In Vivo Evidence for Differential Association of Striatal Dopamine and Midbrain Serotonin Systems With Neuropsychiatric Symptoms in Parkinson's Disease
Abstract
Parkinson's disease affects various neurotransmitter systems. Using SPECT, the authors measured [123I]β-CIT binding ratios of the caudate, putamen, medial thalamus, and dorsal midbrain over cerebellum in 16 patients with Parkinson's disease, and examined correlations with clinical ratings. Whereas striatal binding ratios (reflecting regional dopamine transporter densities) were associated with motor symptoms, dorsal midbrain binding ratios (reflecting regional serotonin transporter densities) were significantly correlated with the mentation, behavior, and mood subscale of the Unified Parkinson's Disease Rating Scale. These findings indicate that degeneration of the nigrostriatal dopaminergic neurons and a dysfunctional serotonergic raphe system contribute differentially to motor deficits and neuropsychiatric symptoms in Parkinson's disease.
Neuropathological alterations in Parkinson's disease (PD) were previously considered to be confined to the nigrostriatal dopamine (DA) pathway. However, more recent studies found that characteristic changes occur in many other subcortical nuclei and result in multiple biochemical and pathophysiological deficits,1,2 which may explain the variety of psychiatric and cognitive symptoms in PD.2
One of the non-dopaminergic neurochemical systems potentially damaged in PD is the serotonin (5-HT) system. In addition to the neuropathological evidence of neuronal loss of brainstem 5-HT neurons,1,2 other studies found evidence of reduced 5-HT metabolite 5-hydroxyindoleacetic acid (5-HIAA) in CSF of depressed PD patients.3,4
2β-Carbomethoxy-3β-(4-iodophenyl)tropane (β-CIT), which can be labeled with iodine-123 (123I) for single-photon emission computed tomography (SPECT)5 or with carbon-11 (11C) for positron emission tomography (PET),6 is a radioligand that has a high affinity for both DA transporters (DAT) and 5-HT transporters (5-HTT). SPECT with [123I]β-CIT has the advantage of imaging simultaneously DAT and 5-HTT; the disadvantage of nonselectivity is minimized by differences in anatomical distribution of these two transporters and different kinetics of β-CIT binding to them.7
A reduced number of striatal DAT due to degeneration of nigrostriatal neurons in PD has been established by Innis et al.8 Recent studies revealed a significant negative correlation between striatal β-CIT binding and severity of motor symptoms.9,10 However, there are no published studies successfully demonstrating neurobehavioral correlates of β-CIT binding to the 5-HT reuptake sites in PD.
The purpose of this study was to examine the relationship between densities of DAT and 5-HTT, measured with [123I]β-CIT-SPECT, and symptom severity in PD. Our special interest was focused on the question of whether disruption of the brainstem raphe 5-HT system is preferentially associated with nonmotor, neuropsychiatric symptoms, in contrast with the reported association between nigrostriatal DA dysfunction and motor symptoms.
METHODS
All patients were participants in an ongoing project targeting PD and cognitive functions and were recruited from the Department of Neurology at the University of Leipzig and the Neurological Hospital Martha-Maria, Halle/Saale. All patients fulfilled the diagnostic criteria of the Parkinson's Disease Society.11 Patients with anticholinergic or antidepressant medication, severe depression, or dementia were excluded. From this wider patient group, 16 patients (13 female) for whom magnetic resonance imaging (MRI) and [123I]β-CIT-SPECT images were available, permitting overlay of both images for region of interest (ROI) analysis, were included in this study. The mean age (±SD) of the patients was 51.5±10.7 years, range 33–71, and the mean disease duration from diagnosis was 35.1±47.2 months, range 1–158. The study was approved by the ethical committee of the University of Leipzig, and informed consent was obtained from all patients.
Symptoms of PD were rated by an experienced neurologist (M.R.), who was blind to the neuroimaging data, using the Unified Parkinson's Disease Rating Scale (UPDRS) that includes the Hoehn-Yahr (HY) staging12 (mean±SD: UPDRS total, 40.9±14.6; UPDRS1, 1.6±1.5; UPDRS2, 9.9±3.6; UPDRS3, 28.1±10.0; HY stage, 2.1±0.6). According to our hypothesis, two measures of motor symptoms, namely HY stage and UPDRS3 (motor subscale), and a measure of psychiatric symptoms, namely UPDRS1 (mentation, behavior and mood subscale) were used for the correlation analyses of this study.
[123I]β-CIT was prepared with high specific activity (>117 TBq/mmol), from the corresponding trimethylstannyl precursor (Biotrend, Cologne, Germany) and [123I]NaI as previously described.13,14 After pretreatment with 100 mg of sodium perchlorate, 185–200 MBq of [123I]β-CIT were administered intravenously to all patients. The cerebral activity was registered over 30 minutes starting at two time points, 2 and 24 hours after injection of the radiopharmaceutical, using a brain-dedicated SPECT camera (Ceraspect 3000, Digital Scintigraphics Inc., Waltham, MA, USA) with three rotating collimators.15 The spatial resolution of the camera system is 8–10 mm (full width half maximum). The SPECT data were reconstructed and attenuation corrected according to a standard protocol.14
Magnetic resonance imaging was performed at 3 tesla on a Bruker Medspec 30/100 system. High-resolution whole-head three-dimensional T1-weighted images were obtained from each subject by using a modified driven equilibrium Fourier transform (MDEFT) pulse sequence: inversion time TI=0.65 s, repetition time TR=2.0 s, echo time TE=6.1 ms, flip angle=25°, field of view FOV=250×250×192 mm3, matrix size=256×256×128, voxel size=1.0×1.0×1.5 mm3.
Regions of interest analysis was performed on a Unix workstation using a commercial software package (Hermes/MultiModality, Nuclear Diagnostics, Hägersten, Sweden) by an experienced rater who was blind to the clinical scorings. In transverse MRI slices parallel to AC–PC line, ROIs for the head of the caudate nucleus, putamen, medial thalamus, and dorsal midbrain were determined at two predefined vertical levels (Figure 1, upper row). As a reference region, ROIs for the cerebellum were determined. ROIs were manually traced according to the anatomical border of each region, except for the ventral border of the dorsal midbrain and lateral border of the medial thalamus, where artificially predefined borders were adopted. The resulting ROIs were transferred to coregistered SPECT images (Figure 1, lower row). This coregistration was performed manually by using external (cerebral longitudinal fistula, lateral sulcus, cerebellum, etc.) as well as internal (striatum and midbrain) landmarks and applying shifting and rotating processes of the SPECT images to the corresponding MRI images. On the basis of regional count densities (counts/pixel), region-specific binding was calculated with the following equation: [binding ratios of a given ROI]=([counts/pixel in a given ROI]−[counts/pixel in cerebellum])/[counts/pixel in cerebellum].16 For further analyses, four respective data sets of binding ratios (upper/lower, right/left) were averaged for the putamen, caudate, and medial thalamus, as well as two data sets (upper/lower) for the dorsal midbrain.
For the measures of binding ratios of medial thalamus and dorsal midbrain, the SPECT images were used; they were acquired starting at 2 hours after tracer injection. For the measures of binding ratios of caudate and putamen, images acquired at 24 hours after tracer injection were analyzed. To check the reliability of coregistration and subsequent analyses, two raters independently determined the binding ratios in all subjects. Interrater correlations for the measured binding ratios were found to range between 0.85 and 0.92.
Statistical analyses were performed by using SPSS 6.1 for Windows (SPSS Deutschland, München). Two-sided P-values below 0.05 were considered as significant. The distribution of variables was tested first with the one-sample Kolmogorov-Smirnov test. As all behavioral variables and SPECT measures were found to be normally distributed, intercorrelations between SPECT results and correlations between behavioral measures and SPECT measures were examined by using Pearson's product-moment correlation coefficient r. We examined 1) intercorrelations between binding ratios, 2) association of striatal binding ratios (considered to reflect DAT densities) and motor ratings, 3) association of striatal binding ratios and the psychiatric rating, 4) association of medial thalamic and dorsal midbrain binding ratios (considered to reflect 5-HTT densities) and motor ratings, and 5) association of medial thalamic and dorsal midbrain binding ratios and psychiatric ratings, separately. Bonferroni's correction for multiple comparisons was applied within each hypothesis testing. Because no significant correlation was found between age and each of the SPECT results, the SPECT results were not corrected for age as a confounding effect before statistical analysis.
RESULTS
Intercorrelations Among SPECT Measures (Table 1)
There was a very strong correlation (r=0.91, P<0.001) between the binding ratios of the caudate and those of the putamen. No other significant correlation (P<0.008, according to Bonferroni's correction) was observed between binding ratios, although there was a trend of positive correlation between medial thalamic and dorsal midbrain binding ratios (r=0.57), and medial thalamic and putaminal binding ratios (r=0.52).
Correlation of Striatal Binding Ratios (Table 2)
Significant negative correlations were found between caudate binding ratios and HY stage (r=–0.75, P=0.001), and between putaminal binding ratios and HY stage (r=–0.64, P=0.008). There were nonsignificant trends of negative correlation for the other measures (caudate binding ratios and UPDRS3, putaminal binding ratios and UPDRS3; P>0.05/4, i.e., P>0.013). On the other hand, correlations between the striatal binding ratios and UPDRS1 were not statistically significant.
Correlation of Medial Thalamic and Dorsal Midbrain Binding Ratios (Figure 2)
No significant correlation was found between the medial thalamic or dorsal midbrain binding ratios and the motor measures (P>0.05/4; i.e., P>0.013). However, there were trends of negative correlation for medial thalamic binding ratios with HY stage (r=−0.52) and with UPDRS3 (r=−0.54). On the other hand, a significant negative correlation was observed between binding ratios of dorsal midbrain and UPDRS1 (r=−0.58, P=0.019). A post hoc item analysis revealed the binding ratios of dorsal midbrain to be significantly correlated with the UPDRS1 depression items (items 3+4, r=−0.53, P=0.034), but not with the UPDRS1 cognition items (items 1+2, r=−0.39, P=0.15).
DISCUSSION
This study was focused on simultaneous imaging of DAT and 5-HTT in PD. To our knowledge, this is the first neurotransmitter imaging study that has successfully demonstrated behavioral correlates of disruption of the raphe 5-HT system in PD.
Putamen and caudate nucleus are the regions where the major β-CIT binding sites are considered to be DAT, whereas β-CIT binding sites in medial thalamus and dorsal midbrain are mainly 5-HTT.17 Our findings of strong intercorrelation between DAT densities in caudate nucleus and putamen, along with the lack of significant correlation between the DAT in striatal regions and the 5-HTT in dorsal midbrain, suggest that these two separate neurochemical systems can be differentially affected in PD. However, medial thalamic binding ratios were correlated both with putaminal and dorsal midbrain binding ratios.
Striatal binding ratios, which are supposed to reflect regional DAT densities, were significantly correlated with HY stage. Although we failed to find significant correlations between striatal binding ratios and UPDRS3, there were trends for negative correlations. These findings are generally in accordance with the previously reported association between DAT densities and motor symptoms in PD.9,10,18
5-HTT densities in dorsal midbrain were not significantly correlated with motor ratings. In contrast, they were significantly correlated with UPDRS1 (mentation, behavior, and mood subscale). A recent study reported a similar finding of a negative correlation between 5-HIAA CSF level and UPDRS1 in PD patients.19 These findings suggest differential association of nigrostriatal DA and raphe 5-HT system disruption with different neurobehavioral correlates in PD. In a previous study by Kim et al.,18 the association between 5-HT transporter integrity and behavioral correlates (UPDRS subscales) was investigated. In contrast to our results, they did not find significant correlations. Difference in results may be due to our measuring method: At first, in the study of Kim et al. the [123I]β-CIT-SPECT data were obtained 20 to 24 hours after injection of the radioagent. Because of the differential kinetics of [123I]β-CIT binding to DA and 5-HT transporters,7,20,21 SPECT measures obtained from early images, as in our study, are considered to reflect 5-HT densities more purely than those from later images. Secondly, in contrast to Kim et al., who defined ROIs directly within the SPECT images, we improved ROI analysis by applying the above-described image fusion technique, using individual MRI data of all patients.22
Dysfunction of the 5-HT system results in a variety of neurobehavioral disturbances both in PD and in other neuropsychiatric diseases. There is converging evidence that depression in PD depends on 5-HT dysfunction, which is evidenced by the findings of reduced 5-HT metabolites in CSF of depressed PD patients,3,4 neuropathological findings of a reduced number of 5-HT neurons,1,2 positive tryptophan depletion test in a depressed PD patient,23 and in vivo transcranial sonography findings of reduced brainstem raphe echogenicity.24 In illicit drug users of the 5-HT neurotoxic agent 3,4-methylenedioxymethamphetamine (MDMA), a reduced level of cortical 5-HTT (measured by [123I]β-CIT-SPECT)25 and an association of reduced CSF 5-HT metabolites with cognitive deficits26 were reported. A 5-HT2 receptor imaging study of stroke patients also showed that cognitive performance is influenced by alterations in the 5-HT system.27 A recent study with [123I]β-CIT-SPECT reported reduced 5-HTT density in patients with major depression.28 Considering that UPDRS1 reflects nonmotor neurobehavioral symptoms including cognitive dysfunction and depression, our results are in good accordance with these findings.
Our post hoc analysis revealed that dorsal midbrain 5-HTT densities were significantly correlated with UPDRS1 depression items but not with cognition items. As severely depressed patients were not included in this study, further studies including PD patients with a wider range of depressive symptoms are needed to confirm the specific association between raphe 5-HT system dysfunction and depression in PD.
There are several limitations to be considered in interpreting the result of the present study. As described, SPECT images, which were acquired 2 hours after tracer injection, were used to estimate the regional 5-HTT densities. [123I]β-CIT binding to the 5-HTTs reaches a stable maximum after this period7,20 and DAT bindings are relatively low in this period, resulting in lower scatter radiation on the quantification of 5-HTT bindings. Therefore, we considered this time range to be an optimal compromise between the stability of binding ratios and selectivity of 5-HTT imaging. There are several studies whose main purpose is to demonstrate the DAT and 5-HTT dissociation. In these studies, acquisition start time similar to ours has been applied.26,29 However, as kinetic studies show,20,21 binding ratios of 2-hour images are not stable enough to be regarded as an equilibrium. A recent study suggests that images acquired between 20 and 24 hours are better for the measurement of 5-HTT densities, mainly because of the gradual decline of the tracer uptake in the reference region.21 Furthermore, no test-retest reproducibility data are available for the ROI study measures from 2-hour images, which is an additional disadvantage of this acquisition time.
Another limitation relates to the interpretation of medial thalamic binding ratios. No significant correlations emerged after Bonferroni's correction, but there was a trend of negative correlation between [123I]β-CIT binding ratios of medial thalamus and motor ratings of PD. In addition, there was a trend of positive correlation between medial thalamic and putaminal binding ratios. These trends are difficult to explain from the known pathophysiology of PD. Recent studies pointed out that scatters from high-activity regions to neighboring regions may induce apparent correlations.30 As described above, we used 2-hour images to avoid this artifact. However, the correlation coefficients of medial thalamic binding ratios with striatal binding ratios from 2-hour images (data not presented) were very high (with caudate, r=0.64; with putamen, r=0.76). Thus, scatter radiation artifact from neighboring striatum to the medial thalamus may have produced an apparent trend of association of medial thalamic 5-HTT densities with motor measures of PD. A scatter experiment, however, would be necessary to verify this hypothesis.
In summary, this preliminary study shows an association of the raphe 5-HT system with neuropsychiatric symptoms in PD. This is in contrast to the association of striatal DA system with motor symptoms in PD. In vivo demonstration of this dissociation was achieved by taking advantage of the nature of β-CIT, which binds to both DAT and 5-HTT.
ACKNOWLEDGMENTS
This work was supported by the German Bundesministerium für Bildung und Forschung (BMBF), Interdisziplinäres Zentrum für Klinische Forschung (IZFK) at the University of Leipzig (Project C-9), and Deutscher Akademischer Austauschdienst (DAAD) Bonn.
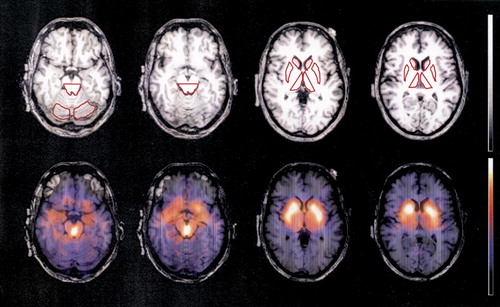
FIGURE 1. Region of interest (ROI) analysisROIs were drawn on individual MRI images. ROIs for the dorsal midbrain were determined at two different levels (the left two images in the upper row). Cerebellar ROIs were also determined for reference. ROIs for the head of caudate, putamen, and medial thalamus were determined bilaterally at two different levels (upper row, third and fourth images from the left). All ROIs were transferred to the manually coregistered SPECT images. The result of coregistration of SPECT and MRI are demonstrated as fusion images in the lower row. The color scale of the SPECT is normalized to each slice separately.
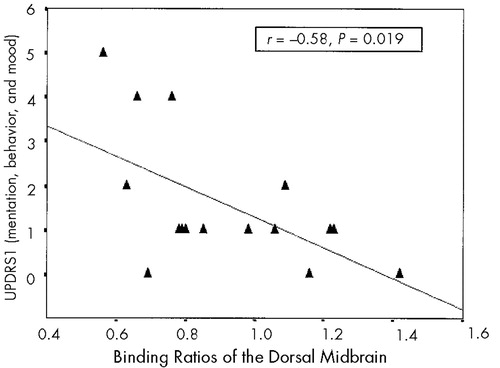
FIGURE 2. Correlation between [123I]β-CIT binding ratios of the dorsal midbrain and UPDRS1
![]() |
![]() |
1 Halliday GM, Li YW, Blumbergs PC, et al: Neuropathology of immunohistochemically identified brainstem neurons in Parkinson's disease. Ann Neurol 1990; 27:373-385Crossref, Medline, Google Scholar
2 Jellinger KA: Post mortem studies in Parkinson's disease: is it possible to detect brain areas for specific symptoms? J Neural Transm 1999; 56(suppl):1-29Google Scholar
3 Mayeux R, Stern Y, Cote L, et al: Altered serotonin metabolism in depressed patients with Parkinson's disease. Neurology 1984; 34:642-646Crossref, Medline, Google Scholar
4 Mayeux R, Stern Y, Williams JB, et al: Clinical and biochemical features of depression in Parkinson's disease. Am J Psychiatry 1986; 143:756-759Crossref, Medline, Google Scholar
5 Neumeyer JL, Wang S, Milius RA, et al: [123I]-β-Carbomethoxy-3-β-(4-iodophenyl)-tropane (β-CIT): High affinity SPECT radiotracer of monoamine reuptake sites in brain. J Med Chem 1991; 34:3144-3146Google Scholar
6 Farde L, Halldin C, Müller L, et al: PET study of [11C]β-CIT binding to monoamine transporters in the monkey and human brain. Synapse 1994; 16:93-103Crossref, Medline, Google Scholar
7 Kuikka JT, Tiihonen J, Bergström KA, et al: Imaging of serotonin and dopamine transporters in the living human brain. Eur J Nucl Med 1995; 22:346-350Crossref, Medline, Google Scholar
8 Innis RB, Seibyl JP, Scanley BE, et al: SPECT imaging demonstrates loss of striatal transporters in Parkinson's disease. Proc Natl Acad Sci USA 1993; 90:11965-11969Google Scholar
9 Seibyl JP, Marek KL, Quinlan D, et al: Decreased single-photon emission computed tomographic [123I]β-CIT striatal uptake correlates with symptom severity in Parkinson's disease. Ann Neurol 1995; 38:589-598Crossref, Medline, Google Scholar
10 Asenbaum S, Brücke T, Pirker W, et al: Imaging of dopamine transporters with iodine-123-β-CIT and SPECT in Parkinson's disease. J Nucl Med 1997; 38:1-6Medline, Google Scholar
11 Paulson HL, Stern MB: Clinical manifestation of Parkinson's disease, in Movement Disorders: Neurological Principles and Practice, edited by Watts RL, Koller WC. New York, McGraw-Hill, 1997, pp 183-199Google Scholar
12 Fahn S, Elton RL, Members of the UPDRS Development Committee: Unified Parkinson's Disease Rating Scale, in Recent Developments in Parkinson's Disease, edited by Fahn S, Marsden CD, Calne DB, et al. Florham Park, NJ, Macmillan Healthcare Information, 1987, pp 153-164Google Scholar
13 Zea-Ponce Y, Baldwin RM, Laruelle M, et al: Simplified multidose preparation of iodine-123-beta-CIT: a marker for dopamine transporters. J Nucl Med 1995; 36:525-529Medline, Google Scholar
14 Bettin S, Kämpfer I, Seese A, et al: Striatal uptake of I-123-β-CIT and I-123-IBZM in patients with extrapyramidal symptoms. Nuklearmedizin 1997; 36:167-172Crossref, Medline, Google Scholar
15 Holman BL, Carvalho PA, Zimmerman RE, et al: Brain perfusion SPECT using an annular single crystal camera: initial clinical experience. J Nucl Med 1990; 31:1456-1461Google Scholar
16 Laruelle M, Wallace E, Seibyl J, et al: Graphical, kinetic, and equilibrium analyses of in vivo [123I]β-CIT binding to dopamine transporters in healthy subjects. J Cereb Blood Flow Metab 1994; 14:982-994Crossref, Medline, Google Scholar
17 Laruelle M, Baldwin RM, Malison RT, et al: SPECT imaging of dopamine and serotonin transporters with [123I]β-CIT: pharmacological characterization of brain uptake in nonhuman primates. Synapse 1993; 13:295-309Crossref, Medline, Google Scholar
18 Kim SE, Lee WY, Choe YS, et al: SPECT measurement of iodine-123-β-CIT binding to dopamine and serotonin transporters in Parkinson's disease: correlation with symptom severity. Neurol Res 1999; 21:255-261Crossref, Medline, Google Scholar
19 Liu H, Iacono RP, Schoonenberg T, et al: A comparative study on neurochemistry of cerebrospinal fluid in advanced Parkinson's disease. Neurobiol Dis 1999; 6:35-42Crossref, Medline, Google Scholar
20 Brücke T, Kornhuber J, Angelberger P, et al: SPECT imaging of dopamine and serotonin transporters with [123I] β-CIT: binding kinetics in the human brain. J Neural Transm [Gen Sect] 1993; 94:137-146Crossref, Medline, Google Scholar
21 Pirker W, Asenbaum S, Hauk M, et al: Imaging serotonin and dopamine transporters with 123I-β-CIT SPECT: binding kinetics and effects of normal aging. J Nucl Med 2000; 41:36-44Medline, Google Scholar
22 Barthel H, Müller U, Seese A, et al: Verbesserung der Diagnostik bei Morbus Parkinson durch multimodale Bilddatenanalyse? [Improvement of the diagnosis of Parkinson's disease using multimodal imaging data analysis] (abstract). Nuklearmedizin 1999; 38:79Google Scholar
23 McCance-Katz EF, Marek KL, Price LH: Serotonergic dysfunction in depression associated with Parkinson's disease. Neurology 1992; 42:1813-1814Google Scholar
24 Becker T, Becker G, Seufert J, et al: Parkinson's disease and depression: evidence for an alteration of the basal limbic system detected by transcranial sonography. J Neurol Neurosurg Psychiatry 1997; 63:590-596Crossref, Medline, Google Scholar
25 Morris PLP, Mayberg HS, Bolla K, et al: A preliminary study of cortical S2 serotonin receptors and cognitive performance following stroke. J Neuropsychiatry Clin Neurosci 1993; 5:395-400Link, Google Scholar
26 Semple DM, Ebmeier KP, Glabus MF, et al: Reduced in vivo binding to the serotonin transporter in the cerebral cortex of MDMA (“ecstasy”) users. Br J Psychiatry 1999; 175:63-69Crossref, Medline, Google Scholar
27 Bolla KI, McCann UD, Ricaurte GA: Memory impairment in abstinent MDMA (“ecstasy”) users. Neurology 1998; 51:1532-1537Google Scholar
28 Malison RT, Price LH, Berman R, et al: Reduced brain serotonin transporter availability in major depression as measured by [123I]-2β-carbomethoxy-3β-(4-iodophenyl)tropane and single photon emission computed tomography. Biol Psychiatry 1998; 44:1090-1098Google Scholar
29 Tiihonen J, Kuikka JT, Bergström KA, et al: Single-photon emission tomography imaging of monoamine transporters in impulsive violent behaviour. Eur J Nucl Med 1997; 24:1253-60Crossref, Medline, Google Scholar
30 Fujita M, Verhoeff NP, Varrone A, et al: Imaging extrastriatal dopamine D2 receptor occupancy by endogenous dopamine in healthy humans. Eur J Pharmacol 2000; 387:179-188Crossref, Medline, Google Scholar