Limb Misidentification: A Clinical-Anatomical Prospective Study
Abstract
The misidentification of one’s own limb (LM) after right hemisphere stroke is a striking phenomenon that is incompletely understood. The authors prospectively studied the natural history and anatomy of LM in 36 patients with hyperacute right middle cerebral artery infarct. Unlike in previous studies, rapid clinical assessment was prioritized. The authors found LM to be common and transient, involving 61% at onset, evolving to 15% at 1 week. Voxel-based lesion-symptom mapping found supramarginal gyrus (SMG) damage associated with LM. This substantiates the SMG’s importance in LM and has broader implications for lesion analysis: timing matters. Rapid assessment of transient disorders minimizes false negatives, which can improve lesion analysis.
The misidentification of one’s own limb is a striking clinical phenomenon: when shown their left hand, patients with right hemispheric lesions may claim it is not their own, but someone else’s. This disorder of bodily awareness has captured the interest of neurologists for over a century. Yet, controversy remains over the pathogenesis and underlying neurological basis of the disorder.
Following neurological damage, parts of the body can be ignored, forgotten, misperceived, denied, or misidentified.1 Asomatognosia serves as the general heading for these disorders of bodily awareness, while the subheading somatoparaphrenia has been used to denote specific patients with the tendency to attribute parts of their own body to someone else.2 The taxonomy of these disorders has been difficult to systematize, and consequently structure function correlations have suffered inconsistencies.1,2 Because limb misidentification (LM) is our core focus of interest, we use this term henceforth for simplicity’s sake.
The era of hyperacute stroke treatment (<6 hours) affords a fresh perspective on neurobehavioral disorders like LM that were classically described in the subacute-chronic phase.3 This is because hyperacute phenomenology, which often remits after days, can be observed hours after stroke and followed daily in the stroke unit. This can help elucidate the core features and recovery trajectory of a syndrome. Moreover, it can also serve to clarify structure function correlations, as brain reorganization, which can mask the default functions of brain areas, has not yet occurred.4
Acute right hemisphere stroke often produces compelling clinical manifestations that rapidly improve.5 One such manifestation is anosognosia, the unawareness of sudden onset hemiplegia (AHP), a disorder frequently associated with LM.6 Vocat et al.5 recently showed the prevalence of AHP is close to 60% at the onset of stroke and then evolves with rapid improvement to 32% within the first 3 days, and 18% at 1 week’s time. This study exposed the perils of lesion-symptom analysis in the acute phase: with each day that passes, the prevalence of target syndrome decreases.
Thus, patients with AHP for 3 days poststroke may be misclassified as not having the disorder if first examined on day 4, simply because they improved by the time of examination. The sensitivity of the neurological examination detecting an acute disorder like AHP or LM is time dependent, and the steep slope of decaying prevalence is likely to be a major confounder in lesion analysis4; this may be a reason why recent anatomical studies of LM have produced divergent results.2
Of these studies, Baier and Karnath7 found LM related to right posterior insular damage; Gandola et al.6 reported a combination of cortical and subcortical lesions; and Feinberg et al.8 reported extensive parietal-temporal regions as well as frontal areas involved. Notably, the negative control groups for these studies were reported as follows: Baier and Karnath7 examined controls at a median of 3 days, and as far out as 9 days; the control group examined by Gandola et al.6 was retrospective and first examined at a mean of 6+/−5 days poststroke; and the control group reported by Feinberg et al.8 was examined within 1 week, number of days not specified. For transient disorders like LM, the more days that pass before first examination, the greater the chance the control group will be contaminated with false negatives.
Thus, time to first examination is a potential confounder that needs to be taken into account in lesion analysis.4 This may be the reason why the findings in Feinberg et al.’s9 original work showed a different distribution of critical lesions than the aforementioned studies. Feinberg et al.9 implicated the right supramarginal gyrus and corona radiata in the genesis of asomatognosia and included a rapid assessment of controls, with a mean time to first exam of 1.3 days.
Given this, we sought to refine the current understanding of LM. We prospectively studied the natural history of the disorder, which hitherto had never been investigated, by examining patients soon after stroke onset and daily thereafter, in order to determine the disorder’s daily prevalence. We then performed a voxel-wise lesion-symptom analysis which, unlike previous studies, prioritized the time to first examination to ensure a relatively uncontaminated control group.
Methods
Patients
We prospectively screened consecutive patients with both 1) acute left hemiparesis and 2) acute spatial neglect admitted to Montefiore Medical Center, Bronx, N.Y., for a 5-year period (September 2010–March 2016). These screening criteria were chosen to ensure that the symptomatic (LM+) and control groups (LM−) were well matched along crucial clinical variables: the presence of hemiparesis and spatial neglect. Moreover, to avoid anatomical confounders related to stroke etiology, we included only patients with lesions in the same vascular distribution: ischemic stroke of the right middle cerebral artery (MCA) territory. We excluded patients with lacunar stroke, hemorrhagic stroke, prior ipsilateral stroke, and inability to participate due to aphasia or inattention. These criteria resulted in a group of 36 out of 64 patients. Informed consent was obtained for all patients enrolled in the study.
The time to first examination was a crucial aspect of this study: we examined patients in the hyperacute-acute phase of stroke, typically hours after stroke onset. Because LM is a transient phenomenon, we wanted to make sure the control LM– group included true negatives and was not contaminated with LM+ patients who simply improved by the time of a first examination. Therefore, we only included patients in our LM– control group who were examined within 36 hours of stroke. This 36-hour ceiling ensured a similar sample to the controls in Feinberg et al.’s9 study (1.3 days), and took into account the AHP prevalence decay rate reported by Vocat et al.5 Time to first examination was less restrictive in the LM+ patients.
Procedure
All patients underwent a battery of tests: the standard neurological examination, NIH Stroke Scale, visual-spatial neglect testing, and tests of bodily awareness.
Spatial neglect was assessed using the NIH Stroke Scale10 and a letter version of the Albert cancellation.11 A deficit found on either test was used as an indication of the presence of neglect. Patients with an NIH neglect score of 1 or 2 were considered to have neglect: 1=visual, tactile, auditory, spatial, or personal inattention or extinction to bilateral simultaneous stimulation in one of the sensory modalities; 2=profound hemi-inattention or extinction to more than one modality; does not recognize own hand or orients to only one side of space (http://www.ninds.nih.gov/doctors/NIH_Stroke_Scale.pdf). The modified Albert test consisted of 10 target letter As among 45 irrelevant letters; one or more target omission differences between left and right was used as an indication of the presence of visual-spatial neglect. We did not examine patients for motor neglect.
AHP was examined using Bisiach’s 4-point scale12: grade 0 (no anosognosia), the hemiparesis is spontaneously reported by the patient following a general question about complaints; grade 1, the hemiparesis is reported only following a specific question about the strength of the patient’s limbs; grade 2, the hemiparesis is acknowledged only after demonstrations through routine techniques of neurological examination; grade 3, no acknowledgment of the hemiparesis can be obtained. Patients who scored grades 2 or 3 were considered to have AHP.
Limb identification was determined as follows. The examiner lifted the left arm by the elbow, and moved the patient’s hand and forearm into the ipsilesional hemispace. The patient was then asked, “Whose hand is this?” A patient was judged to have limb misidentification (LM+) if ownership of the limb was incorrectly reported. Care was taken to keep the examiner’s hand and arm out of the patient’s ipsilateral hemispace.
After first examination, those patients who displayed LM were then re-examined daily or as close to daily as possible (given clinical circumstances) until the day they correctly identified their limb or until 7 days passed, whichever came first. Only two patients with LM did not receive a follow-up exam.
Statistical Analysis
To analyze relationships among clinical and neuropsychological variables, nonparametric continuous variables were compared by the Mann-Whitney U test and categorical variables were compared with Fisher’s exact test.
Lesion Analysis
Acute MRI scans were available for 24 patients and CT scans for the remaining 12. The mean time between stroke onset and scan was 1.3 days (SD=0.47) in the LM– control group and 2.1 days (SD=1.7) in the LM+ group.
Lesion borders were manually drawn directly on the individual MRI or CT scans using MRIcron software.13 Subsequently, both the anatomical scan and the drawn lesion volume were normalized to a standard brain template using the Clinical Toolbox for normalization (http://www.mccauslandcenter.sc.edu/crnl/tools) using the Statistical Parametric Mapping 8 software package (http://www.fil.ion.ucl.ac.uk) implemented in Matlab (http://www.mathworks.com). This validated procedure allows for group comparison consisting of MRI and CT lesions that are mapped and reconstructed onto a common stereotaxic space.14 Because CT has a lower sensitivity for stroke detection than MRI, it is possible that lesion maps created from CT scans did not capture infarcted tissue as well as those based on MRI. Despite this limitation, including CT scans in the study was unlikely to introduce systemic bias in the lesion analysis as the CT scans were evenly distributed between the groups (LM+: eight of 22 scans; LM–: four of 14 scans).
After normalization, all lesions were visually inspected to ensure accuracy of registration into common space. These normalized lesions were then used for voxel-based statistical analysis using the Non-Parametric Mapping software package (http://www.cabiatl.com/mricro/npm/). In this analysis with one dependent variable (LM) and one independent variable (lesion status), a chi-squared Leibermeister test was performed at each voxel, comparing scores on the dependent variable between patients with and without lesions in that voxel.15 The resultant z-scores are coded along a corresponding color scale mapped onto the standardized anatomical brain template.16
Results
We found 61% (22/36) of right MCA infarct patients had LM within the first 36 hours of stroke. As time elapsed, prevalence declined to 44% at 3 days and 15% at 1 week’s time. Two patients in the LM+ group did not have follow-up exams, and thus the prevalence at day 3 and 1 week are based on 34 patients.
Of the LM+ patients, 77% (15/22) provided the answer of “yours” or “the doctor’s” as the owner of their left hand. One patient stated, “I don’t know.” Twenty-three percent (5/22) identified the limb as someone else’s other than the doctor. Of these five patients, one patient displayed misoplegia (“the other guy that I don’t like, the other me”), two patients identified the limb as belonging to a family member (two sons, one daughter), and one had a fixed delusion over days (“a man’s hand was on my chest last night I had to throw it off, a wicked man comes here every night”); of these five patients, two also simultaneously claimed the hand was “the doctor’s.” One patient’s misidentification was not recorded. Limb misidentification transitioned to the correct identification for 17 patients within 1 week; these patients simply identified the correct owner of the limb on the day of remission.
Table 1 shows that AHP and NIH Stroke Scale scores were significantly correlated with LM. The presence of visual-spatial neglect, paresis, or sensory deficits did not differ significantly between the groups.
Characteristic | Normal Limb Identification | Limb Misidentification | p |
---|---|---|---|
Number | 14 | 22 | |
Sex (female) | 6 | 16 | 0.092b |
Age (median [range]) | 55 (22–93) | 67.5 (42–90) | 0.172c |
Handedness (right) | 14 | 18 | 0.141b |
Hours to first examination (median [range]) | 22.5 (1–36) | 24 (1–96) | 0.445c |
Paresis | |||
% Present | 85 | 100 | 0.144b |
Presence of neglect | |||
% NIHSS neglect scale | 100 | 100 | |
% Letter cancellation | 85 | 100 | 0.144b |
Anosognosia | |||
% Present | 50 | 91 | 0.001b |
% Testing not possible | 0 | 9 | |
Sensory deficit | |||
% Present | 78 | 91 | 0.061b |
% Testing not possible | 0 | 9 | |
NIHSS [median (range)] | 10.5 (3–19) | 17 (7–20) | 0.026c |
TABLE 1. Demographic and Clinical Dataa
Lesion Mapping
Figure 1 illustrates the lesion density plots for each group, LM+ and LM–. To identify the structures specifically damaged in patients with LM, we performed a Leibermeister (chi-squared) test at each voxel, which compared the scores of the dependent variable (presence or absence of LM) between patients with and without lesions in that voxel. Figure 1 shows the brain regions significantly associated LM. These voxels survived to the statistical threshold of p<0.01 (critical z-value >3.65), false discovery rate corrected for multiple comparison.
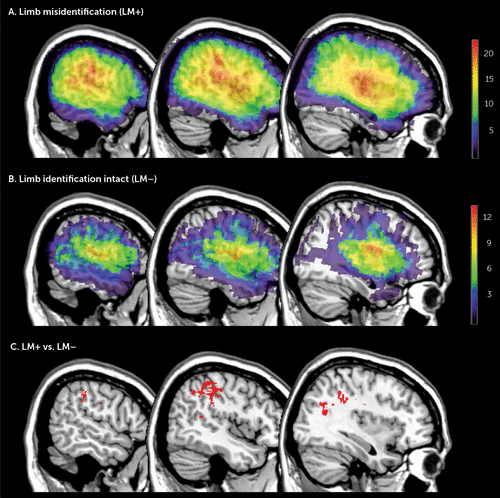
FIGURE 1. Lesion Analysisa
a A) Overlay lesion plots of the patients with limb misidentification (LM+) (N=22). B) Overlay lesion plots of patients with normal limb identification (LM–) (N=14). The number of overlapping lesions is illustrated by different colors coding increasing frequencies from violet to red. C) Anatomical comparison between LM+ and LM− patients. Shown are the brain regions significantly associated with LM+ as identified by the Liebermeister test. The voxels shown in red survived to the statistical threshold of p<0.01 (critical z-value >3.65), false discovery rate corrected for multiple comparison. The largest concentration was located in the supramarginal gyrus and its underlying white matter. Additional voxels are also seen in the postcentral gyrus and middle and superior temporal gyrus. Montreal Neurological Institute x-coordinates: x=56, x=46, x=36.
The largest concentration was located in the inferior parietal lobe, specifically in the supramarginal gyrus and extending to the underlying white matter of the parietal lobe. Additional voxels located in the postcentral gyrus and middle and superior temporal gyrus were also found.
Discussion
Our study is the first to investigate the natural history of limb misidentification. We assessed patients soon after stroke onset and then repeatedly examined them over the ensuing days. The results show that LM is a common and transient phenomenon, involving 61% of right MCA stroke patients at onset and then rapidly evolving to a prevalence of 44% at 3 days and 15% at one week’s time. This is a remission rate similar to that reported for the related disorder AHP.5 Spontaneous biological recovery begins in the first hours and days after stroke, and our results offer a rare glimpse at the trajectory of recovery from a particular body perceptual disorder.17
Our lesion analysis prioritized time to first examination. We report the supramarginal gyrus and its underlying white matter as the most commonly lesioned region in patients with right MCA stroke who misidentify their contralesional limb. This lesion location closely tracks with the results of Feinberg et al.9 Both studies had similar mean time to first examination (present study: 24 hours; Feinberg et al. study: 1.3 days) and only included right MCA ischemic infarcts. The reproduction of these results substantiates the importance of the right supramarginal gyrus in LM and also underscores the importance of rapid assessment and consistent lesion etiology in lesion analysis.
While these results are important for understanding the neurological basis of LM, they also have broader implications on lesion analysis: timing matters. As time elapses after stroke onset, deficit prevalence decreases. The sensitivity of the neurological examination to detect a deficit is time dependent, and thus delayed time to first examination increases false negatives, confounding lesion analysis. Future lesion studies may benefit from taking into account the trajectory of spontaneous biological recovery of the target deficit.
The Supramarginal Gyrus and Limb Misidentification
Converging lines of evidence implicate an important role for the right SMG in the perception and awareness of the body. Cortical stimulation of the human right SMG produces responses of somatosensory sensations, sensory illusions, and body schema alterations.18 Lesions of the right SMG have been shown to be associated with disrupted bodily awareness (personal neglect) as opposed to visual neglect.19
Moreover, recent human and animal work on this region bears a direct relationship to the clinical syndrome of LM. Electrophysiological recordings in monkeys have revealed neurons with multimodal receptive fields in the parietal cortex, which encode the seen and felt position of the upper limb.20 In humans, fMRI studies have implicated the right SMG as the likely human site of this kind of visual and proprioceptive integration.21
Lesions that cause dysfunction at this level of integration may lead to a distorted body perception and awareness. Even in the nonlesioned brain, integrating bodily sensations is a challenge as body posture is always changing. The brain solves this challenge by constantly transforming tactile stimuli from locations on the body surface to coordinates in external space.22,23 The coordinate system for this spatial perception is based on the integration of multisensory information: vision, proprioception, and tactile sensation.22,23 This system, in which the right SMG is critically involved, is not only important for conscious body perception but also critical for the sensation of body ownership (the subjective experience of the body as one’s own).24,25
Thus, patients with varying degrees and combinations of proprioceptive deficit, tactile loss, spatial inattention (neglect), and multisensory disintegration from right hemisphere damage may show a range of phenomenology: from perceptual distortions (i.e., “the left arm feels detached”), to nonverbal behavioral manifestations (i.e., failure to dress the left arm or ignoring it as it dangles into the spokes of a wheelchair), to manifestations in the verbal domain (denying ownership of the left arm or claiming it is someone else’s).1,2,9 Altered body perception and awareness from a damaged multisensory integration system forms the basis of LM and the spectrum of body ownership disorders.2,25 It is difficult, however, to explain manifestations in the verbal domain solely on the basis of right hemisphere damage without considering how this altered multisensory information might be dealt with by language cortex. Under this consideration, the left hemisphere may be fulfilling its natural tendency to assign a coherent verbal explanation of events (e.g., “the hand is yours, not mine”) in the setting of distorted, impoverished, or absent multisensory left body perception (from right hemisphere damage).26
Our finding of the SMG being the most commonly lesioned site in LM from right MCA stroke comports well with the known fundamental behavioral architecture of the brain. It is a multimodal association cortex that is critical for the perception of the complex contents of bodily experience and is also a gateway for those experiential contents to be available to the left hemisphere for verbal description.27
1 : Asomatognosia, in The Behavioral and Cognitive Neurology of Stroke, by Godefroy O. Edited by Bogousslavsky J. Cambridge, Cambridge University Press, 2007, pp 215–253Crossref, Google Scholar
2 : Somatoparaphrenia: a body delusion. A review of the neuropsychological literature. Exp Brain Res 2009; 192:533–551Crossref, Medline, Google Scholar
3 : Cognitive Deficits in Hyperacute Stroke. Stroke 2004; 35:25Crossref, Google Scholar
4 : Using human brain lesions to infer function: a relic from a past era in the fMRI age? Nat Rev Neurosci 2004; 5:813–819Crossref, Medline, Google Scholar
5 : Anosognosia for hemiplegia: a clinical-anatomical prospective study. Brain 2010; 133:3578–3597Crossref, Medline, Google Scholar
6 : An anatomical account of somatoparaphrenia. Cortex 2012; 48:1165–1178Crossref, Medline, Google Scholar
7 : Tight link between our sense of limb ownership and self-awareness of actions. Stroke 2008; 39:486–488Crossref, Medline, Google Scholar
8 : The neuroanatomy of asomatognosia and somatoparaphrenia. J Neurol Neurosurg Psychiatry 2010; 81:276–281Crossref, Medline, Google Scholar
9 : Verbal asomatognosia. Neurology 1990; 40:1391–1394Crossref, Medline, Google Scholar
10 : Improved reliability of the NIH Stroke Scale using video training. Stroke 1994; 25:2220–2226Crossref, Medline, Google Scholar
11 : A simple test of visual neglect. Neurology 1973; 23:658–664Crossref, Medline, Google Scholar
12 : Unawareness of disease following lesions of the right hemisphere: anosognosia for hemiplegia and anosognosia for hemianopia. Neuropsychologia 1986; 24:471–482Crossref, Medline, Google Scholar
13 : Stereotaxic display of brain lesions. Behav Neurol 2000; 12:191–200Crossref, Medline, Google Scholar
14 : Age-specific CT and MRI templates for spatial normalization. Neuroimage 2012; 61:957–965Crossref, Medline, Google Scholar
15 : Improving lesion-symptom mapping. J Cogn Neurosci 2007; 19:1081–1088Crossref, Medline, Google Scholar
16 : Voxel-based lesion-symptom mapping. Nat Neurosci 2003; 6:448–450Crossref, Medline, Google Scholar
17 : Understanding upper limb recovery after stroke. Restor Neurol Neurosci 2013; 31:707–722Medline, Google Scholar
18 : Multimodal responses induced by cortical stimulation of the parietal lobe: a stereo-electroencephalography study. Brain 2015; 138:2596–2607Crossref, Medline, Google Scholar
19 : Neural bases of personal and extrapersonal neglect in humans. Brain 2007; 130:431–441Crossref, Medline, Google Scholar
20 : Coding the location of the arm by sight. Science 2000; 290:1782–1786Crossref, Medline, Google Scholar
21 : Integration of visual and proprioceptive limb position information in human posterior parietal, premotor and extrastriate cortex. J Neurosci 2016; 36:2582–2589Crossref, Medline, Google Scholar
22 : Crossing the hands increases illusory self-touch. PLoS One 2014; 9:e94008Crossref, Medline, Google Scholar
23 : From maps to form to space: touch and the body schema. Neuropsychologia 2010; 48:645–654Crossref, Medline, Google Scholar
24 : The vestibular system: a spatial reference for bodily self-consciousness. Front Integr Neurosci 2014; 8:31Crossref, Medline, Google Scholar
25 : Visual consciousness and bodily self-consciousness. Curr Opin Neurol 2015; 28:23–28Crossref, Medline, Google Scholar
26 : Cerebral specialization and interhemispheric communication: does the corpus callosum enable the human condition? Brain 2000; 123:1293–1326Crossref, Medline, Google Scholar
27 : From sensation to cognition. Brain 1998; 121:1013–1052Crossref, Medline, Google Scholar