Alteration of Hemoglobin Oxygenation in the Frontal Region in Elderly Depressed Patients as Measured by Near-infrared Spectroscopy
Abstract
In an attempt to elucidate the neurobiological basis of hypofrontality in depression, alterations of oxyhemoglobin and deoxyhemoglobin were examined by using near-infrared spectroscopy of the left frontal region in 9 elderly patients with depressive disorders and 10 control subjects. Verbal repetition task, verbal fluency test, hyperventilation, and paper-bag breathing were performed. During the verbal fluency test, oxyhemoglobin significantly increased and deoxyhemoglobin significantly decreased in control subjects, whereas no significant change was observed in patients. During hyperventilation, oxyhemoglobin significantly decreased and deoxyhemoglobin significantly increased in both groups. These findings suggest that functional hypofrontality in elderly depression is not due to altered vasodilator response.
Functional neuroimaging studies of depressive disorder using positron emission tomography (PET) and single-photon emission computed tomography (SPECT) have shown “hypofrontality”: glucose hypometabolism and reduction of blood flow in the frontal lobes.1–8 However, the neurobiological basis of hypofrontality is unknown. Several hypotheses have been proposed to explain why this finding is commonly observed in two separate disorders, depression and schizophrenia: 1) hypometabolism in the dorsolateral prefrontal cortex is related to symptoms, such as psychomotor poverty and psychomotor retardation, rather than disease;8 2) the patients’ attitude toward the experimental conditions is different between patients with depression and those with schizophrenia; and 3) pathophysiological mechanisms underlying prefrontal hypofunction are different between depression and schizophrenia.2 Although cognitive activation studies may be able to shed further light on these possibilities, it is still controversial whether subjects with mood disorder show hypofrontality during activation.9
Recently, the vascular depression hypothesis was presented by Alexopoulos et al.10 and Krishnan et al.11 The hypothesis was based on a finding that elderly patients with depressive disorder, compared with control subjects, more frequently had silent cerebral infarction or deep white matter hyperintensity detected by use of magnetic resonance imaging.12–15 Thus, it is possible that hypofrontality in elderly depressed patients is associated with cerebral arteriosclerosis.
The goal of this preliminary study was to characterize whether hypofrontality is associated with hypoactivity of the nervous system or with vasodilator hyporeactivity of neuronal activation. In this study, cerebral vasodilator responses were investigated during physiological tasks such as hypercapnia and hypocapnia as well as cognitive activation, to test our hypothesis that vasodilator hyporeactivity causes hypofrontality. For this purpose, it was necessary to examine alterations of cerebral blood flow during both cognitive and physiological tasks to evaluate the relationships among the responses to these tasks. However, since PET and SPECT require radioisotopes, examinations cannot be repeated many times. According to our hypothesis, this abnormal vasodilator response, if any, should be a trait-dependent rather than a state-dependent phenomenon. Therefore we examined the patients in the euthymic state because it is difficult for patients with moderately or severely depressive state to perform cognitive tasks.
Near-infrared spectroscopy (NIRS) can noninvasively and continuously monitor alterations in oxygenated (oxyHb) and deoxygenated (deoxyHb) hemoglobin by using near-infrared lights that excellently penetrate biological tissues.16 Studies using NIRS have shown increased oxyHb in the left forebrain during cognitive activation.17–21 In the present study, alterations of oxyHb and deoxyHb during cognitive and physiological tasks were measured, and the relationships among these task-related changes were monitored.
METHODS
Subjects
Subjects were 9 elderly patients with major depressive disorder and 10 healthy control subjects matched for age, sex, education, and Mini-Mental State Examination22 score (Table 1). All female subjects had passed menopause. Patients were 7 women and 2 men (mean age±SD, 65.5±6.4 years) who were recruited from inpatients or outpatients of Tokyo University Hospital. These patients with major depressive disorder had their first depressive episodes after the age of 50 years (mean age at onset, 61.8±10.7 years).
Two senior psychiatrists interviewed these patients for one hour each, referring to a checklist filled out by the patient, and the patients were medically screened by laboratory test. Patients were found not to have substance dependence disorder, endocrinological disease, head trauma, neurological disease, or severe general medical disease that was chronic (requiring lengthy treatment and medication) or acute (causing residual disability). According to DSM-IV criteria, 8 patients met the criteria for major depressive disorder, and 1 met criteria for bipolar II disorder, depressed. The mean score on the 21-item Hamilton Rating Scale for Depression23 was 5.2±4.7. All patients were taking antidepressant medication (trazodone, clomipramine, mianserine, or maprotiline) at the time of the NIRS examination.
Healthy control subjects were recruited from hospital staff. Two psychiatrists (separately) interviewed and referred to a checklist filled out by the subject. Control subjects were found not to have any psychiatric disorder such as schizophrenia or other psychotic disorders, mood disorders, or other psychiatric disorders for which the subject had visited a psychiatrist or a psychologist. Volunteers did not have MRI of the head.
All patients were examined by magnetic resonance imaging of the head and did not have abnormalities except for small silent infarctions13 or mild atrophy.24 This definition consistently applied to all patients. All patients and volunteers with uncontrolled hypertension or diabetes mellitus were excluded from the study. All subjects were right-handed as assessed with the Annett's Scale.25
Written informed consent was obtained from all participants in the study. The ethical committee of University of Tokyo approved this study.
Activation Tasks
Alterations of oxyHb and deoxyHb were measured by using NIRS during the following five conditions:
1. | Rest (3 minutes). Subjects were directed to imagine a dark sky at night. This resting condition was used as a baseline. | ||||
2. | Verbal repetition task (1 minute). Subjects were asked to recite syllables immediately after hearing audiotaped examples. For example, immediately after the tape played “a, i, u, e, o,” subjects spoke the same syllables. This task was used as a control task to the verbal fluency task. After this task, subjects had a recess for 1 minute. | ||||
3. | Verbal fluency task (1 minute). Subjects were instructed to generate as many words beginning with the sound ka as they could. After this task, subjects had a recess for 1 minute. | ||||
4. | Hyperventilation (3 minutes). Subjects were asked to breathe deeply, following the tape message “breathing in” or “breathing out.” After this task, subjects had a recess for 3 minutes. | ||||
5. | Paper-bag breathing (3 minutes). Subjects were directed to breathe with a paper bag held over their mouth. If a subject was out of breath, he or she was allowed to stop at any time. After this task, subjects were directed to have a recess for 3 minutes in order to record the recovery after this task. |
Subjects sat on a comfortable chair in a daylight room and were directed to close their eyes during the examinations.
Near-infrared Spectroscopy
NIRS measurements were performed by using HEO-200 (Omron Ltd., Inc.; Tokyo, Japan). Basic principles of NIRS were extensively discussed in a previous report by Chance et al.,26 and the principles of the NIRS instrument were described by Shiga et al.27 This instrument consisted of two-wavelength light-emitting diodes (760 nm, 840 nm), so that the two light-absorption characteristics of oxyHb and deoxyHb are different from those at the 805-nm isosbestic wavelength. Tissue oximetry using near-infrared spectroscopy was based on the modified Beer-Lambert law. In the present study, the alterations in oxyHb and deoxyHb from arbitrary baseline values were determined according to the follow equations:27
ΔdeoxyHb=A·[ΔOD(760)]−B·[ΔOD(840)]
ΔB.V.=K·[ΔOD(760)+ΔOD(840)]
ΔoxyHb=ΔB.V.−ΔdeoxyHb
where ΔOD(760) and ΔOD(840) indicate changes in optical density at 760 nm and 840 nm, respectively. ΔdeoxyHb and ΔoxyHb denote changes in the concentrations of deoxyHb, oxyHb, respectively. ΔB.V. denotes changes in blood volume, i.e., changes in the total amount of hemoglobin. The coefficients A, B, and K were assumed to be constant.
After the subject's forehead was cleaned with alcohol, the optodes were carefully fixed, using a flexible and adhesive fixation pad and an elastic band. The optodes were placed on the left forehead in the midpoint between the Fp1 and Fp3 positions according to the international 10/20 system used in electroencephalography. The distance between the optodes was 4 cm. Time resolution was set at 0.5 second. All values (arbitrary unit) were averaged and described as means plus or minus standard deviation.
Statistical Analysis
Mean values for oxyHb and deoxyHb during each activation task and the baseline period were calculated. Differences between the values of oxyHb and deoxyHb during the activation period and those at rest were used in statistical analysis with the paired two-tailed Student's t-test. The difference between patients and control subjects was examined by using two-tailed repeated-measures analysis of variance (ANOVA). Alterations of oxyHb and deoxyHb elicited in the verbal fluency and hyperventilation tests, which had been predicted from prior studies, were also compared, using the paired one-tailed Student's t-test. The SPSS software (SPSS; Tokyo, Japan) was used for statistical analysis.
RESULTS
Comparison With Baseline Values
Figure 1 shows the typical time course of the NIRS variables (oxyHb and deoxyHb) during tasks in a control subject and a depressed subject. During the verbal fluency task, oxyHb significantly increased (t=3.0, P<0.05) and deoxyHb significantly decreased (t=4.3, P<0.01) in the control group, whereas no significant alterations were observed in the depressed group (t=0.5, P>0.10, and t=0.3, P>0.10, respectively). During hyperventilation, oxyHb significantly decreased and deoxyHb significantly increased in both groups (control: t=3.4, P<0.01, and t=3.7, P<0.01; patients: t=4.8, P<0.001, and t=3.8, P<0.01, respectively). During the verbal repetition task, oxyHb and deoxyHb were not significantly altered either in patients (t=2.1, P>0.05, and t=1.9, P>0.05, respectively) or in control subjects (t=0.1, P>0.10, and t=0.2, P>0.10, respectively). No significant alterations were found during paper-bag breathing and final recovery period after paper-bag breathing.
Between-Group Comparisons
Repeated-measures ANOVA revealed significant effects of diagnosis on both oxyHb (P<0.05) and deoxyHb (P<0.05), as shown in Figure 2.
Task-evoked changes of oxyHb and deoxyHb during the verbal fluency test were significantly different between control subjects and depressed patients (t=2.6, P<0.05, and t=3.1, P<0.01, respectively). Such differences were not found during hyperventilation (t=0.6, P>0.10, and t=0.8, P>0.10, respectively). These findings were not markedly changed when the patient with bipolar II disorder was excluded.
There was no significant difference in the number of words produced by control subjects and patients during the verbal fluency test (t=1.7, P>0.05).
DISCUSSION
In the NIRS measurement, an increase in oxyHb accompanied by a decrease in deoxyHb has been thought to reflect cortical activation in the area underlying the optodes.28 In this regard, the verbal fluency task, as well as other cognitive tasks (a calculation task, a Stroop task, and the continuous performance test17–21,29), caused cognitive activation in the frontal region because oxyHb is increased and deoxyHb is decreased during this task. PET or SPECT studies also showed that the verbal fluency task activated the left frontal cortex in normal volunteers.30,31 The verbal repetition task did not significantly activate the left frontal cortex, which suggests that the activation observed during the verbal fluency task was not caused by artifacts such as extracranial muscle movement. Villringer et al.20 also showed that NIRS measurements on the left forehead were not significantly affected by skin blood flow.
Similarly to our previous study, oxyHb was significantly decreased and deoxyHb was increased during hyperventilation, which was assumed to cause hypocapnia.32 Reduction of cerebral blood flow and increased slow wave activity on EEG have been reported during hyperventilation.33,34 These findings suggest that reduction of oxyHb in the left forebrain during hyperventilation could be observed with NIRS in real time.
No significant alterations during the paper-bag breathing were found in this study. It may be that the paper-bag breathing did not cause enough hypercapnia to be detectable by using NIRS. For ethical reasons, inhalation of carbon dioxide could not be applied to elderly patients in this study.
Although there was no significant difference in task-evoked changes in oxyHb and deoxyHb between the depressed and control groups during the physiological tasks, the alteration of oxyHb in the depressed group during the verbal fluency test was significantly smaller than that in control subjects, even though depressed subjects were in remission and average performance in this test was similar between the depressed and control groups.
These findings suggest that lack of activation in the left forebrain in the depressed group during the verbal fluency test was not due to vascular distensibility, which is assumed to affect responses to both physiological and cognitive tasks.
What caused the difference between the alterations of oxyHb and deoxyHb in these two groups during the verbal fluency test? One possibility is right frontal activation in the depressed patients. Okada et al.35 reported in their pioneering work on NIRS in affective disorders that two depressed patients who had shown the “nondominant hemisphere response pattern” during the course of the illness changed to the bilateral response pattern when reexamined after recovery from depression. Warkentin et al.31 demonstrated that the verbal fluency task increased the right frontal lobe xenon-133m uptake in 20% of normal subjects. Although in the present study hemoglobin in the right side was not measured, it is possible that these depressed subjects had cognitive activation in the right frontal lobe during the verbal fluency test. To confirm this hypothesis, an investigation of the alterations both in the left and right frontal region using multichannel NIRS is required.
Another possible explanation for poor responses in the left region during the verbal fluency test is decreased dopaminergic activity. Krimer et al.36 showed that dopamine produced vasomotor responses in the cortical vasculature and suggested that their finding might be relevant to the mechanisms underlying changes in blood flow observed in neuropsychiatric disorders. Decreased dopaminergic function has been reported in depression,37 and thus dysfunction of neuronal vasodilator regulation due to decreased dopaminergic activity may have caused poor cognitive activation in depressed elderly individuals.
The exact extent of NIRS-detected changes in hemoglobin oxygenation of tissues cannot be known. It has been suggested that NIRS detects changes in the brain tissue at a depth equivalent to the distance between the two optodes,18 which was 4 cm in the present study. However, Villringer et al.21 examined the best correlation between the changes of rCBF (regional cerebral blood flow) measured with PET and the change of total Hb examined with NIRS and reported penetration to a depth of 0.9 cm from the brain surface when interoptode distance was 4 cm.
A number of methodological issues make the data difficult to interpret. NIRS is affected by a variety of factors that are difficult to control: skin color, skin structure, head thickness, depth at which changes occur, and values in only arbitrary units of oxyHb and deoxyHb. In addition, these alterations measured with NIRS are the average alterations of arterial and venous blood. Because of these methodological limitations, statistical comparison was made only on task-evoked changes in a particular region within an individual. Therefore, it should be noted that it is difficult to be certain that even a state-dependent change was due to alterations in activity in a particular region.
Other methodological problems include small sample size, which compounds difficulty, and the use of patients on medications, which makes it difficult to disentangle drug effects from disease effects. Some of the drugs, such as clomipramine and maprotiline, can affect blood pressure regulation and may affect central nervous system response. However, Okada et al.35 found no effect of antidepressants on responses measured with NIRS. Spontaneous fluctuations of baseline oxyHb and deoxyHb levels might have affected results. In addition, the effects of the former task might not have fully disappeared after only one minute, which might influence the alteration of the following task.
In spite of these limitations, this study suggested that hypofrontality in elderly depressed patients is not solely due to impaired vascular distensibility. Further studies using NIRS in other mental disorders and depressed patients with risk factors of vascular disease, such as hypertension and hyperlipidemia, are warranted.
ACKNOWLEDGMENTS
Work was supported by a Research Grant for Nervous and Mental Disorders from the Japanese Ministry of Health and Welfare (11B-3), and a research grant from Fukuda Foundation for Medical Technology. This study was presented in part at the April 1999 meeting of the Japanese Biological Psychiatry, Sendai, Japan.
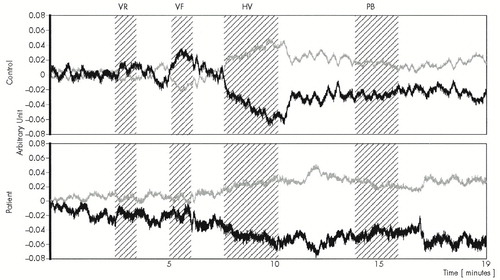
FIGURE 1. Typical time course of the NIRS variables during cognitive and physiological tasks in a control subject and a patient with depressive disorderArbitrary units correspond to micromolar concentration changes under the assumptions described in Methods. Time resolution was 0.5 second. The period in which activation tasks were applied is shaded. Black lines indicate alterations of oxyHb, and gray lines show deoxyHb. VR=verbal repetition task; VF=verbal fluency task, HV=hyperventilation task; PB=paper-bag breathing tasks.
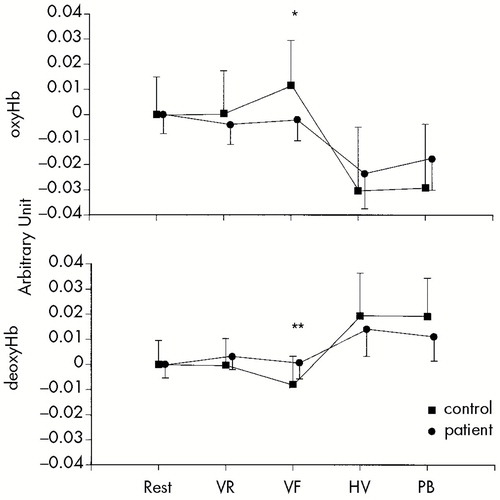
FIGURE 2. Alterations of oxyHb and deoxyHb during cognitive and physiological tasksRepeated-measures analysis of variance revealed significant effects in the diagnosis of both oxyHb (P<0.05) and deoxyHb (P<0.05). During the verbal fluency task, task-evoked changes of oxyHb and deoxyHb were significantly different between control subjects and patients with depressive disorders (*P<0.05, **P<0.01; Student's t-test).
![]() |
1 Baxter LR, Schwartz JM, Phelps ME, et al: Reduction of prefrontal cortex glucose metabolism common to three types of depression. Arch Gen Psychiatry 1989; 46:243–250Crossref, Medline, Google Scholar
2 Berman KF, Doran AR, Pickar D, et al: Is the mechanism of prefrontal hypofunction in depression the same as in schizophrenia? Regional cerebral blood flow during cognitive activation. Br J Psychiatry 1993; 162:183–192Crossref, Medline, Google Scholar
3 Galynker II, Cai J, Ongseng F, et al: Hypofrontality and negative symptom in major depressive disorder. J Nucl Med 1998; 39:608–612Medline, Google Scholar
4 Sackeim HA, Prohovnik I, Moeller JR, et al: Regional cerebral blood flow in mood disorders, I: comparison of major depressives and normal controls at rest. Arch Gen Psychiatry 1990; 47:60–70Crossref, Medline, Google Scholar
5 George MS, Ketter TA, Post RM: SPECT and PET imaging in mood disorders. J Clin Psychiatry 1993; 11(suppl):6–13Google Scholar
6 Dolan RJ, Bench CJ, Brown RG, et al: Neuropsychological dysfunction in depression: the relationship to regional cerebral blood flow. Psychol Med 1994; 24:849–857Crossref, Medline, Google Scholar
7 Dolan RJ, Bench CJ, Brown RG, et al: Regional cerebral blood flow abnormalities in depressed patients with cognitive impairment. J Neurol Neurosurg Psychiatry 1992; 55:768–773Crossref, Medline, Google Scholar
8 Dolan RJ, Bench CJ, Liddle PF, et al: Dorsolateral prefrontal cortex dysfunction in the major psychosis: symptom or disease specificity? J Neurol Neurosurg Psychiatry 1993; 56:1290–1294Google Scholar
9 George MS, Ketter TA, Parekh PI, et al: Blunted left cingulate activation in mood disorder subjects during a response interference task (the Stroop). J Neuropsychiatry Clin Neurosci 1997; 9:55–63Link, Google Scholar
10 Alexopoulos GS, Meyers BS, Young RC, et al: “Vascular depression” hypothesis. Arch Gen Psychiatry 1997; 54:915–922Crossref, Medline, Google Scholar
11 Krishnan KRR, Hays JC, Blazer DG: MRI-defined vascular depression. Am J Psychiatry 1997; 154:497–501Crossref, Medline, Google Scholar
12 Coffey CE, Figiel GS, Djang WT, et al: Subcortical hyperintensity on magnetic resonance imaging; a comparison of normal and depressed elderly subjects. Am J Psychiatry 1990; 147:187–189Crossref, Medline, Google Scholar
13 Fujikawa T, Yokota N, Muraoka M, et al: Response of patients with major depression and silent cerebral infarction to antidepressant drug therapy, with emphasis on central nervous system adverse reactions. Stroke 1996; 27:2040–2042Google Scholar
14 Greenwald BS, Kramer-Ginsberg E, Krishnan KRR, et al: MRI signal hyperintensities in geriatric depression. Am J Psychiatry 1996; 153:1212–1215Google Scholar
15 Lesser IM, Boone KB, Mehringer CM, et al: Cognition and white matter hyperintensities in older depressed patients. Am J Psychiatry 1996; 153:1280–1287Google Scholar
16 Jobsis FF: Noninvasive, infrared monitoring of cerebral and myocardial oxygen sufficiency and circulatory parameters. Science 1977; 198:1264–1267Google Scholar
17 Hoshi Y, Tamura M: Dynamic multichannel near-infrared optical imaging of human brain activity. J Appl Physiol 1993; 75:1842–1846Google Scholar
18 Hoshi Y, Onoe H, Watanabe Y, et al: Non-synchronous behavior of neuronal activity, oxidative metabolism and blood supply during mental tasks in man. Neurosci Lett 1994; 172:129–133Crossref, Medline, Google Scholar
19 Hock C, Muller-Spahn F, Schuh-Hofer F, et al: Age dependency of changes in cerebral hemoglobin oxygenation during brain activation: a near-infrared spectroscopy study. J Cereb Blood Flow Metab 1995; 15:1103–1108Google Scholar
20 Villringer A, Planck J, Hock C, et al: Near infrared spectroscopy (NIRS): a new tool to study hemodynamic changes during activation of brain function in human adults. Neurosci Lett 1993; 154:101–104Crossref, Medline, Google Scholar
21 Villringer K, Minoshima S, Hock C, et al: Assessment of local brain activation: a simultaneous PET and near-infrared spectroscopy study. Adv Exp Med Biol 1997; 413:149–153Crossref, Medline, Google Scholar
22 Folstein MF, Folstein SE, McHugh PR: “Mini-Mental State”: a practical method for grading the cognitive state of patients for the clinician. J Psychiatr Res 1975; 12:189–198Crossref, Medline, Google Scholar
23 Hamilton M: A rating scale for depression. J Neurol Neurosurg Psychiatry 1960; 23:56–62Crossref, Medline, Google Scholar
24 Coffey CE, Figiel GS, Djang WT, et al: White matter hyperintensity on magnetic resonance imaging: clinical and neuroanatomic correlates in the depressed elderly. J Neuropsychiatry Clin Neurosci 1989; 1:135–144Link, Google Scholar
25 Annett M: The binomial distribution of right, mixed and left handedness. Q J Exp Psychol 1967; 19:327–333Crossref, Medline, Google Scholar
26 Chance B, Dait MT, Zhang C, et al: Recovery from exercise-induced desaturation in the quadriceps muscle of elite competitive rowers. Am J Physiol 1992; 262:C766–C775Google Scholar
27 Shiga T, Tanabe K, Nakase Y, et al: Development of portable tissue oximeter using near infra-red spectroscopy. Med Biol Eng Comput 1995; 33:622–626Crossref, Medline, Google Scholar
28 Obrig H, Villringer A: Near-infrared spectroscopy in functional activation studies: can NIRS demonstrate cortical activation? Adv Exp Med Biol 1997; 413:113–127Google Scholar
29 Fallgatter AJ, Stric WK: Right frontal activation during the Continuous Performance Test assessed with near-infrared spectroscopy in healthy subjects. Neurosci Lett 1997; 223:89–92Crossref, Medline, Google Scholar
30 Frith CD, Friston KJ, Liddle PF, et al: A PET study of word finding. Neuropsychologia 1991; 29:1137–1148Google Scholar
31 Warkentin S, Risberg J, Nilsson A, et al: Cortical activity during speech production: a study of regional cerebral blood flow in normal subjects performing a word fluency task. Neuropsychiatry Neuropsychol Behav Neurol 1991; 4:305–316Google Scholar
32 Matsuo K, Kato T, Fukuda M, et al: Functional alterations in hemoglobin oxygenation during cognitive and physiological tasks in the left frontal lobe [in Japanese]. Brain Sci Ment Disord 1999; 10:169–175Google Scholar
33 Jibiki I, Kurokawa K, Matsuda H, et al: Widespread reduction of regional cerebral blood flow during hyperventilation-induced EEG slowing (“buildup”). Observation from subtraction of brain imaging with single photon emission computed tomography using technetium-99m hexamethyl-propyleneamine oxime. Neuropsychobiology 1992; 26:120–124Crossref, Medline, Google Scholar
34 Kraaier V, van Hoffelen AC, Wieneke GH: The hyperventilation-induced ischaemia model in human neuropharmacology: neurophysiological and psychometric studies of aniracetam and 3-OH aniracetam. Eur J Clin Pharmacol 1989; 36:605–611Crossref, Medline, Google Scholar
35 Okada F, Takahashi N, Tokumitsu Y: Dominance of the “nondominant” hemisphere in depression. J Affect Disord 1996; 37:13–21Crossref, Medline, Google Scholar
36 Krimer LS, Muly EC III, Williams GV, et al: Dopaminergic regulation of cerebral cortical microcirculation. Nat Neurosci 1998; 1:286–289Crossref, Medline, Google Scholar
37 Willner P: Dopamine and depression: a review of recent evidence, I: empirical studies. Brain Res 1983; 287:211–224Crossref, Medline, Google Scholar