Comparison Between Olanzapine and Haloperidol on Procedural Learning and the Relationship With Striatal D2 Receptor Occupancy in Schizophrenia
Abstract
The striatum is known to play a primary role in procedural learning. In this study, the authors simultaneously assessed the effects of two antipsychotic drugs on procedural learning and on striatal dopamine (D2) receptor occupancy. Twenty-seven patients receiving either olanzapine or haloperidol as antipsychotic medication were assessed with the Computed Visual Tracking Task (CVTT) and Single Photon Emission Computed Tomography (SPECT) following the administration of Iodine 123-IBZM (123I-IBZM), a radioligand with a high affinity and specificity for the D2 receptors. The results showed poorer procedural learning in the haloperidol-treated patients than in normal control subjects, while no difference could be found between olanzapine-treated patients and normal control subjects. In the haloperidol but not the olanzapine group, significant correlations were found between procedural learning deficits and striatal D2 receptor occupancy. However, there was no significant difference in D2 receptor occupancy between olanzapine- and haloperidol-treated patients, and this may be related to the high doses of olanzapine and low doses of haloperidol administered. The authors concluded that: 1) striatal D2 receptor blockade may alter procedural learning in humans; and 2) olanzapine may have a protective effect on procedural learning, even at doses that produce striatal D2 receptor occupancy as high as that found with haloperidol. This protective effect of olanzapine may be related to its atypical pharmacological properties.
Procedural learning may be defined as the development of skills in which the strategy of execution cannot be explicitly described (learning by doing), such as bicycling or hand writing. Cognitive or motor procedural skills are learned progressively with practice until the optimal performance is automatized.1 This type of learning is usually assessed with tasks requiring unfamiliar motor or cognitive skills. For instance, a subject may be asked to learn and replicate an arbitrarily defined visuospatial sequence or to track a moving target along a predictable path. Results on such tests were first reported to be abnormal in neurological illnesses involving the striatum in diseases such as Huntington's disease (HD) or Parkinson’s disease (PD).2,3,4 The striatum was then suggested to be a critical structure involved in procedural learning. Other cerebral structures such as the cerebellum, the prefrontal cortex, and the supplementary motor area have also been found to be involved, although their specific contributions vary considerably depending on the different learning stages examined and on the specific cognitive or motor processes required in the task.5,6 For instance, PET studies performed on normal subjects have shown that frontal structures are activated during the initial or adaptation stages of a sensorimotor procedural acquisition, while striatal and cerebellar structures are active throughout the learning process.5
Previous studies have concluded that patients with schizophrenia are able to learn new cognitive or motor procedures,7,8,9,10,11 although, qualitatively, the learning profile in these patients appears to differ from that of normal subjects. In particular, low learning rates,7,12 and large inter-trial fluctuations13,14,15 characterize the procedural learning of patients with schizophrenia. These abnormal procedural learning profiles have been suggested to result from a neuroleptic-induced dysfunction of the striatum8,12,13,14 or of the frontal cortex. In normal subjects, acute administration of classical neuroleptics such as chlorpromazine has been found to induce procedural learning deficits.16,17 Although a dopamine-blocking effect is suspected in these studies, there was no direct evidence of such a mechanism, and the specific contribution of the frontal cortex and the striatum remains unresolved.
In recent years, there has been a growing interest for the use of atypical antipsychotics, since, unlike the classical agents, these substances have been found to efficiently counteract the negative symptoms of schizophrenia. In addition, striatal functioning is left essentially unaffected when these substances are administered in therapeutic doses.18,19,20 Additionally, it was found that the prevalence of extrapyramidal symptoms in schizophrenia is lower with atypical than with classical neuroleptics.21,22
Patients with schizophrenia have previously been found to exhibit different learning profiles, depending on whether they were treated with a classical or an atypical neuroleptic.13,14 Clozapine- or risperidone-treated patients show normal progressive learning from trial to trial, while patients treated with haloperidol have significant learning difficulties, characterized by a poor improvement and many fluctuations from trial to trial. These results were attributed to the differential pharmacological effects of these drugs on the striatum, presumably the different dopamine (D2) receptor affinities, although there are actually no direct results supporting such a view. The present study was an attempt to further investigate this question by comparing the effects of a classical neuroleptic (haloperidol) and an atypical neuroleptic (olanzapine), on both a procedural learning task and on the striatal D2 receptor occupancy. The two hypotheses were: 1) given its effect on the striatum, the conventional neuroleptic should be more deleterious on the procedural learning than the atypical neuroleptic and 2) this deleterious effect should be related to the striatal D2 receptor occupancy, as assessed by Single Photon Emission Computed Tomography (SPECT) with 123I-IBZM.
METHODS
Subjects
Thirty patients with schizophrenia and thirty normal control subjects participated in this study. The control subjects were healthy volunteers enrolled from the general population through the local newspaper. Neurological and psychiatric conditions were ruled out during a screening interview that included physical examination and medical history, and that was performed by a trained neuropsychiatrist (E.S.) before the enrollment. The same psychiatrist also diagnosed schizophrenia in every patient taking part in the experiment. These patients were enrolled from an outpatient psychiatric clinic. The diagnosis was based on the DSM-IV criteria for paranoid, residual, undifferentiated, or disorganized forms of the syndrome. None of the patients had any other medical disease and none suffered from substance abuse (with the exception of cigarette smoking). In each group, the number of smoking persons was similar (n = 11). However, patients and control subjects had to refrain from smoking for at least 12 hours before the experiment.
Patients were enrolled on the basis of their antipsychotic treatment with olanzapine or haloperidol. These two neuroleptics were selected because of their classification as conventional and atypical neuroleptics, respectively. One of the conditions for the enrollment of the patients was that they must have been treated with their current neuroleptics for at least 3 months, without interruption, prior to the study (nonrandom assignment). There were 15 patients in the haloperidol group (mean dosage: 5.8 mg/day) and 15 patients in the olanzapine group (mean dosage: 13.8 mg/day). Following a description of the experiment, informed consent in writing was obtained from all subjects. Patients from the two neuroleptic groups were matched with 30 control subjects for age, sex, and education. Three outlier patients were excluded from the study. Two of them were from the olanzapine group and were excluded for not being able to reach the minimum performance threshold on the procedural learning task. The third rejected subject was from the haloperidol group and was excluded because of extreme saturation of dopamine receptors (three standard deviations above mean saturation).
The severity of the psychiatric symptoms in patients with schizophrenia was assessed with the Positive and Negative Syndrome Scale (PANSS).23 Occurrence of extrapyramidal symptoms was evaluated using the Extrapyramidal Symptoms Rating Scale (ESRS)24 and the Barnes Akathisia Scale.25 Neuroleptics may potentially induce motor dysfunctions that can be detected and measured with these extrapyramidal scales.
SPECT
Subjects with schizophrenia (but not the normal control subjects) were assessed with SPECT, using 123I-IBZM. This radiopharmaceutical ligand is a benzamide with an excellent specificity for D2 receptors. For each patient, 185 MBq of 123I-IBZM was injected intravenously. SPECT acquisition (two-detector camera (ADAC Vertex™); 64 projections over 360°, 40 sec./projection, 64 × 64 matrices) was initiated 90 minutes after the injection of the radioactive tracer. At that time, there is an essentially parallel variation of activity within the specific and nonspecific compartments. Reconstruction was done through a Filtered Backprojection using an adaptative Wiener filter developed in-house. Tomograms were reoriented in a standardized fashion. Uptake within the basal ganglia was scored in a semi-automated way. For this purpose, the slice from each subject showing the maximal number of counts for the two striata taken together was selected as the most representative. On that slice, Regions of Interest (ROIs) corresponding to each striatum (isocontour at 80% of the maximal counts/pixel value within the brain) were manually defined. Non-specific activity was defined using an ROI at the 20% of maximal counts/pixel isocontour level; this essentially encompasses the whole slice, excluding the striata. This method for measuring the non-specific activity was chosen given its high level of inter and intraindividual reliability,26 in comparison to the more conventional method of defining a non-specific ROI within the frontal cortex or the cerebellum. SPECT data for the two groups of schizophrenic patients were compared to normal values obtained from seven healthy subjects of the same mean age as the patients, but who did not undergo the procedural learning task. The total time for the procedural and SPECT assessments was approximately three hours (1 hour for the procedural and 2 hours for the SPECT assessment).
Task description
In the minutes following 123I-IBZM injection, a procedural learning evaluation was carried out, using the Computed Visual Tracking Task (CVTT).27 In this task, subjects must track a moving target (black circle of 1.5 cm in diameter) on a computer screen using the computer mouse. All subjects were tested on five blocks of four trials. During each trial, the target moves for 30 seconds and always follows the same geometrical pathway (a 9 × 12 cm diamond shape). Each block of trials was followed by a 45-second rest period.
For each trial, the score consists of the root mean squared error (RMSE), which is a measure of the mean distance between the target and the cursor during the tacking. Before the beginning of the testing session per se, the speed of the target was adjusted for each subject. For this purpose, practice trials were successively performed until the RMSE reached a value ranging from 30 to 45. During these practice trials, the speed of the target was progressively reduced. This adjustment allows all subjects to begin the test at the same level of performance.
Measures used as procedural learning indices included three variables: 1) the “Total Procedural Learning” is obtained by the difference of RMSE scores between the first and the last block of trials; 2) the “Average Performance” was also computed for each subject, and was defined as the mean RMSE across the 20 trials of the task; 3) the “Learning Smoothness” was computed using the statistical coefficient of determination (r2) for the 20 trials. The higher the learning smoothness score, the better the progressive improvement in performance from trial to trial. Low Learning Smoothness indicates large intertrial fluctuations during learning, that is, a difficulty to progressively automatize the performance.
Data and Statistical Method
Normality of distributions was assessed using Kolmogorov-Smirnov tests. A one-way analysis of variance (ANOVA) was used to assess between group differences on sociodemographic and pretesting procedural learning data (target speed, number of practice trials, and first trial performance). Significant between-group differences were further assessed using Tukey post hoc analysis. Clinical data were analyzed using Student's t test. The number of patients taking anticholinergic medication in each group was compared using chi square analysis. Procedural learning task analysis was carried out using a 3 (Groups) × 5 (Blocks) ANOVA with repeated measures. Between-group differences on Total Procedural Learning, Average Performance, and Learning Smoothness were assessed using an analysis of covariance (ANCOVA) with the number of practice trials (trials before the test per se) as covariate. Post hoc analyses were carried out using Tukey post hoc analyses for multiple comparisons. Relationships between procedural learning variables on the one hand, and SPECT or clinical variables on the other hand, were assessed using Pearson correlation analyses (two-tailed). Finally, a mediator effect was verified between antipsychotic doses, striatal 123I-IBZM binding, and procedural learning variables, by using both regression analyses, and a hierarchical multiple regression analysis.
RESULTS
Sociodemographic and Clinical Data
No statistically significant between-group difference was found for age and education. Clinical score analysis using Student's t test did not reveal any significant between-group differences on the PANSS total score and subscores, nor on the extrapyramidal symptoms as evaluated by the ESRS and the Barnes Akathisia Scale. Chi square analysis revealed no significant differences either, on the number of subjects taking anticholinergic medication (Table 1).
Procedural Learning Task
The one-way ANOVA revealed that the number of practice trials (before the testing session) [F(2,54) = 10.33, P < 0.001], and the speed of the moving target [F(2,54) = 12.26, P < 0.001], differed significantly across groups. Post hoc analysis revealed a significant difference between haloperidol- and olanzapine-treated patients [number of practice trials (p<0.001), target speed (p<0.001)], as well as between haloperidol patients and normal controls on these two measures [number of practice trials (P < .05); target speed (P < 0.01)]. No difference was found between the olanzapine-treated patients and control subjects for the number of practice trials and target speed. No between-group difference was found on performance at the first trial, which confirms that the three experimental groups were equal at the beginning of the test Figure 1.
The 3 (Groups) × 5 (Blocks) ANOVA with repeated measures yielded significant block [F(4,2.955) = 3.44, P < 0.05] and group [F(2,54) = 4.321, P < 0.05] effects. The Groups × Blocks interaction did not reach significance. The ANCOVAs carried out on Total Learning [F(2,54) = 5.011, P < 0.01], Average Performance [F(2,54) = 3.820, P < 0.05] and Learning Smoothness [F(2,54) = 4.021, P < 0.05] showed significant differences. Post hoc analysis revealed that the haloperidol-treated patients performed significantly worse than the normal control subjects on all the procedural learning variables [Total Learning (P < 0.05); Average Performance (P < 0.05); Learning Smoothness (P < 0.05)], while olanzapine-treated patients did not differ from the control subjects Figure 2. Haloperidol-treated patients showed also significantly lower Total Learning (P < 0.05), and Average Performance (P < 0.05) than olanzapine-treated patients. Learning Smoothness did not differ between the two groups of patients. Normality of distribution of these procedural learning variables was confirmed with the Kolmogorov-Smirnov test (Total Learning: p = 0.200; Average Performance: p = 0.200; Learning Smoothness: p = 0.173). Quality of the linear fit of the Learning Smoothness was assessed using linear regression analysis, that showed a very good fit [F(1,18) = 90.06, p < 0.001].
SPECT Analysis
A one-way ANOVA carried out on 123I-IBZM binding shows significant between groups difference for the right [F(2,32) = 33.50, P < .001], the left [F(2,32) = 39.06, P < 0.001] and the total [F(2,32) = 39.32, P < 0.001] striatal binding. Post hoc comparisons revealed no significant difference of 123I-IBZM binding between the olanzapine and haloperidol groups, although the two separate groups differed from the control subjects (Table 2).
Correlation Analysis
In the two groups of patients, antipsychotic doses correlated well with the Total Learning [haloperidol (r = −0.51, P < 0.05); olanzapine (r = −0.50, P < 0.05)], as well as with the Average Performance [haloperidol (r = 0.67, P < .01); olanzapine (r = −0.50, P < 0.05)]. However, there was no correlation between antipsychotic doses and Learning Smoothness. In addition to the procedural learning variables, antipsychotic doses also showed significant correlations with all the 123I-IBZM binding measures. More specifically, haloperidol doses correlate with the right striatum (r = −0.550, P < 0.05), left striatum (r = −0.705, P < 0.005) and the total striata (r = −0.692, P < 0.005), and olanzapine doses correlate with the right striatum (r = −0.550, P < 0.05), left striatum (r = −0.705, P < 0.005) and the total striata (r = −0.692, P < 0.005). When considering the relationship between striatal 123I-IBZM binding and the procedural learning variables, there was no significant correlation in the olanzapine group. However, in the haloperidol group, Total Learning did correlate with the left striatum (r = 0.681, P < 0.01), and the total striatum (r = 0.648, P < 0.01)], and Average Performance correlated with the left striatum (r = −0.827, P < 0.01) and total striatum (r = −0.672, P < 0.01)] (Figure 3). Learning smoothness did not correlate with any of the striatal 123I-IBZM binding, in either haloperidol- or olanzapine-treated patients. No significant correlation was found either between right striatal 123I-IBZM binding and any of the procedural learning measures, in any of the two neuroleptic groups. There was also no correlation between any of the procedural learning measures and any of the psychiatric or extrapyramidal symptom scales.
In the haloperidol group, the mediator effect of the striatal 123I-IBZM binding was confirmed first by the mediated regression analyses showing that the antipsychotic doses were significantly associated with both, 123I-IBZM binding [Total striatum F(1,12) = 11.021 p = 0.006], and CVTT scores [Average performance (F(1,12) = 9.885 p = 0.008); Total learning (F(1,12) = 5.724 p = 0.034)], and that the 123I-IBZM binding was associated with the CVTT scores [Average performance (F(1,12) = 10.274, p = 0.008) and Total learning (F(1,12) = 5.724, p = 0.034.)]. Second, a hierarchical multiple regression showed no difference of the R2 between, on the one hand, antipsychotic doses and 123I-IBZM binding taken together, and, on the other hand, the CVTT abnormal scores. This confirms a full mediation of the striatal 123I-IBZM binding, between haloperidol doses and the CVTT abnormal scores.
In the olanzapine group the mediator effect was not confirmed, given that the antipsychotic doses were significantly associated only with the 123I-IBZM binding [Total striatum F (1.11) = 5.724, p = 0.034]. No significant relationship could be detected between 123I-IBZM binding and the procedural learning scores.
DISCUSSION
The results obtained in this study indicate that patients with schizophrenia may show procedural learning disturbances, depending on their neuroleptic medication. Patients treated with olanzapine performed as well as normal controls, while those treated with haloperidol showed procedural learning deficits affecting both quantitative (total learning, average performance) and qualitative (learning smoothness) aspects of learning. The procedural learning deficits observed in the haloperidol treated patients were related to both the dose and the level of D2 receptor occupancy in the striatum. Furthermore, the mediator analyses revealed that the striatal D2 receptor occupancy is probably the mechanism by which haloperidol induces procedural learning deficits. No correlation was found between the procedural learning deficits and the social or clinical variables, including age, education, disease duration, or any of the clinical symptom scales. These social and clinical variables were not found to differ between the olanzapine and haloperidol groups. Therefore, it appears that haloperidol, but not olanzapine, induces procedural learning deficits in patients with schizophrenia, and that this effect is related to the dopaminergic blockade in the striatum.
The view that procedural learning deficits are related to striatal dopaminergic process is in accordance with other studies. Using healthy volunteers, Kumari et al.28 showed that procedural learning, as measured by a target-tracking task, may improve or worsen, depending on whether the subjects have received a dopamine agonist (such as amphetamine) or antagonist (such as haloperidol), respectively. In animals (rats), it has also been shown that the firing of the dopaminergic nigrostriatal fibers is specifically increased during the learning of a T-maze procedural task.29 Furthermore, previous studies performed in patients with schizophrenia have found that conventional neuroleptics, such as haloperidol, are more deleterious on procedural learning than atypical neuroleptics, such as clozapine.13,30 In general, the deleterious effect was hypothesized to be related to the higher striatal D2 receptor occupancy of the conventional neuroleptics, compared with the atypical neuroleptics.19,31,32 However, this view may not explain the result of the present study which shows no procedural learning deficit in the olanzapine-treated patients, in spite of a D2 receptor occupancy as high as in the haloperidol-treated patients.
The fact that the striatal D2 receptor occupancy, as measured by 123I-IBZM, did not differ between groups may appear surprising given that previous studies have shown that haloperidol possesses much greater affinity for D2 receptors than does olanzapine.32,34,35 Using 123I-IBZM single photon emission tomography (SPET), Pilowsky et al.34 reported that the striatal D2 occupancy of olanzapine is similar to that of clozapine, and much lower than that of typical neuroleptics, including haloperidol. However, this study had several limitations, including the small sample size of olanzapine-treated patients (n = 6), non-randomized dose assignment, and the different duration of olanzapine treatment among participants before SPECT scanning. Furthermore, the study found no relationship between the dose administered and D2 receptor occupancy, which is inconsistent with the literature on olanzapine,36,37,38,39 and leads one to question the internal consistency of the results.31 In another study, Tauscher and his group35 have shown that, at therapeutic doses, striatal D2 receptor occupancy is lower for olanzapine-treated patients (75%) than for haloperidol-treated patients (84%). However, despite significant group differences for striatal D2 receptor occupancy, these authors argue that the observed difference might have been confounded by the high daily dose of haloperidol used in their study (mean value of 13 mg for haloperidol and 18 mg for olanzapine). Kapur and Seeman40 also criticize the fact that clinical trials generally use high doses of typical antipsychotics (i.e., 10–20 mg/day of haloperidol), which give rise to D2 occupancy of greater than 90%; this compares to doses of atypical antipsychotics that give rise to D2 receptor occupancy of less than 80%. In fact, clinical studies with haloperidol do not suggest any advantage for dosages exceeding 5 mg/day.41 Low doses (1–5 mg/day) of haloperidol induce 60%–80% dopamine D2 receptor occupancy, which seems to be adequate to produce a clinically efficient neuroleptic response.42 As for olanzapine, Kapur and his group31 argued that at clinical doses this neuroleptic occupies more than 60% of D2 receptors and, if used at doses above 20 mg/day, occupies more than 80% of dopamine D2 receptors. They concluded that D2 receptor occupancy with olanzapine is greater than that of clozapine, and comparable to that of typical neuroleptics.31 This is consistent with our results. In our study, doses of haloperidol (5.6 mg/day) remain within the low therapeutic range, whereas olanzapine doses (13.8 mg/day) were slightly over the recommended range for that age group (10–12mg/day). This may explain why the haloperidol- and olanzapine-treated patients had equivalent striatal D2 receptor occupancy.
Even if doses and binding considerations might explain the absence of difference between the two drugs D2 receptors occupancy, this does not explain why patients treated with olanzapine had no procedural learning disturbances, while those treated with haloperidol had noticeable deficits. Olanzapine may have some protective properties against its D2 affinity. One possibility is that the anticholinergic properties of olanzapine may modulate its own D2-blocking effect within the striatum.43 It is well known that substances with anticholinergic properties may have a protective effect against D2-antagonist-induced striatal dysfunction. This constitutes the theoretical premise for administering anticholinergic substances to reduce neuroleptic-induced extrapyramidal symptoms in schizophrenia. On the other hand, patients treated with haloperidol in the present study received concurrent anticholinergic medication, and this has not prevented their procedural learning deficits. In addition, clinical scales of extrapyramidal symptoms were not different between the haloperidol- and olanzapine-treated patients, suggesting that the anticholinergic treatment in the haloperidol group was sufficient to compensate for the extrapyramidal symptoms but not the procedural learning disturbances. In other words, the procedural learning tests may be more sensitive than the extrapyramidal symptom scale at detecting neuroleptic-induced striatal dysfunction.
Another explanation may be related to the relative 5-HT2/D2 receptor affinity of the medications. Greater serotonin 5-HT2 than dopamine D2 activity is a characteristic shared by atypical neuroleptics.32,44,45,46,47 Olanzapine is classified as an atypical neuroleptic and possesses greater in vitro 5-HT2 than D2 receptor affinity.32 Haloperidol is a highly selective dopaminergic D2 receptor antagonist, and is devoid of 5-HT2 receptor activities.48 Higher 5-HT2 than D2 receptor affinity of neuroleptics has been found to be associated with a reduced incidence of extrapyramidal symptoms,21 presumably due to a compensating effect of the 5-HT2 blocking activity over the D2 blocking activity in the basal ganglia. Although the mechanism underlying this effect is not fully understood, it may involve a 5-HT2 blockade of the inhibiting serotoninergic terminals arising from the raphe and projecting to the dopaminergic terminals in the striatum.19,31,46,49 This 5-HT2 receptor blockade would increase the dopaminergic firing, thereby compensating for the D2 receptor blockade. It has been suggested previously18 that, at therapeutic doses, atypical neuroleptics do not reach a critical receptor occupancy in the nigrostriatal system (presumably associated with extrapyramidal symptoms and procedural learning deficits), while at these doses these substances may occupy significant 5-HT2 receptors in the mesolimbic and mesocortical systems (presumably associated with schizophrenic symptoms). This would protect against striatal dysfunction when treating for psychotic symptoms. However, our finding of similar striatal binding of 123I-IBZM in the haloperidol and olanzapine groups does not favor this hypothesis.
It has also been suggested that the protective effect of olanzapine in the striatum is related to its high 5-HT1A receptor agonist activity.50 These somatodendritic serotonin autoreceptors have been found in high numbers in the raphe nuclei, where they modulate the firing of serotoninergic neurons. 5-HT1A agonists like olanzapine inhibit the firing of serotoninergic neurons, once again increasing the level of dopamine in the striatum, which would have a compensating effect on its D2 receptor occupancy. The absence of any such compensating effect in the haloperidol group would, in contrast, favor striatal dysfunctions and procedural learning disturbances.
Finally, differences in procedural learning in our two groups of patients could also be explained by social and clinical factors that influence the choice of an antipsychotic. Factors such as history of treatment response, history of recent relapse, or occupational functioning and history of success in the community may all influence the choice of a conventional or atypical antipsychotic in a given patient. This would indicate different clinical profiles that may not necessarily be detected with clinical scales (as those used in the present study), but that may indirectly contribute to the differences in procedural learning between groups.
CONCLUSION
The neuroleptics examined in this study have been shown to differently affect procedural learning. Olanzapine does not disturb procedural learning in patients with schizophrenia, while haloperidol produces a generalized procedural learning deficit. These results also show a primary role of the dopaminergic processes in procedural learning, as significant correlations between procedural learning deficits and striatal D2 receptor occupancy of 123I-IBZM have been observed. The high 5-HT2 relative to D2 receptor affinity of olanzapine may be responsible for its protective effect against the neuroleptic-induced striatal dysfunction. The anticholinergic and 5-HT1A agonist activity of olanzapine may also contribute to this differential effect. Given that procedural learning plays a crucial role in many daily activities, one should take the pharmacological profile of a neuroleptic into account before using it to treat schizophrenia, in order to avoid any skill learning deficiency.
![]() |
![]() |
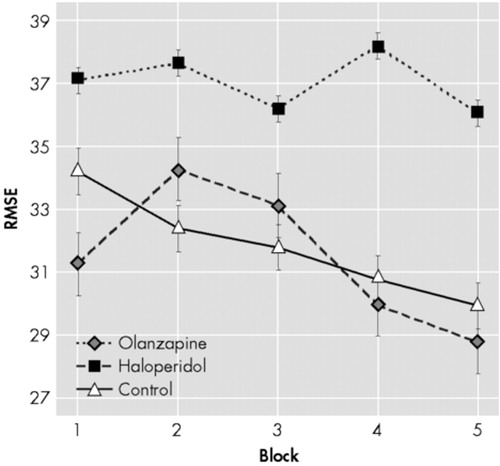
FIGURE 1. Group Performance On Each Block of Trials
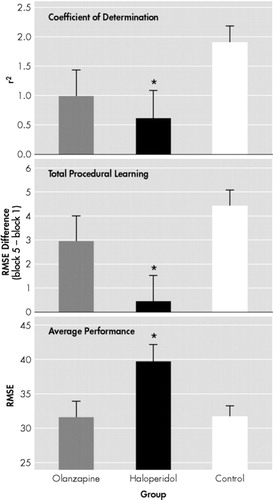
FIGURE 2. Learning Variables in the Three Groups of Subjects
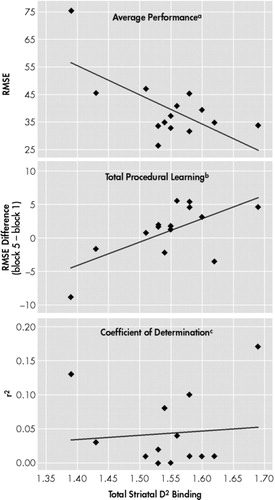
FIGURE 3. Relationship Between Learning Variables and D2 Receptor Occupancy in the Haloperidol-Treated Patients
1 Cohen NJ, Squire LR: Preserved learning and retention of pattern-analyzing skill in amnesia: Dissociation of knowing how and knowing that. Science 1980; 210:207–210Crossref, Medline, Google Scholar
2 Harrington DL, Haaland KY, Yeo RA, et al: Procedural memory in Parkinson’s disease: Impaired motor but not visuoperceptual learning. J Clin Exp Neuropsychol 1990; 12:323–339Crossref, Medline, Google Scholar
3 Knopman D, Nissen MJ: Procedural learning is impaired in Huntington's disease: Evidence from the serial reaction time task. Neuropsychologia 1991; 29:245–254Crossref, Medline, Google Scholar
4 Saint-Cyr JA, Taylor AE, Lang AE: Procedural learning and neostriatal dysfunction in man. Brain 1988; 111:941–959Crossref, Medline, Google Scholar
5 Grafton ST, Mazziotta JC, Presty S, et al: Functional anatomy of human procedural learning determined with regional cerebral blood flow and PET. J Neurosci 1992; 12:2542–2548Crossref, Medline, Google Scholar
6 Jueptner M, Frith CD, Brooks DJ, et al: Anatomy of motor learning. II. Subcortical structures and learning by trial and error. J Neurophysiol 1997; 77:1325–1337Crossref, Medline, Google Scholar
7 Goldberg TE, Saint-Cyr JA, Weinberger DR: Assessment of procedural learning and problem solving by tower of Hanoi type tasks. J Neuropsychiatr Clin Neurosci 1990; 2:165–173Link, Google Scholar
8 Granholm E, Bartzokis G, Asarnow RF, et al: Preliminary association between motor procedural learning, basal ganglia T2 relaxation times and tardive dyskinesia in schizophrenia. Psychiatr Res Neuroimag 1993; 50:33–44Crossref, Medline, Google Scholar
9 Gras-Vincedon A, Danion JM, Grangé D et al: Explicit memory, repetition priming and cognitive skill learning in schizophrenia. Schizophr Res 1994; 13:117–126Crossref, Medline, Google Scholar
10 Green MF, Kern RS, Williams O, et al: Procedural learning in schizophrenia: Evidence from striatal reaction time. Cognit Neuropsychiatry 1997; 2:123–134Crossref, Medline, Google Scholar
11 Michel L, Danion JM, Grang D, et al: Cognitive skill learning and schizophrenia: Implications for cognitive remediation. Neuropsychol 1998; 12:590–599Crossref, Google Scholar
12 Schwartz BL, Rosse RB, Veazey C, et al: Impaired motor skill learning in schizophrenia: Implications for corticostriatal dysfunction. Biol Psychiatry 1996; 39:241–248Crossref, Medline, Google Scholar
13 Bédard MA, Schérer H, Delorimier J, et al: Differential effect of D2 and D4-blocking neuroleptics on the procedural learning of schizophrenic patients. Can J Psychiatry 1996; 41:21S-24SCrossref, Medline, Google Scholar
14 Bédard MA, Schérer H, Stip E, et al: Procedural learning in schizophrenia: Further consideration on the deleterious effect of neuroleptics. Brain Cogn 2000; 43:31–39Medline, Google Scholar
15 Clare L, McKenna PJ, Mortimer AM, et al: Memory in schizophrenia: What is impaired and what is preserved? Neuropsychologia 1993; 31:1225–1241Crossref, Medline, Google Scholar
16 Danion JM, Peretti S, Grange D, et al: Effects of chlorpromazine and lorazepam on explicit memory, repetition priming and cognitive skill learning in healthy volunteers. Psychopharmacology 1992; 108:345–351Crossref, Medline, Google Scholar
17 Peretti CS, Danion JM, Kauffman-Muller F, et al: Effects of haloperidol and amisulpride on motor and cognitive skill learning in healthy volunteers. Psychopharmacology (Berl) 1997; 131:329–338Crossref, Medline, Google Scholar
18 Chiodo LA, Bunney BS: Typical and atypical neuroleptics: Differential effect of chronic administration on the activity of A9 and A10 midbrain dopamine neurons. J Neurosci 1983; 3:1607–1619Crossref, Medline, Google Scholar
19 Kapur S, Remington G, Zipursky RB, et al: The D2 dopamine receptor occupancy of risperidone and its relationship to extrapyramidal symptoms: A PET study. Life Sci 1995; 57:103–107Crossref, Medline, Google Scholar
20 Kapur S: A new framework for investigating antipsychotic action in humans: lessons from PET imaging. Mol Psychiatry 1998; 3:135–140Crossref, Medline, Google Scholar
21 Farde L, Nordström AL, Wiesel FA, et al: Positron emission tomographic analysis of central D1 and D2 dopamine receptor occupancy in patients treated with classical neuroleptics and clozapine: Relation to extrapyramidal side effects. Arch Gen Psychiatry 1992; 49:538–544Crossref, Medline, Google Scholar
22 Miller CH, Mohr F, Umbricht D, et al: The prevalence of acute extrapyramidal signs and symptoms in patients treated with clozapine, risperidone and conventional antipsychotics. J Clin Psychiatry 1998; 59:69–75Crossref, Medline, Google Scholar
23 Kay SR, Fiszbein A, Opler LA: The positive and negative syndrome scale (PANSS) for schizophrenia. Schizophr Bull 1998; 13:261–276Crossref, Google Scholar
24 Chouinard G, Chouinard AR: Extrapyramidal symptom rating scale (ESRS), 1979Google Scholar
25 Barnes TR: A rating scale for drug-induced akathisia. Br J Psychiatry 1989; 154:672–676Crossref, Medline, Google Scholar
26 Lobbezoo F, Soucy JP, Montplaisir JY, et al: Striatal D2 receptor binding in sleep bruxism: A controlled study with iodine-123-iodobenzamide and single-photon-emission computed tomography. J Dental Res 1996; 75:1804–1810Crossref, Medline, Google Scholar
27 Willingham DB, Hollier J, Joseph J: A Macintosh analogue of the rotary pursuit task. Behav Res Methods Instrum Comput 1995 27:491–495Google Scholar
28 Kumari V, Coor PJ, Mulligan OF, et al: Effects of acute administration of d-amphetamine and haloperidol on procedural learning in man. Psychopharmacology 1997 129:271–276Google Scholar
29 Jog MS, Kubota Y, Connolly CI, et al: Building neural representation of habits. Science 1999; 286:1745–1749Crossref, Medline, Google Scholar
30 Schérer H, Paquet F, Stip E, et al: Mild procedural learning disturbances in neuroleptic-naive patients with schizophrenia. J Neuropsychiatry Clin Ceurosci 2002 (in press).Google Scholar
31 Kapur S, Zipursky RB, Remington G, et al: 5-HT2 and D2 receptor occupancy of olanzapine in schizophrenia: A PET investigation. Am J Psychiatry 1998; 155:921–928Crossref, Medline, Google Scholar
32 Kapur S, Zipursky RB, Remington G: Clinical and theoretical implications of 5-HT2 and D2 receptor occupancy of clozapine, risperidone and olanzapine in schizophrenia. Am J Psychiatry 1999; 156:286–293Medline, Google Scholar
33 Bymaster FP, Calligaro DO, Falcone JF, et al: Radioreceptor binding profile of atypical antipsychotic olanzapine. Neuropsychopharmacol 1996; 14:87–96Crossref, Medline, Google Scholar
34 Pilowsky LS, Busatto GF, Taylor M, et al: Dopamine D2 receptor occupancy in vivo by the novel atypical antipsychotic olanzapine—A 123I IBZM single photon emission tomography (SPET) study. Psychopharmacology 1996; 124:148–153Crossref, Medline, Google Scholar
35 Tauscher J, Küfferle B, Asenbaum S, et al: In vivo 123I IBZM SPECT imaging of striatal dopamine-2 receptor occupancy in schizophrenic patients treated with olanzapine in comparison to clozapine and haloperidol. Psychopharmacology 1999; 141:175–181Crossref, Medline, Google Scholar
36 Dresel S, Mager T, Rossmuller B, et al: In vivo effects of olanzapine on striatal dopamine D(2)/D(3) receptor binding in schizophrenic patients: an iodine 123 iodobenzamine single-photon emission tomography study. Eur J Nucl Med 1999; 26:862–868Crossref, Medline, Google Scholar
37 Lavalaye J, Linszen DH, Booij J, et al: Dopamine D2 receptor occupancy by olanzapine or risperidone in young patients with schizophrenia. Psychiatry Res 1999; 92:33–44Crossref, Medline, Google Scholar
38 Meisenzahl EM, Dresel S, Frodl T, et al: D2 receptor occupancy under recommended and high doses of olanzapine: an iodine-123-iodobenzamine SPECT study. J Psychopharmacol 2000; 14:364–370Crossref, Medline, Google Scholar
39 Readler TJ, Knable MB, Lafargue T, et al: In vivo determination of striatal dopamine D2 receptor occupancy in patients treated with olanzapine. Psychiatry Res 1999; 90:81–90Crossref, Medline, Google Scholar
40 Kapur, S, Seeman P: Does fast dissociation from the dopamine d(2) receptor explain the action of atypical antipsychotics? A new hypothesis. Am J Psychiatry 2001; 158:360–369Crossref, Medline, Google Scholar
41 Tauscher J, Kapur S: Choosing the right dose of antipsychotics in schizophrenia: Lessons from neuroimaging studies. CNS Drugs 2001; 15:671–678Crossref, Medline, Google Scholar
42 Kapur S, Zipursky R, Roy P, et al: The relationship between D2 receptor occupancy and plasma levels on low dose oral haloperidol: A PET study. Psychopharmacology 1997; 131:148–152Crossref, Medline, Google Scholar
43 Michal P, Lysikova M, El-Fakahany EE, et al: Clozapine interaction with M2 and M4 subtypes of muscarinic receptors. Eur J Pharmacol 1999; 376:119–125Crossref, Medline, Google Scholar
44 Meltzer HY, Matsubara S, Lee JC: The ratio of serotonin2 and dopamine2 affinities differentiate atypical and typical antipsychotic drugs. Psychopharmacol Bull 1989; 25:390–392Medline, Google Scholar
45 Meltzer HY, Matsubara S, Lee JC: Classification of typical and atypical antipsychotic drugs on the basis of dopamine D-1, D-2 and serotonin pKi values. J Pharmacol Exp Ther 1989; 251:238–251Medline, Google Scholar
46 Nyberg S, Farde L, Eriksson L, et al: 5-HT2 and D2 dopamine receptor occupancy in the living human brain: A PET study with risperidone. Psychopharmacology 1993; 110:265–272Crossref, Medline, Google Scholar
47 Remington G, Kapur S: D2 and 5-HT2 receptor effects of antipsychotics: Bridging basic and clinical findings using PET. J Clin Psychiatry 1999; 60:15–19Medline, Google Scholar
48 Herrick-Davis K, Grinde E, Teitler M: Inverse agonist activity of atypical antipsychotic drugs at human 5-Hydroxytryptamine2C receptors. J Pharmacol Exp Ther 2000; 295:226–232Medline, Google Scholar
49 Meltzer HY: New insights into schizophrenia through atypical antipsychotic drugs. Neuropsychopharmacology 1998; 1:193–196Crossref, Google Scholar
50 Seeger TF, Seymour PA, Schmidt AW, et al: Ziprazidone (CP-88,059): A new antipsychotic with combined dopamine and serotonin receptor antagonist activity. J Pharmacol Exp Ther 1995; 275:101–133Medline, Google Scholar