Diagnostic Utility of Visual Evoked Potential Changes in Alzheimer's Disease
Abstract
Previous studies have consistently found a selective delay of the P2 flash visual evoked potential (VEP) component among groups of patients with Alzheimer's dementia (AD) compared with control groups. Several authors have termed the selective P2 delay a “marker” for AD and have called for its use in clinical diagnosis. This study examined the diagnostic utility of the selective P2 delay in a retrospective sample of 45 AD patients and 60 age-equivalent healthy control subjects. Although significant between-group differences were found, classification accuracies for individual patients and controls were too low for the P2 delay to contribute meaningfully to clinical diagnosis.
A substantial literature has documented a delayed P2 component of the flash visual evoked potential (VEP) in groups of patients with probable Alzheimer's dementia (AD) compared with groups of age-matched normal control subjects. Because many visual system pathologies can produce VEP component delays, the selective P2 delay is often measured relative to either the flash P1 or the pattern reversal P100. The P2 is said to be selectively delayed because both the flash P1 and the pattern reversal P100 VEP components are normal in probable AD patients. The selective P2 delay is thought to mirror the cholinergic deterioration in AD because it arises from the richly cholinergic visual association cortex, whereas the normal P1 and P100 arise from the sparsely cholinergic primary visual cortex.1 A selective P2 delay may be produced in subjects who do not have dementia by pharmacological suppression of cholinergic functioning.1,2
Among AD patients with otherwise intact visual systems, these interpeak measures show the same delays as absolute P2 latency measures.3,4 The selective P2 delay is not found in patient groups suffering from nondementing psychiatric disorders5,6 or other forms of dementia,3,4 but in AD groups it increases over time, paralleling dementia severity.7,8 These findings show remarkable consistency across laboratories and recording techniques.9 Several authors5,9,10 have suggested that the flash P2 delay should be investigated as a simple and reliable diagnostic test for AD, perhaps in its earliest stages.11 Currently the diagnostic workup for AD described by McKhann et al.,12 a process of exclusion rather than detection, is costly, time-consuming, and cumbersome. A simple positive test for AD could add precision to the diagnostic process or, if it were correlated highly with the final clinical diagnosis, could shortcut the process.
This study addressed the question of the diagnostic utility of the selective P2 delay: To what extent is a selectively delayed flash P2 correlated with the clinical diagnosis of AD according to the McKhann criteria in individual patients?
METHOD
Indexes of sensitivity and specificity were calculated for a range of possible cutoff scores on VEP component data from retrospective samples of 45 AD patients (mean age, 73.2 years, SD = 8.69) and 60 healthy elderly control subjects (mean age, 70.7 years, SD = 8.03). All AD patients had been referred for participation in research studies conducted at the Southern Illinois University School of Medicine or at the Mercer University School of Medicine and had received thorough workups culminating in a diagnosis of probable AD according to the McKhann et al.12 criteria. Diagnostic assessment was made before VEP testing was done. The healthy controls had been recruited at the same time and for the same research studies as the patients. Except for signs and symptoms related directly to Alzheimer's disease in the patients, patients and controls all had medical histories free of significant neurological or psychiatric difficulties and had no other health problems that were likely to affect brain activity. Both patients and controls were not taking any medications that have significant effects on the cholinergic system, including cholinesterase inhibitors. Although they were not specifically assessed, the general educational level and medical burden (except for the dementia itself) on patients and controls appeared comparable. All participants originally had enrolled in various research studies examining EEG changes in dementia, conducted either at the Southern Illinois University School of Medicine or at the Mercer University School of Medicine. After all procedures had been carefully explained to participants and, when appropriate, their caregivers, written informed consent, as approved by the institutional review boards of the respective medical schools, had been obtained from all participants or their caregivers.
For all participants, flash and pattern reversal VEP data were recorded from 20 active scalp channels of the international 10-20 system, using techniques that have been detailed elsewhere.3 To include recordings using different physical reference electrodes, all data were converted to a “global average” virtual reference system involving all scalp electrodes.4 Additional post hoc analyses were performed using partial average and Hjorth Laplacian reference systems. All analyses were performed blind to participant group.
Since many clinical neurodiagnostic laboratories lack the ability to perform topographic mapping or sophisticated quantitative analysis of electrophysiological data, simplified nontopographic methods were used to identify VEP components in this study. The flash P1 was identified as the maximum positivity at Pz within a 60–100 ms latency window. The flash P2 and pattern reversal P100 components were identified as the maximum positivities at Oz within 100–300 ms and 90–140 ms latency windows, respectively. Previous topographic studies9 identified these recording sites as showing the components at their maximum amplitudes in both probable AD patients and healthy elderly controls. The P2 latency was measured as an interpeak latency relative to the P1 or P100.
Measures of sensitivity (proportion of patients correctly identified) and specificity (proportion of controls correctly identified) were calculated for each interpeak latency and reference system. Assuming that both psychometric properties are of equal value in clinical diagnosis, the latency was identified at which the sum of sensitivity and specificity was maximal. At that latency the overall proportion of correct classifications was computed as an “accuracy” measure. Positive and negative predictive values also were calculated, as was the area under each ROC curve. ROC areas provide an overall estimate of the diagnostic accuracy of each dependent variable, complementing the point measures of accuracy computed from sensitivity and specificity.
A multivariate analysis of covariance (MANCOVA) was conducted to determine whether probable AD patients exhibit a selective P2 delay relative to healthy elderly controls. Age was used as a covariate, and the selective P2 delays associated with the global and partial average reference systems and the Hjorth reference system were treated as dependent measures. The family-wise error rate (FWa) associated with the MANCOVA was 0.05. Separate ANCOVAs were used as follow-up tests. All statistical tests were two-tailed.
RESULTS
The primary analysis used global average referenced data (Table 1; Figure 1). Compared with the control group, the probable AD group exhibited a significant delay in the P2 component, whether measured as interpeak latencies from the P1 (F = 6.22, df = 1, 102, P<0.05) or from the P100 (F = 10.04, df = 1, 102, P<0.05), thereby replicating the P2 delays commonly reported in this disorder. Measures of treatment effect, eta-squared, for the P1–P2 and P100–P2 interpeak latencies were 0.06 and 0.09, respectively. However, the classification accuracies for the P1–P2 (accuracy 62%, sensitivity 80%, specificity 53%) and for the P100–P2 (accuracy 68%, sensitivity 60%, specificity 75%) were too low to add meaningful information to the McKhann diagnostic process or to substitute for the complete diagnostic workup.
One possible reason for the low diagnostic accuracy of the global average referenced VEP data might be a contamination of the reference by electro-oculogram (EOG) artifacts that were of low enough amplitude to avoid the automatic EOG rejection algorithm during data acquisition.13
In the next part of the study, VEP data were transformed to a “partial average” virtual reference system omitting Fp1, Fpz, and Fp2 electrodes to minimize any possible EOG distortions. The partial average reference again demonstrated a significant delay in the P2 component among the AD group (Table 1; Figure 1), whether measured as interpeak latencies from the P1 (F = 6.30, df = 1,102, P<0.05) or from the P100 (F = 9.03, df = 1,102, P<0.05), replicating the between-group differences found above. Eta-squared for the P1–P2 and P100–P2 interpeak latencies were 0.06 and 0.08, respectively. Although the two groups clearly differed on average, there was considerable overlap, and the classification accuracies for the P1–P2 (accuracy 63%, sensitivity 76%, specificity 55%) and for the P100–P2 (accuracy 68%, sensitivity 44%, specificity 87%) again were too low to add meaningful information to the McKhann diagnostic process or to serve as a shortcut for the clinical workup.
Another possible reason for the low diagnostic accuracy of the global average and partial average referenced VEP data could be that the waveforms recorded at one or more of the electrodes represent a mixture of activity from several closely adjacent areas. Activity that is time-locked to the visual stimulus but unaffected by AD would constitute “noise” in the present context, thereby degrading any AD-specific signal. Although the topographic distributions of the pattern reversal P100 component generated by primary visual cortex and the flash P2 component generated by visual association cortex differ, both show maximal amplitudes at Oz. To assess the relative contributions of the AD-specific signal and nonspecific noise at Oz, VEP data were transformed to a Hjorth Laplacian reference system emphasizing locally generated components with high spatial frequencies.
The Hjorth reference (Table 1; Figure 1) showed nonsignificant P2 component delays in the AD group, whether measured as interpeak latencies from the P1 (F = 0.01, df = 1, 102, P>0.05) or from the P100 (F = 1.42, df = 1, 102, P>0.05). Eta-squared values for the P1–P2 and P100–P2 interpeak latencies were 0.00 and 0.01, respectively. AD and control groups showed virtually complete overlap, and the classification accuracies for the P1–P2 (accuracy 58%, sensitivity 38%, specificity 77%) and for the P100–P2 (accuracy 64%, sensitivity 58%, specificity 78%) were unimpressive.
It appears that the locally generated VEP activity recorded at Oz (to the extent that this is accentuated by the Hjorth reference) is unaffected by the AD process and that the very significant between-group differences found using other reference systems represents activity deriving from the visual association areas surrounding Oz. However, this interpretation is speculative, since inspection of Hjorth-referenced waveforms recorded from the international 10-20 system electrode sites surrounding Oz also showed no evidence of delayed P2 components among AD participants. This suggests that the spatial sampling of the cortex by the 20-electrode array is too coarse to resolve affected areas and that higher-density arrays will be necessary if the advantages of the Hjorth system are to be exploited.
DISCUSSION
The purpose of this study was to examine the selective delay of the flash P2 component in AD patients using simple stimulation, recording, and analysis methods available in most clinical electroneurodiagnostic laboratories and to ascertain whether the P2 delay contained diagnostically relevant information about individual patients. The between-group differences obtained using global and partial average reference systems in this study (as well as a variety of other reference systems used by others) suggest that an AD-specific signal containing potentially pathognomonic information is contained within VEP data. However, the modest classification accuracies found in this study show that present methods do not adequately capture that information. In terms of clinical utility, measurement of the flash P2 latency using standard methods does not appear to add meaningful information to the McKhann diagnostic process and does not offer a useful shortcut of the complete diagnostic workup.
Future research should refine the methods currently being used. This may include a refinement of the visual stimulus to accentuate the flash P2 signal or the use of a higher-density electrode array. The increased spatial resolution offered by the latter may allow for a differentiation of an AD-specific signal. Finally, alternative analytic methods14,15 may better separate AD patients from healthy controls,16 with the limitation that these methods should be appropriate for the facilities found in most electroneurodiagnostic laboratories. A follow-up study examining these possibilities is in progress.
ACKNOWLEDGMENTS
Portions of these results were presented at the Eighth World Congress of the International Society for Brain Electromagnetic Topography, Zurich, March 6–8, 1997. This work was supported in part by grants from the Medical Center of Central Georgia.
![]() |
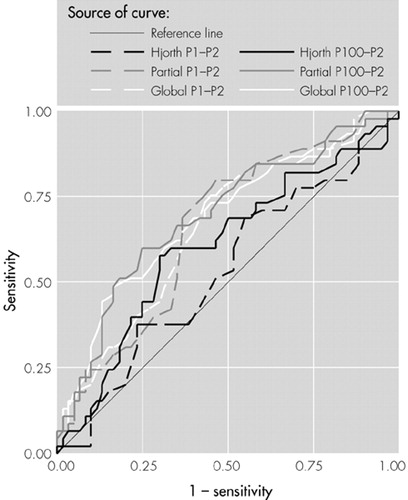
FIGURE 1. Receiver operating characteristic (ROC) curves associated with the partial-, global-, and Hjorth-referenced P2 interpeak latencies. Diagonal segments are produced by ties.
1 Coburn KL, Parks RW, Pritchard WS: Electrophysiological indexes of cortical deterioration and cognitive impairment in dementia, in Neuropsychology of Alzheimer's Disease and Other Dementias. Edited by Parks RW, Zec RF, Wilson RS. New York, Oxford University Press, 1993, pp 511-533Google Scholar
2 Bajalan AAA, Wright CE, Van Der Vliet VJ: Changes in the human visual evoked potential caused by the anticholinergic agent hyocine hydrobromide: comparison with results in Alzheimer's disease. J Neurol Neurosurg Psychiatry 1986; 49:175-182Crossref, Medline, Google Scholar
3 Coburn KL, Ashford JW, Moreno MA: Visual evoked potentials in dementia: selective delay of flash P2 in probable Alzheimer's disease. J Neuropsychiatry Clin Neurosci 1991; 3:431-435Link, Google Scholar
4 Coburn KL, Ashford JW, Moreno MA: Delayed late component of visual global field power in probable Alzheimer's disease. J Geriatr Psychiatry Neurol 1993; 6:72-77Crossref, Medline, Google Scholar
5 Wright CE, Harding GFA, Orwin A: Presenile dementia: the use of the flash and pattern VEP in diagnosis. Electroencephalogr Clin Neurophysiol 1984; 57:405-415Crossref, Medline, Google Scholar
6 Wright CE, Harding GFA, Orwin A: The flash and pattern VEP as a diagnostic indicator of dementia. Doc Ophthalmol 1986; 62:89-96Crossref, Medline, Google Scholar
7 Moore NC, Tucker KA, Jann MW, et al: Flash P2 delay of primary degenerative dementia increases with time. Brain Topogr 1995; 7:333Google Scholar
8 Parks RW, Zec RF, Kuhn M, et al: Electrocortical mapping, MRI, and neuropsychological measures: evidence of Alzheimer's disease in the presence of vascular lesions. Arch Clin Neuropsychol 1991; 6:393-408Crossref, Medline, Google Scholar
9 Moore NC, Tucker KA, Jann MW, et al: Flash P2 delay in primary degenerative dementia of the Alzheimer type. Prog Neuropsychopharmacol Biol Psychiatry 1995; 19:403-410Crossref, Medline, Google Scholar
10 Doggett CE, Harding GFA, Orwin A: Flash and pattern evoked potentials in patients with presenile dementia. Electroencephalogr Clin Neurophysiol 1981; 52:100PGoogle Scholar
11 Moore NC, Tucker KA, Khairy NM, et al: Flash P2 visual evoked response delay may be a marker of cognitive dysfunction in healthy elderly volunteers. Int Psychogeriatr 1996; 8:549-559Crossref, Medline, Google Scholar
12 McKhann G, Drachman D, Folstein M, et al: Clinical diagnosis of Alzheimer's disease. Neurology 1984; 34:939-944Crossref, Medline, Google Scholar
13 Coburn KL, Moreno MA: Facts and artifacts in brain electrical activity mapping. Brain Topogr 1988; 1:37-45Crossref, Medline, Google Scholar
14 Pritchard WS, Duke DW, Coburn KL: Dimensional analysis of topographic EEG: some methodological considerations, in Measuring Chaos in the Human Brain. Edited by Duke DW, Pritchard WS. Singapore, World Scientific Press, 1991, pp 181-198Google Scholar
15 Pritchard WS, Duke DW, Coburn KL, et al: Nonlinear dynamical EEG analysis applied to nicotine psychopharmacology and Alzheimer's disease, in The Biology of Nicotine. Edited by Lippiello P, Collins A, Gray J, et al. New York, Raven Press, 1992, pp 195-214Google Scholar
16 Pritchard WS, Duke DW, Coburn KL, et al: EEG-based, neural-net predictive classification of Alzheimer's disease versus control subjects is augmented by nonlinear EEG measures. Electroencephalogr Clin Neurophysiol 1994; 91:118-130Crossref, Medline, Google Scholar