Central Executive Network and Executive Function in Patients With Alzheimer’s Disease and Healthy Individuals: Meta-Analysis of Structural and Functional MRI
Abstract
Objective:
The neural architecture of executive function is of interest given its utility as a transdiagnostic predictor of adaptive functioning. However, a gap exists in the meta-analytic literature assessing this relationship in neuropsychiatric populations, concordance between structural and functional architecture, and the relationship with neuropsychological assessment of executive function. Given the importance of the central executive network (CEN) in Alzheimer’s disease, this population may be useful in understanding this relationship in Alzheimer’s disease pathology.
Methods:
A meta-analysis of studies (k=21) was conducted to elucidate the relationship between executive function and CEN for structural architecture (k=10; N=1,027) among patients with Alzheimer’s disease (k=6; N=250) and healthy control subjects (HCs) (k=4; N=777) and for functional architecture (k=11; N=522) among patients with Alzheimer’s disease (k=6; N=306) and HCs (k=5; N=216). Random-effects modeling was used to increase accuracy of conclusions about population means.
Results:
Analyses revealed a positive brain-behavior relationship (pr=0.032, 95% CI=0.07, 0.54), although there was a lack of statistically significant heterogeneity between functional and structural neuroimaging (Q=9.89, p=0.971, I2=0.00%) and between the Alzheimer’s and HC groups in functional (Q=8.18, p=0.612, I2=0.00%) and structural (Q=1.60, p=0.996, I2=0.00%) neuroimaging. Similarly, a lack of statistically significant heterogeneity was revealed between functional and structural neuroimaging among patients with Alzheimer’s disease (Q=3.59, p=0.980, I2=0.00%) and HCs (Q=3.67, p=0.885, I2=0.00%).
Conclusions:
Structural and functional imaging in the CEN are predictive of executive function performance among patients with Alzheimer’s disease and HCs. Regardless of how the CEN is affected, behavior is correlated to the degree to which the CEN is affected. Findings are significant in the context of methodological decisions in multimodal neuroimaging research.
The neural architecture of executive function is of considerable interest given the strong clinical utility of executive function as a transdiagnostic predictor of adaptive functioning. Executive function is a cognitive process that promotes planning, coordinating, and regulation of higher order behavior. Previously, executive function has been defined as a set of distinct skills (1), a general unitary factor (2), or reflecting both unitary and diverse influences (3), supported by factor analytic and imaging studies (4, 5). Thus, there is debate regarding the construct of measurement in executive function tasks, which is important for contextualizing the executive function brain-behavior relationship. Historically, executive function has been associated with the frontal lobe and validated by lesion studies (6), and it has recently been associated with the central executive network (CEN), with its central hubs in the dorsolateral prefrontal cortex and posterior parietal cortices, via functional neuroimaging research (7). The CEN is a key intrinsic connectivity network whose functions are similar to brain functions revealed through study of the effects of frontal and parietal lobe lesions. Consistent evidence supports the sensitivity of various neuropsychological executive function tasks to the presence of brain damage, but the degree to which these abilities can be localized to the prefrontal cortex in the context of lesion studies is less clear (8). Given this high variability in the understanding of executive function, a meta-analysis may be useful to consolidate and interpret the range of results.
There is considerable debate about the structural and functional organization of executive function that parallels that about the functional heterogeneity of the prefrontal cortex (7). For instance, little evidence supports focalized organization of the prefrontal cortex for executive function as measured by clinical neuropsychological tasks, although some are more closely linked to brain volume than others (7). In addition, in a meta-analysis, larger cortical volume and thickness of the prefrontal cortex have been associated with better executive function task performance among healthy adults (9). Together, these studies provide inconsistent support for the specific organization of the prefrontal cortex as important for executive function.
Contemporary views highlight a systems-level approach to executive function rather than a one-to-one brain-behavior relationship (6). For example, among psychiatric patients, executive deficits have been observed to be the result of multiple attentional circuits connecting frontal and posterior regions that are adaptive to multiple contexts (8). Thus, the literature suggests that executive function operates within a system that monitors this function and allows individuals to apply general strategies to new situations; this system has been identified as a general-purpose central executive (10, 11) or a supervisory attentional system (12).
Stemming directly from these seminal works, contemporary views indicate that executive function works as a unified functional system in the CEN, a functional brain network with central hubs in the prefrontal and posterior parietal cortices. Specifically, the CEN links the dorsolateral frontal (i.e., bilateral dorsolateral prefrontal cortex, ventrolateral prefrontal cortex, dorsomedial prefrontal cortex) and parietal cortices, because these are linked to behavioral correlates, including working memory and control processes (13). As such, the CEN is evidenced to play a critical role in executive function, such as goal-directed behavior, impulsivity, and the maintenance and manipulation of information for working memory (13). The brain is a complex, intertwined system, and executive function is a broad construct, and thus the systems-level approach highlighting the CEN as the localization for executive function may be more promising than structurally localizing executive function solely in the prefrontal cortex.
Recent scientific advances provide insight into the functional organization of executive function linked to regional networks (14), yet there is debate regarding specific constructs measured by executive function tasks, important for contextualizing the relationship between executive function tasks and the neural organization of the brain. The literature broadly supports that adaptive functions of executive function are used in conjunction with one another and exist as part of a larger system of cognitive control. Executive function is classically observed via neuropsychological and neurocognitive tasks, which are under scrutiny regarding their utility in capturing executive function constructs such as formulating goals, planning, and executing tasks because they often assess several cognitive domains at once (14). Challenges in measuring executive function, along with the complexity of executive function as a construct, directly relate to the difficulty in localizing the structural and functional organization of executive function. Examining these relationships in the context of both healthy and neuropsychiatric populations can clarify how these tasks and brain organization are linked and the comparative contribution of cortical morphometry and functional networks for tasks of executive function for these two groups.
Executive function deficits are prominent in a range of psychiatric diagnoses, including degenerative disorders such as Alzheimer’s disease. Compromised executive function is associated with lowered instrumental activities of daily living (15), general cognitive decline (16), and increased mortality (17), validating the strength of executive function as a transdiagnostic predictor of important clinical outcomes. There is a gap in the meta-analytic literature assessing this relationship among neuropsychiatric populations, the concordance between structural and functional architecture, and the relationship with neuropsychological assessment of executive function.
Although episodic memory impairment is predominant among people with Alzheimer’s disease, working memory impairment and executive dysfunction contribute significantly to IADL impairment. Even after accounting for degree of memory deficit, executive dysfunction is a key contributor to IADL impairment (18). Seminal work in this area highlights how individuals with Alzheimer’s disease show impaired central executive functioning, reflected in their ability to consecutively perform two concurrent tasks (11). More important, individuals in the later stages of Alzheimer’s disease, who are observed to have higher levels of atrophy, also have lower executive function performance (19) and likely experience more day-to-day functional impairment. Because the CEN has been identified as a site of pathology in Alzheimer’s disease, this population is useful in understanding the relationship between the structural and functional architectures of executive function and their relationship with executive function tasks. Therefore, this study aimed to assess the relationship between structural and functional imaging in central hubs of the CEN and performance on executive function tasks among both Alzheimer’s disease and healthy control (HC) populations by addressing the following research questions:
Research Question 1: Is there a positive association between functional signal strength and better performance on executive function tasks?
Research Question 2: Is there a positive association between increased cortical volume and thickness and better performance on executive function tasks?
Research Question 3: Is there a difference in the magnitude of effect sizes between the HC and Alzheimer’s disease groups?
Research Question 4: Is there a difference in the magnitude of effect sizes between functional and structural neuroimaging modalities?
Methods
Protocol Registration
Preferred Reporting Items for Systematic reviews and Meta-Analyses (PRISMA) guidelines were followed in the creation of the protocol for this study (20), which is registered under the National Institute for Health Research’s International Prospective Register of Systematic Reviews (PROSPERO) (identifier CRD42020141544). PRISMA and PROSPERO promote transparency in research and adherence to pre hoc analytic plans in systematic reviews and meta-analyses.
Selection of Studies
Four separate literature searches of the PubMed and EBSCO databases (including PsycINFO and PsycARTICLES) were conducted in May 2019. Terms for Alzheimer’s disease structural studies were as follows: (dorsolateral OR dorsal lateral OR BA9 OR BA46 OR BA8 OR BA10 OR posterior parietal OR parietal lobule OR BA5 OR BA7 OR BA49 OR BA30) AND (volume* OR atrophy OR cortical thickness OR cortical thinning OR morphometry) AND (executive OR card sort* OR color word OR Stroop OR trail* OR verbal fluency OR working memory) AND (Alzheimer* OR Alzheimer* disease OR AD OR dementia). Terms for HC structural studies were as follows: (dorsolateral OR dorsal lateral OR BA9 OR BA46 OR BA8 OR BA10 OR posterior parietal OR parietal lobule OR BA5 OR BA7 OR BA49 OR BA30) AND (volume* OR atrophy OR cortical thickness OR cortical thinning OR morphometry) AND (executive OR card sort* OR color word OR Stroop OR trail* OR verbal fluency OR working memory) AND (healthy). In total, 655 (238 Alzheimer’s disease, 417 HC) structural studies were assessed for inclusion.
Alzheimer’s disease functional terms were as follows: (central executive OR frontal executive OR frontal parietal) AND (functional MRI OR fMRI OR functional connectivity OR BOLD) AND (executive OR card sort* OR color word OR Stroop OR trail* OR verbal fluency OR working memory) AND (Alzheimer* OR Alzheimer* disease OR AD OR dementia). HC functional terms were as follows: (central executive OR frontal executive OR frontal parietal) AND (functional MRI OR fMRI OR functional connectivity OR BOLD) AND (executive OR card sort* OR color word OR Stroop OR trail* OR verbal fluency OR working memory) AND (healthy). In total, 1,832 (489 AD, 1,343 HC) functional studies were assessed for inclusion.
Inclusion Criteria
Studies were included if they contained one or more structural neuroimaging measures of gray matter volume, thickness, or morphometry in the regions of interest or functional neuroimaging of either task or resting state signal strength in the central hubs of the CEN (i.e., dorsolateral prefrontal cortex and posterior parietal cortex). In this study, the dorsolateral prefrontal cortex was defined using Brodmann’s areas (BAs) 9, 46, 8, and 10, and the posterior parietal lobe was defined using BAs 5, 7, 49, and 30 (Figure 1). Although these regions are often activated and associated with various networks, there is a dearth of evidence supporting the idea that parts of the dorsolateral prefrontal cortex are functionally isolated. Therefore, although these structural regions are not unique to the CEN, they are most commonly associated with the CEN and are consistently defined as central hubs of the CEN. Thus, they were used to capture a broad range of studies that associated executive function task performance with neuroimaging of these regions. Cortical thickness and volume were collapsed into a single indicator for analyses because previous meta-analysis has shown that their effect sizes are equivalent (9). Inclusion criteria indicated that studies must contain one or more measures of executive function and required statistics exhibiting the relationship between executive function and either structural or functional imaging modality. For some studies, the executive function-CEN association was a core focus; for others, that association was embedded in the broader study context. Studies were included if they reported one or more of the following: correlation coefficients (Pearson’s r or r2, Fischer’s Z), standardized β coefficients (−0.50 > β >0.50), t statistics, or p values. For β coefficients and partial correlations, standard error was also required for inclusion. When neuroimaging and executive function task performance were collected but no association between the two was reported, the corresponding author for each study was contacted to request the information. For the purposes of this study, case studies, research with nonhuman subjects, and research with human participants with a mean age younger than 50 years were excluded from the analyses. For studies that contained both populations, Alzheimer’s disease and HC groups were used separately.
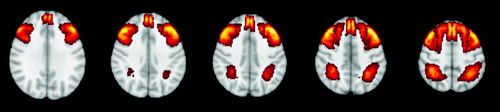
FIGURE 1. Central hubs used to define the central executive network (CEN)a
a The central hubs of the CEN were defined as the dorsolateral prefrontal cortex (Brodmann’s areas [BAs] 9, 46, 8, and 10) and the posterior parietal lobe (BAs 5, 7, 49, and 30). A Montreal Neurological Institute map (generated in FMRIB Software Library) of the CEN is shown as defined in this study.
The search items described earlier produced 2,487 research items in total. Trained graduate research assistants systematically excluded articles on the basis of inclusion and exclusion criteria. All titles and abstracts were examined to determine their appropriateness for the present study, and studies were retained for further consideration. Of the 727 Alzheimer’s disease studies assessed, 715 were excluded on the basis of the inclusion criteria, leaving 12 total Alzheimer’s disease studies (six functional and six structural). Of the 1,760 HC studies assessed, 1,751 were excluded on the basis of the inclusion criteria, leaving nine total HC studies (five functional and four structural) for the final analyses. Notably, many of the studies initially identified through our broad search criteria did not meet the inclusion criteria, primarily because of a lack of statistical data provided for the relationship between imaging and executive function task performance. (See Figure 2 for an eligibility flow diagram and Table 1 for details on the articles included in this study.)
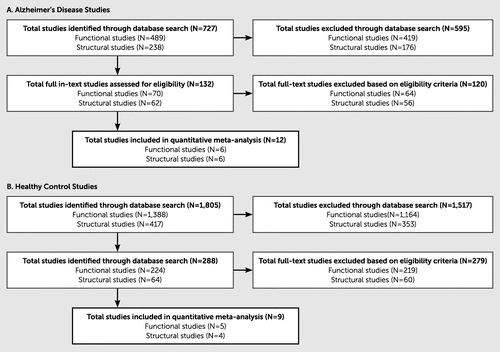
FIGURE 2. Study eligibility flow diagrama
a The study flow diagram (adapted from Moher et al. [20]) includes structural and functional neuroimaging studies in the Alzheimer’s disease group and structural and functional neuroimaging studies in the healthy control group.
Age (years) | Number of variables controlled | ||||||||
---|---|---|---|---|---|---|---|---|---|
Variable | Sample size | Mean | SD | Female (%) | Imaging modality | Brain region | Task | Coefficient | |
Healthy control subjects | |||||||||
Structural | |||||||||
Cox et al. (27) | 90 | 70 | — | 0 | GM | Prefrontal cortex | EFC | r=0.17 | 1 |
Vonk et al. (28) | 505 | 74.1 | 5.8 | 55.6 | Thickness | Prefrontal cortex | VF | t=2.8 | 5 |
Drobetz et al. (29) | 40 | 73.7 | 7.7 | 65 | Thickness | Prefrontal cortex | Delayed discounting | r=0.40 | 2 |
Weinstein et al. (30) | 142 | 66.4 | 5.5 | 64.1 | GM | Prefrontal cortex | CWI | r=0.128 | 4 |
Functional | |||||||||
Methqal et al. (31) | 20 | 69.45 | 4.54 | 85 | fMRI | Prefrontal cortex and parietal cortex | VF | r=–0.1395 | 2 |
Chen et al. (32) | 77 | 67.66 | 5.69 | 51.9 | rfMRI | Prefrontal cortex | WM | r=0.35 | 3 |
Riekmann et al. (33) | 18 | 70.33 | 3.25 | 50 | fMRI | Prefrontal cortex and parietal cortex | WM | r=–0.491 | 0 |
Gold et al. (34) | 34 | 77.03 | 7 | 55.9 | fMRI | Prefrontal cortex | WM | r=0.026 | 3 |
Zhang et al. (35) | 67 | 79.5 | 4.2 | 52.2 | rfMRI | Prefrontal cortex and parietal cortex | VF | r=0.164 | 0 |
Alzheimer’s disease | |||||||||
Structural | |||||||||
Heuer et al. (36) | 28 | 60.9 | 1.65 | 42.9 | Thickness | Prefrontal cortex | EFC | r=0.34 | 1 |
Chang et al. (37) | 104 | 72.97 | — | 49 | Thickness | Prefrontal cortex | EFC | r=0.23 | 2 |
Foxe et al. (38) | 21 | 63.7 | 6.4 | 33.3 | Thickness | Parietal cortex | WM | r=0.00 | 2 |
Vasconcelos et al. (39) | 19 | 75.42 | 4.81 | 52.6 | GM | Parietal cortex | CWI | B=–0.093, t=–0.359 | 4 |
Possin et al. (40) | 46 | 65.5 | 9.7 | 41.3 | Thickness | Prefrontal cortex and parietal cortex | Figure copy | r=0.39 | 5 |
Wong et al. (41) | 32 | 63.53 | 6.98 | 37.5 | Thickness | Prefrontal cortex and parietal cortex | EFC | t=2.89 | 2 |
Functional | |||||||||
Chang et al. (42) | 91 | 71.61 | — | 42.9 | rfMRI | Prefrontal cortex and parietal cortex | EFC | r=0.18 | 2 |
Vipin et al. (43) | 94 | 77.25 | — | 66 | fMRI | Prefrontal cortex and parietal cortex | EFC | r=0.32 | 6 |
Wang et al. (44) | 32 | 71.25 | 8.63 | 56.3 | rfMRI | Prefrontal cortex and parietal cortex | EFC | t=3.22 | 3 |
Li et al. (45) | 22 | 61.5 | 4.55 | 72.7 | rfMRI | Parietal cortex | TMT | r=0.67 | 4 |
Ranasinghe et al. (46) | 27 | 63.2 | 7.1 | 59.3 | rfMRI | Prefrontal cortex and parietal cortex | EFC | r=0.65 | 1 |
Agosta et al. (47) | 13 | 74.5 | 9.7 | 38.5 | rfMRI | Prefrontal cortex and parietal cortex | TMT | r=0.60 | 3 |
TABLE 1. Demographic and clinical characteristics of patients with Alzheimer’s disease and healthy control subjectsa
Variable Coding
Trained graduate research assistants extracted the following variables: year published, sample type (i.e., HC or Alzheimer’s disease), sample size, mean±SD age, age range, sample percentage women, sample percentage left handed, country of origin, executive function task, statistical coefficient between executive function performance and brain imaging, and brain region or regions. Executive function tasks were coded as one of the following: Stroop Color and Word Test, verbal fluency, working memory, Trail-Making Test, Parts A and B, Wisconsin Card-Sorting Test, or an executive function composite score. Studies that reported multiple associations with executive function task or brain regions were collapsed under a single effect size by averaging Fisher’s z-scores for initial analyses. For studies with multiple statistics indicating the association between executive function task and brain region (e.g., with or without covariates), we used the simplest statistic. For example, when the option was a correlation coefficient or a partial correlation coefficient, we used the former. Executive function measures were reverse coded as needed, so that all positive effect sizes indicated that better performance on executive function tasks was associated with larger volume or thickness or greater signal strength in central hubs of the CEN.
Data Extraction
The data extraction procedure was designed by the senior authors (D.A.G.) in collaboration with the primary coders. Titles were reviewed and excluded by A.B.W. and reviewed by K.M.D. with 96.5% agreement. K.M.D. coded abstracts as “excluded,” “included,” or “unsure,” with senior authors serving as secondary reviewers as needed. The “unsure” code was used when the abstract contained insufficient information to determine whether a study should be excluded; therefore, the full article was pulled for in-depth review. A total of 21 studies (11 functional, 10 structural) were retained for further examination and data extraction. For all 21 studies, M.O.P. was trained to extract coded demographic variables.
The 21 studies were divided between K.M.D. and A.B.W. to determine eligibility and extract variables indicating the brain-behavior relationship in each. Both raters independently coded approximately 10% (N=3) of the 21 studies. Cohen’s κ, the preferred statistic for calculating the degree of interrater reliability, was used to determine the agreement between the two raters on coding studies for inclusion in this meta-analysis, and it revealed near-perfect agreement (κ=0.884; 95% CI=0.862, 0.906).
Effect Size Calculation
All correlation coefficients (i.e., r) were transformed using Fisher’s Zr transformation to determine the effect size for each sample. Mean effect size (MES) was computed for groups by weighting (w) each effect size by its sample size. For each MES, a standard error was calculated, and a Z statistic was generated to determine whether the association between executive function and brain imaging was significant. The 95% confidence intervals were calculated using standard errors.
Random-Effects Modeling
Random-effects modeling was used to increase accuracy in conclusions about population means and to generalize findings beyond the present samples. The Meta-Essentials workbooks for partial correlation data (21) were used to conduct random-effects modeling. A Z statistic was generated to determine whether the association between executive function and brain regions was significant in the random-effects model. By using these new weights, standard errors were used to calculate 95% confidence intervals for each group of studies. Significant between-group differences were compared using analysis of variance statistical analysis and determining whether confidence intervals overlapped.
Results
Sample Characteristics
The 10 functional studies comprised 522 participants in total (mean age 72.63 years; females, 55.6%), and the 11 structural studies comprised 509 participants (mean age 71.28 years; females, 49.6%).
fMRI, Resting-State MRI, and Executive Function Task Performance
In the full sample, the average partial correlation between measures of executive function and fMRI-resting state fMRI (rfMRI) signal strength was positive (pr=0.32, Ztest=3.28, p=0.001, 95% CI=0.07, 0.54), indicating that greater signal strength is associated with better executive function task performance. In the sample with Alzheimer’s disease, the average partial correlation between measures of executive function and fMRI-rfMRI signal strength was positive (pr=0.44, Ztest=4.33, p<0.001, 95% CI=0.13, 0.67), indicating that greater signal strength is associated with better executive function task performance. Finally, in the HC sample, the average partial correlation between measures of executive function and fMRI-rfMRI signal strength was positive but not significantly different from zero (pr=0.18, Ztest=1.10, p=0.136, 95% CI=−0.38, 0.65), indicating that greater signal strength is moderately associated with better executive function task performance.
MRI and Executive Function Task Performance
In the full sample, the average partial correlation between measures of executive function and fMRI measures was positive (pr=0.21, Ztest=4.82, p<0.001, 95% CI=0.09, 0.32), indicating that larger volume and thickness were associated with better executive function task performance. In the sample with Alzheimer’s disease, the average partial correlation between measures of executive function and fMRI was positive (pr=0.26, Ztest=3.94, p<0.001, 95% CI=0.05, 0.45), indicating that larger volume and thickness were also associated with better executive function task performance. Finally, in the HC sample, the average partial correlation between measures of executive function and fMRI was positive (pr=0.17, Ztest=3.10, p=0.001, 95% CI=−0.06, 0.38), indicating that larger volume and thickness were associated with better executive function task performance.
Magnitude of Effect Size in HC Versus Alzheimer’s Disease Samples
When separated by sample type, both HC and Alzheimer’s disease groups showed positive brain-behavior relationships. In the functional neuroimaging modality, the strength of the association between executive function and fMRI-rfMRI measures varied across samples, as indicated by partial correlations ranging from 0.18 to 0.44. Heterogeneity tests in functional neuroimaging indicated no significant heterogeneity (Q=7.08, p=0.718, I2=0.00%) between the Alzheimer’s disease (Q=2.07, p=0.839, I2=0.00%) and HC (Q=3.61, p=0.462, I2=0.00%) groups. For structural neuroimaging, the strength of the association between executive function and fMRI measures varied across samples, indicated by partial correlations ranging from 0.17 to 0.26. Heterogeneity tests revealed no significant heterogeneity (Q=1.44, p=0.998, I2=0.00%) between the Alzheimer’s disease (Q=0.80, p=0.977, I2=0.00%) and HC (Q=0.45, p=0.930, I2=0.00%) groups. Together, the results indicate no significant difference in the strength of the brain-behavior relationship between the Alzheimer’s disease and HC groups in both the functional and the structural neuroimaging modalities.
Structural Neuroimaging Versus Functional Neuroimaging Modalities
When separated by imaging modality, larger volume and thickness and greater blood-oxygen-level-dependent (BOLD) activations were associated with better executive function task performance. For Alzheimer’s disease, the strength of the association between executive function and brain imaging measures varied across samples, indicated by partial correlations ranging from 0.26 to 0.44. Heterogeneity tests in the Alzheimer’s disease group indicated no significant heterogeneity (Q=3.59, p=0.980, I2=0.00%) between functional neuroimaging (Q=2.07, p=0.839, I2=0.00%) and structural neuroimaging (Q=0.80, p=0.977, I2=0.00%). In the HC group, the strength of the association between executive function and brain imaging measures lacked significant variability across samples, indicated by partial correlations ranging from 0.17 to 0.18. Heterogeneity tests in the HC group indicated no significant heterogeneity (Q=4.06, p=0.851, I2=0.00%) between the functional neuroimaging (Q=3.61, p=0.462, I2=0.00%) and the structural neuroimaging (Q=0.45, p=0.930, I2=0.00%) groups. Across all studies, the strength of the association between executive function and brain imaging measures ranged from 0.21 to 0.32. Heterogeneity tests in the full sample indicated no significant heterogeneity (Q=9.09, p=0.982, I2=0.00%) between all functional neuroimaging studies (Q=7.08, p=0.718, I2=0.00%) and all structural neuroimaging studies (Q=1.44, p=0.998, I2=0.00%). Together, the results indicate no significant difference in the strength of the brain-behavior relationship between functional and structural neuroimaging in the full sample and when separated into Alzheimer’s disease and HC groups.
Exploratory Analyses
To ensure moderators did not affect the outcome of the analyses, exploratory moderator analyses were run for age and sex. Age as a moderator did not contribute significant variability between the Alzheimer’s disease and HC groups in the functional neuroimaging modality (β=−0.44, p=0.246) or in the structural neuroimaging modality (β=−0.39, p=0.638). Age also did not contribute a significant amount of variability between structural and functional neuroimaging modalities in the Alzheimer’s disease group (β=−0.43, p=0.417), in the HC group (β=0.05, p=0.912), or across the full sample (β=−0.33, p=0.319). Sex as a moderator also did not contribute significant variability between the Alzheimer’s disease and HC groups in functional neuroimaging (β=0.40, p=0.298) or structural neuroimaging (β=0.02, p=0.985). In addition, sex did not contribute a significant amount of variability between structural and functional neuroimaging modalities in the Alzheimer’s disease (β=0.45, p=0.399) or HC (β=0.24, p=0.628) groups or across the full sample (β=0.27, p=0.439).
Measurement issues such as brain region and executive function tests were also considered in exploratory analyses. When considering the region of interest included in analyses across both functional and structural neuroimaging modalities and across the Alzheimer’s disease and HC groups, there was no significant difference (Q=7.15, p=0.976, I2=0.00%) between studies that used solely the frontal lobe (pr=0.26, 95% CI=0.14, 0.36, Q=3.11, p=0.978, I2=0.00%) and studies that considered both the frontal and the parietal lobes (pr=0.26, 95% CI=−0.06–0.48, Q=4.40, p=0.623, I2=0.00%). When considering whether one executive function task or a composite of executive function tasks were used, no significant difference was found (Q=9.09, p=0.982, I2=0.00%) between studies that used a single test (pr=0.22, 95% CI=0.03, 0.36, Q=6.71, p=0.867, I2=0.00%) and studies that used a composite of executive function tests (pr=0.32, 95% CI=0.17, 0.44, Q=1.96, p=0.962, I2=0.00%). Together, exploratory analyses revealed that neither age and sex moderators nor region and executive function tasks contribute to the robust findings of this study.
Discussion
This study reveals concordance in the brain-behavior relationship between functional and structural imaging modalities at a meta-analytic level, such that larger cortical size and greater BOLD activation were both moderately associated with better performance on measures of executive function among patients with Alzheimer’s disease and HCs. However, when separated into subgroups comparing disease groups or imaging modality, results consistently revealed a lack of heterogeneity, indicating that the relationship between brain and behavior did not vary as a function of disease state or imaging modality. Exploratory analyses revealed that the lack of heterogeneity was also not due to age, sex, region imaged, or executive function task type. A key implication of this finding is that it is not Alzheimer’s disease pathology, per se, that gives rise to deficits in executive function, but the degree to which the CEN is compromised, a point relevant to the interpretation of neuropsychological data.
Together, the results reveal relatively and consistently robust outcomes, indicating that on a meta-analytic level, when assessing the relationship between brain and executive function behavioral performance, both structural and functional imaging are similarly predictive of performance in both HC and Alzheimer’s populations when performed on central hubs of the CEN. Findings of within-network connectivity of CEN among people with Alzheimer’s disease have been inconsistent, regardless of its relationship to executive function. Results of concordance suggest that patterns of atrophy in Alzheimer’s disease (i.e., medial-temporal atrophy vs. whole-brain atrophy) may help to explain the variability of these findings. In summary, investigations of executive function among people with Alzheimer’s disease may benefit from exploring the interactions between structural and functional measures of neural integrity as potential predictors of function.
An important factor to consider when assessing the brain-behavior relationship in Alzheimer’s disease is disease progression and severity of the disease. Cognitive deficits vary on the basis of clinical presentation of Alzheimer’s, which is heterogeneous (22), and brain atrophy is associated with executive function deficits in AD (23). Together with this evidence, the results of this meta-analysis highlight how Alzheimer’s disease pathology may not be the primary factor that gives rise to executive function deficits, but the degree to which the CEN is compromised. It is also important to note that, because Alzheimer’s disease severity was not standardized across studies in this analysis, it is possible that confounding variables (e.g., patients’ inability to perform tasks as a result of other limitations aside from atrophy) associated with varying levels of Alzheimer’s disease severity may have affected these results. With more research across varying levels of Alzheimer’s disease severity, future work will have the power to elucidate confounding variables. Future work in this area may be beneficial, particularly as clinical research focuses on identifying prodromal markers for Alzheimer’s disease to facilitate early intervention and may be able to highlight the strength of the brain-behavior relationship as a potential marker as well. In addition, because executive function is crucial for adaptive functioning, future research would benefit from assessing how functional measures of executive function, related to adaptive functioning, are associated with the structural and functional integrity of the CEN.
The association found between brain and behavior generally coincides with the findings of previous meta-analytic research in the field with HC populations, which show a positive relationship between brain volume in various regions (e.g., medial prefrontal cortex, orbitofrontal cortex, and lateral prefrontal cortex) and performance on executive function tasks (9). Norman and Shallice’s (12) meta-analysis of the supervisory attention control system (control over nonroutine responding) found that a right midcingulate-inferior frontal core network emerged, although whole brain analysis indicated that the dorsolateral prefrontal cortex was a noncore associate as well (24). More important, the present study expanded the approach of Yuan and Raz (9) by including functional neuroimaging. By taking a network approach, this work is consistent with current directions in the field, focusing on a systems-level understanding of behavior rather than the older region-of-interest one-to-one approach between brain and behavior.
Although findings of concordance are important in the context of the brain-behavior relationship, the lack of heterogeneity between the Alzheimer’s disease and HC groups is curious because of the nature of Alzheimer’s as a neurodegenerative disease. However, research shows that preclinical individuals report subjective cognitive decline before evidence of decline, as suggested by cognitive assessments (25), and decline in working memory and executive function is seen across normally aging people as well as those with mild cognitive impairment or Alzheimer’s disease (26). Together, this work indicates that aging HC individuals may also experience some level of cognitive decline, with the presumed similarities in sample score distributions contributing to a lack of heterogeneity between groups. As such, this lack of clarity and precision in assessing executive function is a considerable limitation in the field.
Considering the debate regarding the construct of measurement in executive function tasks, which is important for contextualizing the relationship between neural architecture and executive function, the field would benefit from a larger number of studies that treat executive function tasks as their own independent variables. For instance, assessing executive function as an independent variable may help clarify cortical morphometry and functional networks that are centrally important for executive function. In addition, assessing this relationship across various executive function tasks may help to narrow the knowledge gap in understanding the utility of executive function tasks in capturing executive function constructs. More important, expanding research in this way has the potential to provide important information for neuropsychiatric groups, such as those with Alzheimer’s disease, who may benefit from clear executive function assessment that provides accurate information about neurocognitive functioning. Following this logic, a future direction of this research involves ecological validity, not only of executive function measures but also of related measures of adaptive functioning.
Increasing the amount of work in this field will be useful in elucidating the brain-behavior relationship in executive function for both healthy and neuropsychiatric groups. When conducting a thorough review of the literature, various studies reported assessing for a brain-behavior relationship but neglected to provide statistics for those relationships, highlighting a potential file drawer effect, in which researchers are not encouraged to report findings that are not statistically significant in the field. To assess whether publication bias was present in this study, a graph representing the funnel plot was generated (Figure 3), which reveals that publication bias is not a major concern.
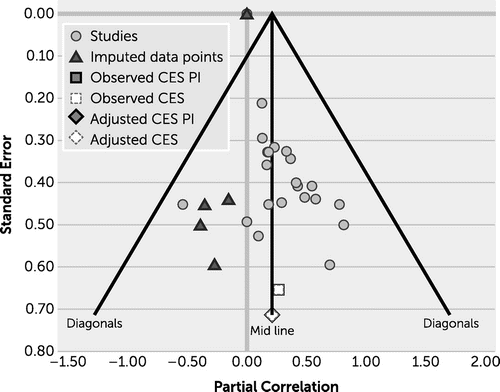
FIGURE 3. Publication bias funnel plota
a The publication analysis funnel plot includes the full sample with both structural and functional neuroimaging studies for the Alzheimer’s disease and healthy control groups. Observed pr=0.27 (SE=0.05), Q=11.58, I2=0.00%. CES=combined effect size; CES-PI=combined effect size-prediction interval.
In addition, although the present results provide evidence of positive associations among imaging modalities, behavior, and disease groups, they should be cautiously interpreted because of methodological limitations related to lack of published statistics on this topic. It is difficult to make conclusions about differences between the HC structural group, which only included studies reporting prefrontal cortex associations, and the Alzheimer’s structural group, which included studies reporting both prefrontal and parietal associations. Thus, the HC group may have more of a region-of-interest approach, and future work may expand on this by considering a detailed network approach. Moreover, studies included in this meta-analysis differed in the variables that were controlled for when examining the relations between brain structure-function and executive function. One implication of this is that our aggregated effect sizes based on the partial effects represent a conservative estimate of the association between brain structure-function and executive function.
In summary, the results of this meta-analysis indicate that the relationship between brain and behavior does not vary by disease state or imaging modality. More important, this work highlights that behavior is correlated to the degree to which the CEN is affected, regardless of how this network is affected. These findings help contextualize previous Alzheimer’s disease research and are significant in the context of methodological decisions made in multimodal neuroimaging research.
1 : Executive functions and the frontal lobes: a conceptual view. Psychol Res 2000; 63:289–298Crossref, Medline, Google Scholar
2 : The relationship between working memory capacity and executive functioning: evidence for a common executive attention construct. Neuropsychology 2010; 24:222–243Crossref, Medline, Google Scholar
3 : Age-related change in executive function: developmental trends and a latent variable analysis. Neuropsychologia 2006; 44:2017–2036Crossref, Medline, Google Scholar
4 : Executive functions and their disorders. Br Med Bull 2003; 65:49–59Crossref, Medline, Google Scholar
5 : The elusive nature of executive functions: a review of our current understanding. Neuropsychol Rev 2007; 17:213–233Crossref, Medline, Google Scholar
6 : Executive function and the frontal lobes: a meta-analytic review. Neuropsychol Rev 2006; 16:17–42Crossref, Medline, Google Scholar
7 : Functions of the frontal lobes: relation to executive functions. J Int Neuropsychol Soc 2011; 17:759–765Crossref, Medline, Google Scholar
8 : Is there a dysexecutive syndrome? Philos Trans R Soc Lond B Biol Sci 2007; 362:901–915Crossref, Medline, Google Scholar
9 : Prefrontal cortex and executive functions in healthy adults: a meta-analysis of structural neuroimaging studies. Neurosci Biobehav Rev 2014; 42:180–192Crossref, Medline, Google Scholar
10 : Recent developments in working memory. Curr Opin Neurobiol 1998; 8:234–238Crossref, Medline, Google Scholar
11 : Dementia and working memory. Q J Exp Psychol A 1986; 38:603–618Crossref, Medline, Google Scholar
12 : Attention to action; in Consciousness and Self-Regulation. Edited by . New York, Springer, 1986Crossref, Google Scholar
13 : Dissociable intrinsic connectivity networks for salience processing and executive control. J Neurosci 2007; 27:2349–2356Crossref, Medline, Google Scholar
14 : Fifty years of prefrontal cortex research: impact on assessment. J Int Neuropsychol Soc 2017; 23:755–767Crossref, Medline, Google Scholar
15 : Executive cognitive abilities and functional status among community-dwelling older persons in the San Luis Valley Health and Aging Study. J Am Geriatr Soc 1998; 46:590–596Crossref, Medline, Google Scholar
16 : Executive functioning as a potential mediator of age-related cognitive decline in normal adults. J Exp Psychol Gen 2003; 132:566–594Crossref, Medline, Google Scholar
17 : Executive function, more than global cognition, predicts functional decline and mortality in elderly women. J Gerontol A Biol Sci Med Sci 2007; 62:1134–1141Crossref, Medline, Google Scholar
18 : Executive function and instrumental activities of daily living in mild cognitive impairment and Alzheimer’s disease. Alzheimers Dement 2011; 7:300–308Crossref, Medline, Google Scholar
19 : Volumetric correlates of memory and executive function in normal elderly, mild cognitive impairment and Alzheimer’s disease. Neurosci Lett 2006; 406:60–65Crossref, Medline, Google Scholar
20 : Preferred reporting items for systematic reviews and meta-analyses: the PRISMA statement. PLoS Med 2009; 6:e1000097Crossref, Medline, Google Scholar
21 : Introduction, comparison, and validation of Meta-Essentials: a free and simple tool for meta-analysis. Res Synth Methods 2017; 8:537–553Crossref, Medline, Google Scholar
22 : Variability in cognitive presentation of Alzheimer’s disease. Cortex 2008; 44:185–195Crossref, Medline, Google Scholar
23 : Medial temporal lobe atrophy relates to executive dysfunction in Alzheimer’s disease. Int Psychogeriatr 2012; 24:1474–1482Crossref, Medline, Google Scholar
24 : Three key regions for supervisory attentional control: evidence from neuroimaging meta-analyses. Neurosci Biobehav Rev 2015; 48:22–34Crossref, Medline, Google Scholar
25 : Working memory and control of attention in persons with Alzheimer’s disease and mild cognitive impairment. Neuropsychology 2007; 21:458–469Crossref, Medline, Google Scholar
26 : Working memory and executive function decline across normal aging, mild cognitive impairment, and Alzheimer’s disease. BioMed Res Int 2015; 2015:748212Crossref, Medline, Google Scholar
27 : Correlational structure of “frontal” tests and intelligence tests indicates two components with asymmetrical neurostructural correlates in old age. Intelligence 2014; 46:94–106Crossref, Medline, Google Scholar
28 : Letter and category fluency performance correlates with distinct patterns of cortical thickness in older adults. Cereb Cortex 2019; 29:2694–2700Crossref, Medline, Google Scholar
29 : Structural brain correlates of delay of gratification in the elderly. Behav Neurosci 2014; 128:134–145Crossref, Medline, Google Scholar
30 : The association between aerobic fitness and executive function is mediated by prefrontal cortex volume. Brain Behav Immun 2012; 26:811–819Crossref, Medline, Google Scholar
31 : Age-related shift in neuro-action during a word-matching task. Front Aging Neurosci 2017; 9:265Crossref, Medline, Google Scholar
32 : Brain network connectivity mediates education-related cognitive performance in healthy elderly adults. Curr Alzheimer Res 2019; 16:19–28Crossref, Medline, Google Scholar
33 : Caudate dopamine D1 receptor density is associated with individual differences in frontoparietal connectivity during working memory. J Neurosci 2011; 31:14284–14290Crossref, Medline, Google Scholar
34 : Clinically silent Alzheimer’s and vascular pathologies influence brain networks supporting executive function in healthy older adults. Neurobiol Aging 2017; 58:102–111Crossref, Medline, Google Scholar
35 : The relationship between voxel-based metrics of resting state functional connectivity and cognitive performance in cognitively healthy elderly adults. Brain Imaging Behav 2018; 12:1742–1758Crossref, Medline, Google Scholar
36 : Antisaccade task reflects cortical involvement in mild cognitive impairment. Neurology 2013; 81:1235–1243Crossref, Medline, Google Scholar
37 : Associations of Bcl-2 rs956572 genotype groups in the structural covariance network in early-stage Alzheimer’s disease. Alzheimers Res Ther 2018; 10:17Crossref, Medline, Google Scholar
38 : The neural correlates of auditory and visuospatial span in logopenic progressive aphasia and Alzheimer’s disease. Cortex 2016; 83:39–50Crossref, Medline, Google Scholar
39 : The thickness of posterior cortical areas is related to executive dysfunction in Alzheimer’s disease. Clinics (São Paulo) 2014; 69:28–37Crossref, Google Scholar
40 : Distinct neuroanatomical substrates and cognitive mechanisms of figure copy performance in Alzheimer’s disease and behavioral variant frontotemporal dementia. Neuropsychologia 2011; 49:43–48Crossref, Medline, Google Scholar
41 : Contrasting prefrontal cortex contributions to episodic memory dysfunction in behavioural variant frontotemporal dementia and Alzheimer’s disease. PLoS One 2014; 9:e87778Crossref, Medline, Google Scholar
42 : APOE-MS4A genetic interactions are associated with executive dysfunction and network abnormality in clinically mild Alzheimer’s disease. Neuroimage Clin 2019; 21:101621Crossref, Medline, Google Scholar
43 : Cerebrovascular disease influences functional and structural network connectivity in patients with amnestic mild cognitive impairment and Alzheimer’s disease. Alzheimers Res Ther 2018; 10:82Crossref, Medline, Google Scholar
44 : Differentially disrupted functional connectivity of the subregions of the inferior parietal lobule in Alzheimer’s disease. Brain Struct Funct 2015; 220:745–762Crossref, Medline, Google Scholar
45 : Distinct patterns of interhemispheric connectivity in patients with early-and late-onset Alzheimer’s disease. Front Aging Neurosci 2018; 10:261Crossref, Medline, Google Scholar
46 : Regional functional connectivity predicts distinct cognitive impairments in Alzheimer’s disease spectrum. Neuroimage Clin 2014; 5:385–395Crossref, Medline, Google Scholar
47 : Resting state fMRI in Alzheimer’s disease: beyond the default mode network. Neurobiol Aging 2012; 33:1564–1578Crossref, Medline, Google Scholar