The Habenula’s Role in Adaptive Behaviors: Contributions From Neuroimaging
A growing body of research indicates that the habenula, although tiny, plays a pivotal role in enabling adaptive behavior. The habenula’s connections to the brainstem nuclei that modulate key aspects of behavior (e.g., reward, mood, arousal) are the most studied. The habenula labels experiences as aversive or rewarding compared with expectations. This provides an important signal to guide adapting behavior to a changing or unpredictable environment. Thus, the habenula is essential for survival. Furthermore, dysregulation of the habenula may underlie many aspects of psychiatric disorders.19–23
The habenula (from the Latin word meaning little rein for its elongated shape) is an evolutionarily conserved structure that has been studied in a wide range of species (i.e., mammals, reptiles, birds, fish, amphibians).1–3 The human habenula is quite small, approximately 5–9 mm in diameter (volume 15–36 mm3).4–7 It protrudes slightly into the third ventricle on either side of the midsagittal plane between the medial dorsal thalamus and the pineal gland (Figure 1). Extensive white matter fibers make this structure appear bright on T1-weighted magnetic resonance imaging (MRI). The habenula has two primary divisions (medial and lateral), as well as multiple subnuclei. Species specific differences have been noted in both the internal organization and external connections, with both becoming more complex in higher vertebrates.7,13 A study that compared human to rodent noted that the subnuclear organization of the medial division was similar; however, the organization and relative size of the lateral division differed significantly.24 The lateral division of the habenula was sixteen times larger than the medial division in humans and only three times larger in rodents.24 The human habenula was also found to have a significantly larger area of afferent connections (via the stria medullaris). Efferent connections (via the fasciculus retroflexus) were found to exit more laterally.24 Studies in other mammals indicate that the connections and functions of the habenula’s medial and lateral divisions differ considerably. However, noninvasive studies in living humans are of the whole habenula as it is not yet possible to reliably distinguish the lateral and medial divisions on neuroimaging. Research into the functional connectivity of the human habenula is still emerging. There is growing evidence for important differences across species.7,13,25
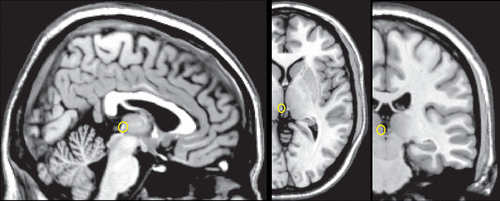
COVER AND FIGURE 1. The habenula is an evolutionarily conserved structure that has been studied in mammals, reptiles, birds, fish, amphibians.1–3 In humans the habenula is a small structure that protrudes slightly into the third ventricle on either side of the midsagittal plane between the medial dorsal thalamus and the pineal gland.4–7 Extensive white matter fibers make the habenula appear bright on T1-weighted magnetic resonance imaging (MRI). The location of the habenula is marked (yellow) on representative sagittal, axial and coronal MRIs.
In mammals, the habenula provides an important interface (sometimes termed the dorsal diencephalic conduction system) between limbic-related forebrain areas and brainstem nuclei that modulate important aspects of function including reward, affect and defense (Figure 2).1–3,8–13 Although the small size of the habenula makes it challenging to study in humans, methodological advances (e.g., higher field [7 Tesla] and higher resolution [smaller voxel] MRI, myelin sensitive imaging [ratio of T1-weighted MRI to T2-weighted MRI], diffusion tensor imaging [DTI]) are improving delineation.4–6 A few studies have assessed either structural connectivity (DTI tractography) or functional connectivity (resting state functional MRI) in healthy individuals. In general, both types of connectivity studies have reported results in humans consistent with the brainstem and subcortical connections of the habenula identified in other mammals. For example, a DTI study reported structural connectivity between the habenula (seed) and the brainstem nuclei (termination masks) that are known projection targets (i.e., ventral tegmental area/substantia nigra, raphe nuclei, periaqueductal gray, interpeduncular nucleus) for the habenula.26 Of these, only the ventral tegmental area/substantia nigra and periaqueductal gray have been reported by resting state functional MRI (fMRI) studies, perhaps due to the lower resolution of this neuroimaging approach.25,27–29
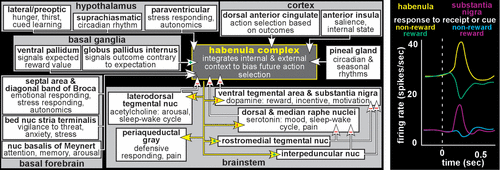
COVER AND FIGURE 2. Left. The habenula provides an important interface between limbic-related forebrain areas and brainstem nuclei that modulate multiple aspects of behavior, including reward, affect and defense.1–3,8–13 Habenula inputs (white) and outputs (yellow) are summarized, with nature labeled as excitatory (green plus) or inhibitory (red minus) where known. Right. The core role identified for the habenula is to signal occurrence of negative events that have relevance (salience) to behavior. The activity level of neurons in the habenula is increased by negative (yellow) and decreased by positive (green) reward-related events, a pattern that is the inverse of reward-related change in brainstem dopamine neurons (purple, blue).9,12,14
The greatest area of disagreement with studies in other mammals is cortical connectivity with the habenula. A DTI study that assessed structural connectivity between the habenula (termination mask) and multiple areas of prefrontal cortex (seeds) reported expected connections (pregenual anterior cingulate cortex, dorsal anterior cingulate cortex, anterior insula), but also reported connections with multiple other prefrontal areas (i.e., Brodmann’s area [BA] 8–10, BA 12, BA 44–47).30 Resting state fMRI studies have also reported functional connectivity between the habenula and both expected (e.g., medial prefrontal cortex, anterior cingulate cortex) and unexpected (e.g., hippocampus, posterior insula, parahippocampal, parieto-occipital, retrosplenial, supplementary motor, primary auditory, sensorimotor) areas of cortex.27–29 These results must be considered quite preliminary. Note that there is little overlap between the two types of connectivity studies on cortical areas that were not predicted by studies in other mammals. Although some of these may be true species differences, it is important to consider the fundamental limitations of noninvasive neuroimaging compared with invasive tracing of connections. Both DTI and resting state fMRI provide indirect measures of connectivity at comparatively low resolution.31 Overall, the structural and functional connectivity of the human habenula appears generally similar to other mammals.
Early studies on the habenula found that this structure is involved in a broad array of functions including cue-dependent learning, feeding, mating, and appropriate response to aversive events.1 The core role identified for the habenula is to signal occurrence of negative events that have relevance (salience), initially conceptualized as simply suppressing behaviors that resulted in negative outcomes. Recent studies have utilized a variety of more complex tasks (e.g., delay discounting, probabilistic reversal learning). Results indicate that the habenula integrates a very broad range of information relevant to internal state and external context to support altering behavior quickly and appropriately in response to a changing environment (e.g., behavioral flexibility, ability to change action choice based on whether previous actions resulted in expected outcomes).2,7,10,12,13,32,33 The habenula increases activity in response to a wide variety of negative events (e.g., threat/potential danger, pain/punishment, stress/distress, worse than expected outcome) or cues indicating a negative event will occur. Conversely, the habenula decreases activity to positive stimuli and cues indicating positive events will occur. Although the habenula modulates multiple brainstem areas (Figure 2), the most studied are its mutually inhibitory interactions with the monoaminergic (dopamine–ventral tegmental area/substantia nigra, serotonin–raphe nuclei) and cholinergic (acetylcholine–laterodorsal tegmental nucleus) nuclei. Thus, the habenula increases inhibition (suppresses the activity) of the brainstem modulatory nuclei when outcomes are less than optimal/expected and decreases inhibition when outcomes indicate successful choices/decisions (Figure 2). By these routes, the habenula may influence many aspect of function including mood/affective state, cognition/learning, sleep/wakefulness, and reward/ motivation (Figure 3).
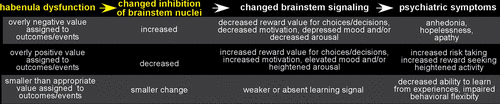
FIGURE 3. Although the habenula projects to multiple brainstem areas, the most studied are its mutually inhibitory interactions with the monoaminergic (dopamine, ventral tegmental area/substantia nigra; serotonin, raphe nuclei) and cholinergic (acetylcholine, laterodorsal tegmental nucleus) nuclei. The habenula increases inhibition of these modulatory nuclei when outcomes or events are negative (e.g., threat/potential danger, pain/punishment, stress/distress, worse than expected outcome, cue indicating a negative event will occur) and decreases inhibition when outcomes indicate successful choices/decisions.15–18 By these routes, the habenula provides an important signal to guide choices/decisions for a wide range of behaviors (e.g., goal directed, defensive, appetitive, aversive). Thus, a dysfunctional habenula has the potential to impair many aspect of function including mood/affective state, cognition/learning, sleep/waking, and reward/ motivation.
Although the majority of studies are in rodent, electrophysiological studies in nonhuman primate have confirmed that activity level of neurons in the habenula is modulated by both positive and negative reward-related events in a pattern that is the inverse of reward-related change in brainstem dopamine neurons (Figure 2).9,11,12,14,19,34 In brief, behaviorally salient negative events (e.g., omission of an expected reward [negative reward prediction error], cue signaling reward will not be delivered, cue signaling aversive stimulation will be delivered) induced increased activity, whereas positive events (e.g., receiving a larger than expected reward [positive reward prediction error], cue signaling reward will be delivered, cue signaling aversive stimulation will not be delivered) induced activity decreases. Responses were greater when the context was negative than positive even when the physical experiences were identical, indicating response modulation by subjective rather than objective value. Basal ganglia projections to the habenula are one source of both positive and negative reward-related information. Globus pallidus internus provides excitatory negative reward signaling, while ventral pallidum provides inhibitory positive reward signaling. Anterior cingulate cortex, which also projects to the habenula, is important for monitoring outcomes and appropriately adjusting goal directed behavior. The anterior cingulate cortex contains both neurons activated by negative outcomes and neurons activated by positive outcomes. Neurons in the habenula produced short latency (<200 ms) short duration (phasic) responses to negative events that were not modulated by the outcome of the previous trial. Neurons in the anterior cingulate cortex produced longer latency (>400 ms) longer duration (tonic) responses that were modulated by the outcome of the previous trial(s). This pattern of results supports the habenula’s role as signaling occurrence of behaviorally relevant negative events and anterior cingulate cortex’s role as synthesizing the history of negative events to guide initiating changes in choice behavior.
The few studies in healthy individuals utilizing task activated fMRI also support a role for the habenula in specifically signaling negative or aversive behaviorally relevant events (e.g., cue indicating painful electric shock, noxious heat, monetary loss outcome).26,35–37 A meta-analysis of fMRI studies that utilized tasks involving reinforcement learning (i.e., Pavlovian conditioning, instrumental conditioning) reported that aversive prediction errors (receiving a greater than expected punishment) activated the habenula.38 In addition to simply signaling an aversive event, the habenula is increasingly activated by environmentally related cues (conditioned stimulus) that signal negative events as they become more predictive through repeated exposure.37 Functional connectivity of the habenula with the ventral tegmental area increased during events, with greater increases to aversive than pleasant stimuli.35
The habenula has been implicated in multiple disorders that share dysregulation of dopamine and serotonin, including bipolar disorder, schizophrenia, depression, addiction, pain, and sleep disorders.17–23 As detailed above, a properly functional habenula is tonically active in the resting state, allowing it to adaptively label events as either negative (increased activity) or positive (decreased activity) outcomes.2,7,10,12,13,32,33,39 It therefore provides an important signal to guide choices/decisions for a wide range of behaviors (e.g., goal directed, defensive, appetitive, aversive). A dysfunctional habenula could label events/outcomes too strongly (i.e., overly positive, overly negative) or not strongly enough (Figure 3).15–18 It has been hypothesized that a habenula that is tonically in an overly excitatory state or is hyper-reactive will promote a negative (pessimistic) bias by signaling that outcomes are worse (e.g., less rewarding, more aversive, lower probability) than the actual events. This could increase caution and lead to over-avoidance of events that should be reinforcing. Conversely, a habenula that is tonically in an under-excitatory state or is hypo-reactive could provide a positive (optimistic) bias by signaling that outcomes are less negative (e.g., more rewarding, less aversive, higher probability) than the actual events. This could promote/reinforce maladaptive behaviors/decisions that should normally be inhibited, and lead to increased risk-taking and goal directed behaviors, as well as heightened mood, arousal, and reward-seeking.15,17,18,39 A tonically over or under active habenula might also have a smaller range of phasic responding (ceiling and/or floor effects), which could result in an outcome being labeled less strongly than appropriate, reducing signals that are essential to reinforcement learning (Figure 3).
The majority of research has focused on affective disorders as increased habenular metabolism has been reported in animal models of depression (e.g., chronic stress, learned helplessness).7,10,15,19,39–41 In addition, inhibition of the habenula (e.g., deep brain stimulation, pharmacological) reduced depressive symptomology (e.g., anhedonia, lack of motivation, distress, behavioral response to pain) in animal studies. Some of the findings have been replicated in studies examining patients with major depression (MDD). The two studies that used arterial spin labeled MRI to measure habenular cerebral blood flow (an indirect measure of metabolism) did not report resting state elevations in patients with MDD.37,42 However, tryptophan depletion (to decrease serotonin) resulted in increased resting state habenular cerebral blood flow (H215O positron emission tomography, arterial spin labeled MRI) in patients with remitted MDD but not healthy individuals.42,43 Increased habenular blood flow was associated with increased symptoms of depression in only one of the studies.43 Bilateral deep brain stimulation (DBS) of the stria medullaris to inhibit the habenula was reported successful in two patients with treatment resistant depression.44–46 DBS yielded complete sustained remission of depressive symptoms in one case and 50% reduction in the other. Onset of symptom reduction required many (8–12) weeks, while relapse was rapid when stimulation was interrupted (device malfunctions and device exchange due to infection).45,47
Differences between patients with MDD and healthy individuals on degree and direction of task-related activation (fMRI) of the habenula have also been reported. An fMRI study utilizing a probabilistic guessing task (monetary gains and losses) reported that the habenula was activated more by losses than gains in both healthy individuals and patients with MDD, but loss-related activations were lower in the MDD group and were not modulated by probability of loss.36 Similar results (trend level) were reported in an fMRI study that used a probabilistic reinforcement learning task.48 This study also reported that both greater volume of the habenula and reduced differential activations to punishments and rewards were associated with higher anhedonia. Another study reported the opposite, that smaller volume of the habenula was associated with higher anhedonia.37 This study also found that as cues became more predictive of receiving a painful electric shock, activations increased in the healthy group and decreased in the MDD group.37 Overall these studies indicate reduced habenular activation to loss/punishment in patients with MDD, a deficiency in predicting negative outcomes and in modulating behavior accordingly.36,37 These results implicate the habenula in the presentation and maintenance of symptomology which are common across both affective disorders and the affective experience of somatic disorders (i.e., pain).27,36,37,48 Dysfunctional signaling and expectation of outcomes can manifest as not learning from mistakes.22,37 Difficulty in learning from negative outcomes (errors/mistakes) and effectively processing rewards can be impaired in some psychiatric disorders.22,49,50 An fMRI study that used a visuospatial matching-to-sample task reported activation of the habenula in healthy individuals following negative feedback that was not seen in patients with schizophrenia.49 The schizophrenia group also failed to improve performance based on feedback, further supporting a deficit in processing negative feedback.49 Overall these findings suggest that habenular dysfunction is not specific to a particular psychiatric disorder, but rather that specific functional changes contribute to sets of symptoms common across disorders (Figure 3). The habenula’s role in reward signaling has led to its exploration in addiction.51 Studies in animals suggest a role for the habenula in both developing addiction and avoidance of withdrawal from drugs such as alcohol, opiates, nicotine, and stimulants.23 The habenula has been implicated in sleep disorders due to its influence on the serotonergic system, although its functional role is still largely unclear.52
Conclusions
In conclusion, the habenula has been found to influence adaptive behaviors and bias choice in responding to aversive/positive events. This evolutionarily preserved structure is important in both animals and humans with implications in several symptom clusters across disorders. With connections to serotoninergic, cholinergic, and dopaminergic systems, this structure has immense potential as a future target for innovative treatment interventions.
1 : The dorsal diencephalic conduction system: a review of the anatomy and functions of the habenular complex. Neurosci Biobehav Rev 1982; 6:1–13Crossref, Medline, Google Scholar
2 : The habenula. Curr Biol 2016; 26:R873–R877Crossref, Medline, Google Scholar
3 : Review of the cytology and connections of the lateral habenula, an avatar of adaptive behaving. Pharmacol Biochem Behav 2017; 162:3–21Crossref, Medline, Google Scholar
4 : Defining the habenula in human neuroimaging studies. Neuroimage 2013; 64:722–727Crossref, Medline, Google Scholar
5 : High-resolution MRI and diffusion-weighted imaging of the human habenula at 7 Tesla. J Magn Reson Imaging 2014; 39:1018–1026Crossref, Medline, Google Scholar
6 : Human habenula segmentation using myelin content. Neuroimage 2016; 130:145–156Crossref, Medline, Google Scholar
7 : Translating the habenula-from rodents to humans. Biol Psychiatry 2017; 81:296–305Crossref, Medline, Google Scholar
8 : Topography of descending projections from anterior insular and medial prefrontal regions to the lateral habenula of the epithalamus in the rat. Eur J Neurosci 2012; 35:1253–1269Crossref, Medline, Google Scholar
9 : Diverse sources of reward value signals in the basal ganglia nuclei transmitted to the lateral habenula in the monkey. Front Hum Neurosci 2013; 7:778Crossref, Medline, Google Scholar
10 : Habenula: recently recognized functions and potential clinical relevance. Neurology 2015; 85:992–1000Crossref, Medline, Google Scholar
11 : Roles of the lateral habenula and anterior cingulate cortex in negative outcome monitoring and behavioral adjustment in nonhuman primates. Neuron 2015; 88:792–804Crossref, Medline, Google Scholar
12 : Information processing in the vertebrate habenula. Semin Cell Dev Biol 2017; S1084-9521(16)30437-2, [Epub ahead of print]Crossref, Medline, Google Scholar
13 : The lateral habenula and adaptive behaviors. Trends Neurosci 2017; 40:481–493Crossref, Medline, Google Scholar
14 : Peril and pleasure: an rdoc-inspired examination of threat responses and reward processing in anxiety and depression. Depress Anxiety 2014; 31:233–249Crossref, Medline, Google Scholar
15 : Functional opposition between habenula metabolism and the brain reward system. Front Hum Neurosci 2013; 7:662Crossref, Medline, Google Scholar
16 : Circuit dynamics of adaptive and maladaptive behaviour. Nature 2014; 505:309–317Crossref, Medline, Google Scholar
17 : Circuits regulating pleasure and happiness in bipolar disorder. Front Neural Circuits 2017; 11:35Crossref, Medline, Google Scholar
18 : Mood instability and reward dysregulation: a neurocomputational model of bipolar disorder. JAMA Psychiatry 2017Crossref, Medline, Google Scholar
19 : The habenula: from stress evasion to value-based decision-making. Nat Rev Neurosci 2010; 11:503–513Crossref, Medline, Google Scholar
20 : DREADDing the lateral habenula: a review of methodological approaches for studying lateral habenula function. Brain Res 2013; 1511:93–101Crossref, Medline, Google Scholar
21 : The lateral habenula in addiction and depression: an anatomical, synaptic and behavioral overview. Eur J Neurosci 2014; 39:1170–1178Crossref, Medline, Google Scholar
22 : The habenula in psychiatric disorders: more than three decades of translational investigation. Neurosci Biobehav Rev 2017; S0149-7634(16)30654-6 (Epub ahead of print)Crossref, Medline, Google Scholar
23 : The medial habenula and interpeduncular nucleus circuitry is critical in addiction, anxiety, and mood regulation. J Neurochem 2017; 142(Suppl 2):130–143Crossref, Medline, Google Scholar
24 : Morphologic and immunohistochemical organization of the human habenular complex. J Comp Neurol 2011; 519:3727–3747Crossref, Medline, Google Scholar
25 : Asymmetry in functional connectivity of the human habenula revealed by high-resolution cardiac-gated resting state imaging. Hum Brain Mapp 2016; 37:2602–2615Crossref, Medline, Google Scholar
26 : Mapping pain activation and connectivity of the human habenula. J Neurophysiol 2012; 107:2633–2648Crossref, Medline, Google Scholar
27 : Habenula functional resting-state connectivity in pediatric CRPS. J Neurophysiol 2014; 111:239–247Crossref, Medline, Google Scholar
28 : Resting-state functional connectivity of the human habenula in healthy individuals: associations with subclinical depression. Hum Brain Mapp 2016; 37:2369–2384Crossref, Medline, Google Scholar
29 : Resting state connectivity of the human habenula at ultra-high field. Neuroimage 2017; 147:872–879Crossref, Medline, Google Scholar
30 : Affective and cognitive prefrontal cortex projections to the lateral habenula in humans. Front Hum Neurosci 2014; 8:819Medline, Google Scholar
31 : Measuring macroscopic brain connections in vivo. Nat Neurosci 2015; 18:1546–1555Crossref, Medline, Google Scholar
32 : Control of behavioral flexibility by the lateral habenula. Pharmacol Biochem Behav 2017; 162:62–68Crossref, Medline, Google Scholar
33 : The habenula: darkness, disappointment, and depression. Biol Psychiatry 2017; 81:e27–e28Crossref, Medline, Google Scholar
34 : Representation of negative motivational value in the primate lateral habenula. Nat Neurosci 2009; 12:77–84Crossref, Medline, Google Scholar
35 : Distinct midbrain and habenula pathways are involved in processing aversive events in humans. J Neurosci 2015; 35:198–208Crossref, Medline, Google Scholar
36 : Habenula responses to potential and actual loss in major depression: preliminary evidence for lateralized dysfunction. Soc Cogn Affect Neurosci 2016; 11:843–851Crossref, Medline, Google Scholar
37 : Disrupted habenula function in major depression. Mol Psychiatry 2017; 22:202–208Crossref, Medline, Google Scholar
38 : Prediction error in reinforcement learning: a meta-analysis of neuroimaging studies. Neurosci Biobehav Rev 2013; 37:1297–1310Crossref, Medline, Google Scholar
39 : Reward processing by the lateral habenula in normal and depressive behaviors. Nat Neurosci 2014; 17:1146–1152Crossref, Medline, Google Scholar
40 : Deep brain stimulation of the lateral habenula in treatment resistant major depression. Med Hypotheses 2007; 69:1305–1308Crossref, Medline, Google Scholar
41 : Depression: from psychopathology to pathophysiology. Curr Opin Neurobiol 2015; 30:24–30Crossref, Medline, Google Scholar
42 : The effects of tryptophan depletion on neural responses to emotional words in remitted depression. Biol Psychiatry 2009; 66:441–450Crossref, Medline, Google Scholar
43 : Covariation of activity in habenula and dorsal raphé nuclei following tryptophan depletion. Neuroimage 1999; 10:163–172Crossref, Medline, Google Scholar
44 : Remission of major depression under deep brain stimulation of the lateral habenula in a therapy-refractory patient. Biol Psychiatry 2010; 67:e9–e11Crossref, Medline, Google Scholar
45 : A new translational target for deep brain stimulation to treat depression. EMBO Mol Med 2013; 5:1151–1153Crossref, Medline, Google Scholar
46 : Deep brain stimulation for psychiatric disorders: where we are now. Neurosurg Focus 2015; 38:E2Crossref, Medline, Google Scholar
47 . Deep brain stimulation of the lateral habenular complex in treatment-resistant depression: traps and pitfalls of trajectory choice. Neurosurgery. 2013;72(2 Suppl Operative)Medline, Google Scholar
48 : Association between habenula dysfunction and motivational symptoms in unmedicated major depressive disorder. Soc Cogn Affect Neurosci 2017; 12:1520–1533Crossref, Medline, Google Scholar
49 : Schizophrenia in translation: the presence of absence: habenular regulation of dopamine neurons and the encoding of negative outcomes. Schizophr Bull 2006; 32:417–421Crossref, Medline, Google Scholar
50 : Dopaminergic circuitry and risk/reward decision making: implications for schizophrenia. Schizophr Bull 2015; 41:9–14Crossref, Medline, Google Scholar
51 . Addiction as a stress surfeit disorder. Neuropharmacology. 2014;76 Pt B:370-382.Crossref, Medline, Google Scholar
52 : The role of lateral habenula-dorsal raphe nucleus circuits in higher brain functions and psychiatric illness. Behav Brain Res 2015; 277:89–98Crossref, Medline, Google Scholar