late-life cognitive impairment in retired professional football players. Neurosurgery 2005; 57:719–726, discussion 719–726Crossref, Medline, Google Scholar
14 : Head injury as a risk factor for Alzheimer’s disease: the evidence 10 years on; a partial replication. J Neurol Neurosurg Psychiatry 2003; 74:857–862Crossref, Medline, Google Scholar
15 : Brain function decline in healthy retired athletes who sustained their last sports concussion in early adulthood. Brain 2009; 132:695–708Crossref, Medline, Google Scholar
16 : Inhibitory control of saccadic eye movements and cognitive impairment in Alzheimer’s disease. Biol Psychiatry 2005; 57:1052–1060Crossref, Medline, Google Scholar
17 : Don’t look now or look away: two sources of saccadic disinhibition in Parkinson’s disease? Neuropsychologia 2008; 46:3108–3115Crossref, Medline, Google Scholar
18 : Visual tracking synchronization as a metric for concussion screening. J Head Trauma Rehabil 2010; 25:293–305Crossref, Medline, Google Scholar
19 : Impaired eye movements in post-concussion syndrome indicate suboptimal brain function beyond the influence of depression, malingering or intellectual ability. Brain 2009; 132:2850–2870Crossref, Medline, Google Scholar
20 : Diagnostic tests for concussion: is vision part of the puzzle? J Neuroophthalmol 2015; 35:73–81Crossref, Medline, Google Scholar
21 : White matter abnormalities in veterans with mild traumatic brain injury. Am J Psychiatry 2012; 169:1284–1291Crossref, Medline, Google Scholar
22 : Functional neuroimaging of acute oculomotor deficits in concussed athletes. Brain Imaging Behav 2015; 9:564–573Crossref, Medline, Google Scholar
23 : Follow-up evaluation of oculomotor performance with fMRI in the subacute phase of concussion. Neurology 2015; 85:1163–1166Crossref, Medline, Google Scholar
24 : Differential eye movements in mild traumatic brain injury versus normal controls. J Head Trauma Rehabil 2015; 30:21–28Crossref, Medline, Google Scholar
25 : Filling in the gaps: Anticipatory control of eye movements in chronic mild traumatic brain injury. Neuroimage Clin 2015; 8:210–223Crossref, Medline, Google Scholar
26 : Oculomotor function in chronic traumatic brain injury. Cogn Behav Neurol 2007; 20:170–178Crossref, Medline, Google Scholar
27 : Covert orienting of attention and overt eye movements activate identical brain regions. Brain Res 2008; 1204:102–111Crossref, Medline, Google Scholar
28 : Orienting of attention and eye movements. Exp Brain Res 1994; 98:507–522Crossref, Medline, Google Scholar
29 : Reorienting attention across the horizontal and vertical meridians: evidence in favor of a premotor theory of attention. Neuropsychologia 1987; 25:31–40Crossref, Medline, Google Scholar
30 : Space and selective attention, in Attention and Performance XV: Conscious and Nonconscious Information Processing. Edited by Umiltà C, Moscovitch M. Cambridge, MA, MIT Press, 1994, pp 231–265Google Scholar
31 : The Premotor theory of attention: time to move on? Neuropsychologia 2012; 50:1104–1114Crossref, Medline, Google Scholar
32 : Multimodal assessment of visual attention using the Bethesda Eye & Attention Measure (BEAM). J Clin Exp Neuropsychol 2016; 38:96–110Crossref, Medline, Google Scholar
33 : Definition of mild traumatic brain injury. J Head Trauma Rehabil 1993; 8:86–88Crossref, Google Scholar
34 : Persistent postconcussion syndrome: The structure of subjective complaints after mild traumatic brain injury. J Head Trauma Rehabil 1995; 10:1–17Crossref, Google Scholar
35 : Wechsler Test of Adult Reading: Manual. San Antonio, TX, Pearson, Inc., 2001Google Scholar
36 : Wechsler Adult Intelligence Scale–Fourth Edition: Administration and Scoring Manual. San Antonio, TX, Pearson, Inc., 2008Google Scholar
37 : WAIS-IV: Technical and Interpretive Manual. San Antonio, TX, Pearson, Inc., 2008Google Scholar
38 : Conners’ Continuous Performance Test (CPT-II): Computer Program for Windows Technical Guide and Software Manual. Toronto, ON, Multi-Health Systems, Inc., 2000Google Scholar
39 : Delis-Kaplan Executive Function System. San Antonio, TX, The Psychological Corporation, 2001Google Scholar
40 : The relation of the trail making test to organic brain damage. J Consult Psychol 1955; 19:393–394Crossref, Medline, Google Scholar
41 : Lafayette Grooved pegboard task. Instruction/Owner's manual. Lafayette, Ind, Lafayette Instrument Company, 1989Google Scholar
42 : California Verbal Learning Test, 2nd ed. San Antonio, TX, Psychological Corporation, 2000Google Scholar
43 : Use of embedded cognitive symptom validity measures in mild traumatic brain injury cases, in Mild Traumatic Brain Injury: Symptom Validity Assessment and Malingering. Edited by Carone DA, Bush SS. New York, NY, Springer Publishing Company, 2013, pp 159–181Google Scholar
44 : Clinicopathological heterogeneity in the classification of mild head injury. Neurosurgery 1996; 38:245–250Crossref, Medline, Google Scholar
45 : Dementia diagnoses from clinical and neuropsychological data compared: the Cache County study. Neurology 2000; 54:1290–1296Crossref, Medline, Google Scholar
46 : A review of magnetic resonance imaging and diffusion tensor imaging findings in mild traumatic brain injury. Brain Imaging Behav 2012; 6:137–192Crossref, Medline, Google Scholar