Neural and Endocrine Correlates of Sadness In Women: Implications for Neural Network Regulation of HPA Activity
Abstract
In order to evaluate the suprahypothalamic neuroanatomical sites of relevance to adrenocorticotropic hormone (ACTH) and cortisol regulation, single photon emission computed tomography (SPECT) investigation of induced sadness was combined with a linear regression analysis of these hormone levels during mood induction. Images from eight healthy women were analyzed by statistical parametric mapping (SPM), replicating many findings from prior sadness induction studies. Statistical parametric mapping endocrine covariate analysis showed that the ventromedial prefrontal, anterior cingulate, and insular cortices may regulate ACTH and the insular cortex may be related to regulation of cortisol during sadness. Dysfunction of these sites may contribute to the cortisol dysregulation observed in some subjects with major depression.
The hypothalamic-pituitary-adrenal (HPA) axis holds a prominent role in the history of biological psychiatry. Much of the research evaluating dysfunction of this neuroendocrine axis was conducted in major depressive disorder (MDD). The discovery of cortisol nonsuppression following dexamethasone challenge1 initiated several years of research that were further highlighted by the demonstration of elevated corticotropin releasing factor (CRF) in the cerebrospinal fluid of depressed subjects, thus confirming that dysfunction of the HPA axis in MDD was not of hypophysial origin.2
Subsequent research involving the HPA axis has evaluated neuroendocrine function in a myriad of conditions, including several psychiatric disorders and normal emotional states. In addition, investigation of the HPA axis has evolved to include efforts to delineate the suprahypothalamic neural network that regulates HPA function. In this regard, neuroanatomical studies of regulatory circuits have shown that the medial prefrontal cortex (PFC) may regulate adrenocorticotropic hormone (ACTH) and cortisol homeostasis.3,4 Many studies investigating these circuits, however, are lesion studies in animals, a limitation of which is the inability to evaluate neural systems in an intact state. This limitation can be surmounted by functional neuroimaging that allows for the investigation of connectivity among intact neural networks.5
Several behavioral states have been shown to reliably provoke the HPA axis; stress and anxiety have a long history in this regard.6 Our lab, and others, have shown that induced sadness can also increase peripheral cortisol levels.7,8 In this study, we used statistical parametric mapping (SPM) for omnibus analysis of single photon emission computed tomography (SPECT) images acquired during sadness in healthy women, and we further used the covariate function of SPM to undertake an exploratory analysis of the neural network specifically associated with the neuroendocrine response to sadness. The endocrine covariate analysis compared ACTH and cortisol levels collected at serial time points during mood induction with neuronal metabolic activity during the same time, thus introducing a neuroimaging methodology that has implications for evaluating regulation of the neuroendocrine axis as a dynamic system.
Neuroanatomical sites of a priori significance for the regulation of ACTH and cortisol during sadness include the amygdala,9 hippocampus,10 and the medial and subgenual PFC.3,11,12 Neuroanatomical sites of a priori significance for sadness (independent of neuroendocrine measures) would also be expected to affect these regions,13,14 but further recruit a distributed network of structures, including the dorsolateral PFC, orbitofrontal PFC, and insular and inferior parietal cortices.13–15 This study, therefore, has the primary objective of identifying the neuroanatomical regions of relevance to regulation of the HPA axis during sadness (i.e., the SPM endocrine covariate analysis) and a secondary objective of replicating both the neural correlates of sadness independent of neuroendocrine measures and the endocrine response to sadness independent of the assessment of brain activity.
METHODS
Subjects
Eight healthy, right-handed women aged 18–30 were recruited via advertisement. Women were recruited exclusively because of their tendency to show greater effects from the Velten mood induction procedure16 and because mood induction studies produce a gender specific neural activation profile.17,18 Exclusion criteria included any recent medical history (e.g., within the 6 months prior to the study) or any history of psychiatric illness, abuse of alcohol or illicit substances, or prescription of any medications within the 6 months prior to the study. Informed consent was obtained after all relevant procedures were fully explained. Subjects received $100 for each experimental session.
Procedures
The sadness and neutral mood inductions described in this manuscript were part of a larger study.
Subjects underwent sadness and neutral mood induction SPECT scans on separate days, each for 30 minutes. In order to control for the diurnal variation of cortisol secretion, each experimental session took place between noon and 5:00 p.m. Similarly, in order to account for a possible effect of gonadal hormones, the sadness and neutral scans were both acquired on approximately the same day of the luteal or follicular phase for each respective subject.
Time zero was defined as the first blood draw and followed subjects’ completion of the Beck Depression Inventory,19 Positive and Negative Affect Schedule,20 and the Spielberger Anxiety Scale.21 Mood induction started at time 150 minutes (following a 90-minute bland travel video) and finished at time 180 minutes. Radiotracer injection (Tc-99m) occurred in the midst of mood induction, at approximately time 167 minutes. SPECT scanning commenced at time 225 minutes and finished at time 255 minutes [cerebral binding of Tc-99m occurs within the few minutes following injection (time 167 minutes) and then has a 6-hour half-life].22,23
Mood Induction
The Velten mood induction procedure (VMIP), developed by Emmett Velten in 1968,24 has been shown to successfully produce sad mood as evaluated by physiological measures and self and observer reports.25 It entails visual presentation of 50 written sentences to be read silently, with instructions to simultaneously visualize a mood congruent scenario. Each sentence is reviewed in this manner for approximately 20 seconds, and subjects are further instructed to maintain the target mood for an additional 15 minutes after completion of all the sentences. Thus, the duration of the target mood was approximately 30 minutes. Each sadness and neutral induction occurred on separate days, and the order of exposure was randomized. Examples of sentences used in the VMIP that were intended to induce sadness are: “I have too many bad things in my life” and “Every now and then I feel so tired and gloomy that I’d rather just sit than do anything.” Examples of sentences that were intended to induce a neutral mood are: “Utah is a beehive state,” and “This book or any part thereof must not be reproduced in any form.”
Scales and Mood Self-Report
Mood, characterized through measures of anger, sadness, elation, and fear, was assessed through repeated administration of a visual analog scale (VAS) prior to, during, and following mood induction, producing a total of six VAS assessments (times 0, 15, 90, 135, 165, and 210 minutes). We assessed the degree of sadness achieved by the sadness induction relative to the neutral induction by comparing the difference in VAS scores before and during the sadness induction to the difference in VAS sadness scores before and during the neutral mood induction. Specifically, we established a mood baseline by averaging the two VAS scores sampling the 45 minutes prior to mood induction (times 90 and 135 minutes) and compared the mood baseline to the VAS score at the midst of mood induction (time 165 minutes). The degree of change in pre- and midst-VMIP sadness VAS scores was also compared to the degree of change in VAS scores for the other three indices of mood in order to assess whether the target mood was achieved exclusively. Statistical comparisons were performed by means of single tailed Wilcoxon Signed Ranks Test (WSRT). The use of nonparametric statistics was warranted by a sample size of eight subjects.26 Statistical assessment of change in VAS score was one-tailed because the experimental question was intended to address only whether the VMIP altered mood toward the target emotion (i.e., whether subjects were more sad after the VMIP, as compared to preinduction).
Image Acquisition
The CeraSPECT 3000 stationary annular camera, produced by Digital Scintigraphics Incorporated (DSI, Waltham Massachusetts), was used for image acquisition. This camera contains a sodium iodide crystal, and counts are acquired in 120 views, with three projections in each view. The scanner has a resolution of 5.3 mm for cortical neuroanatomy and a resolution of 7.7 mm for the deep nuclei.27,28 Scans were acquired at the Department of Nuclear Medicine, St. Elizabeth’s Hospital, Boston, Massachusetts.
Radiotracer (Tc-99m ECD) injection occurred in the midst of mood induction, 1 hour prior to scanning while subjects were seated in a quiet room with their eyes open amid background ambient lighting and with instructions to maintain either a sad or neutral mood for 15 minutes after completion of the VMIP. Tc-99m has a physical half-life of 6 hours,23 and brain retention of Tc-99m ECD at 1 hour postinjection is approximately 80% of the 5-minute postinjection concentration.22,29 Single photon emission computed tomography brain images collected 1.5 hours postinjection clearly demonstrate an intense radiotracer presence.22 During each scan, subjects rested quietly with the posterior and lateral aspects of their heads supported by the scanner head rest. Further support was afforded by use of a headholder applied across the anterior aspect of the head.
Image Processing
Imaging data, initially collected in DSI proprietary format, were converted to Interfile format and, subsequently, Analyze format. Following reconstruction, movement corrected whole brain normalized images were transformed to Montreal Neurological Institute (MNI) standard anatomical space.30 The mean concentration of each run was obtained as an area-weighted sum of the concentration of each slice and adjusted to a nominal value of 50 ml/minute per 100 g, yielding normalized blood flow images. The data were then rescaled and smoothed with a two-dimensional Gaussian filter of 15 mm in width (full width at half maximum).
Image Analysis
Omnibus analysis of SPECT data was conducted following the theory of SPM31 through use of the SPM99 software package (Wellcome Department of Cognitive Neurology, London UK). Three separate primary analyses were conducted. The first was a contrast of the sadness condition versus the neutral condition independent of neuroendocrine measures. For this contrast, the analysis of variance conducted within the group considered scan condition as the main effect (sadness versus neutral) and subjects as a block effect. The two other primary analyses included one for the sadness condition with cortisol as the covariate of interest and one for the sadness condition with ACTH as the covariate of interest. Two corresponding endocrine control analyses were conducted for the neutral mood induction.
Statistical parametric mapping endocrine covariate analyses assessed the neural correlates of ACTH and cortisol levels that were averaged from values determined from blood draws collected at times 165 and 180 minutes, time points bracketing the injection of radiotracer. Both of these blood draws occurred during the time of the sad mood state. For these maps, the covariates option was selected, generating separate regression analyses that tested the linear relationship between rCBF associated with either the sadness condition or the neutral condition and ACTH or cortisol collected during sadness and neutral inductions across subjects, yielding a Z score at each voxel. For ease of discussion, we refer to the findings in terms of significant “correlations,” although the analysis formally involved linear regression rather than assessment of correlation. While complete scanning data were collected for all eight subjects, and the analysis of the sadness condition independent of neuroendocrine measures included all eight subjects, blood samples were incomplete for two subjects. Thus, the SPM endocrine covariate analyses were performed only for this subset of six subjects.
For all three primary image analyses, regions containing foci of activation with Z scores >3.09 are reported. A Z score of >3.09 corresponds to a p<0.001 (one-tailed), uncorrected for multiple comparisons.32 Since, to our knowledge, this is the first study of its kind, we chose to employ relatively liberal statistical thresholds in an effort to generate more refined hypotheses for future studies. More stringent thresholds together with more circumscribed hypotheses are recommended for future experiments.
Endocrine Measures and Analysis
Blood draws for cortisol and ACTH (10 ml each) occurred at times 0, 90, 150, 165, 180, 195, and 210 minutes. Blood samples were placed on ice after blood draw and aliquoted and frozen after the experimental session. Serum cortisol was assayed by a commercially available solid phase double antibody iodine-125 radioimmunoassay (125I RIA), which has a sensitivity of 1.2 μg/dl. Plasma ACTH was assayed through use of equilibrium 125I RIA, which has a sensitivity of 20 pg/ml (Diagnostic Products Corporation, Los Angeles).
The degree of change in cortisol levels resulting from mood induction was calculated by comparing the baseline cortisol level (the average of times 90 and 150 minutes, i.e., pre-VMIP) with the average of the four cortisol levels following the VMIP (times 165, 180, 195, and 210 minutes). Two subjects did not have adequate blood draws at various time points. Thus, assessment of the endocrine response to sadness (independent of brain activity) was performed in this subset of six subjects. The same procedure was used to assess pre- and post-VMIP ACTH levels. Comparisons were made by means of WSRT, with use of nonparametric statistics warranted by a sample size of six subjects.26 Two-tailed assessments were conducted in order to evaluate for either an increase or decrease in hormone levels.
RESULTS
Mood Data
Comparison of change in group mean sadness VAS scores before and at the midst of sadness and neutral inductions demonstrated a significant increase in sadness scores for the sadness VMIP (mean=17, SD=26.9) relative to the neutral VMIP (mean=0.0, SD=8.6) [one-tailed WSRT; Z=−1.859, df=7, p=0.032]. In contrast, assessment of VAS scores for anger (mean=3.0, SD=7.4), elation (mean=20.6, SD=12.6), and fear (mean=6.0, SD=16.6), before and at the midst of sadness induction did not demonstrate any significant change in mood, which confirms that our subjects achieved a sad state not significantly contaminated by anger, elation, or fear (one-tailed WSRT, anger: Z=−0.420, df=7, p=−0.335, elation: Z=−0.105, df=7, p=0.46, fear: Z=−1.483, df=7, p=0.069).
Imaging Data
As demonstrated by omnibus (i.e., SPM) evaluation, sadness induction produced several regions of altered rCBF relative to neutral mood induction (Table 1). Areas showing increased rCBF include the left orbitofrontal cortex (OFC), Broca’s area, the right anterior cingulate cortex (ACC), and the right mid-insular region. Areas showing decreased rCBF during sadness include the anterior cerebellum and the middle and superior temporal gyri in the left hemisphere, and the medial occipital gyrus, lingual gyrus, and anterolateral cerebellum in the right hemisphere.
SPM assessments of the within-group analysis of the sadness condition with ACTH as the covariate of interest showed a significant positive correlation between ACTH and rCBF in the left anterior cingulate and right insular cortices (Table 1, Figure 1, and Figure 2) and a significant negative correlation between ACTH and rCBF at the junction of the right caudal OFC and ventromedial PFC (Table 1 and Figure 3). Within group analysis of the sadness condition with cortisol as the covariate of interest showed a significant positive correlation between cortisol and rCBF in the left insula (Table 1 and Figure 4). Importantly, the control covariate analyses of neuroendocrine data and the neutral condition did not reveal any significant correlations in any of these regions.
Endocrine Data
Comparison of ACTH levels before and after sadness VMIP showed a significant increase (two-tailed WSRT; Z=−2.21, p=0.027) in ACTH levels in response to sadness induction (mean δ=7.1 μg/dl, SD=5.1) relative to neutral induction (mean δ=3.0 μg/dl, SD=6.25). Comparison of cortisol levels before and after sadness VMIP showed a nonsignificant change (two-tailed WSRT; Z=−0.105, df=5, p=0.917) in cortisol levels in response to sadness induction (mean δ=1.26 μg/dl, SD=2.3) relative to the neutral induction (mean δ=1.43 μg/dl, SD=4.2).
DISCUSSION
The investigation of mood from the perspective of a paradigm that more directly integrates psychoendocrinology and functional neuroimaging holds significance for understanding the neurobiology of the basic emotions and for furthering an understanding of the pathophysiology of some psychiatric illnesses (e.g., mood disorders). Our study concurrently investigated the endocrine and neural correlates of induced sadness by means of collecting endocrine measures at time points bracketing the temporal window of radiotracer injection, thus allowing for an integrated evaluation of these two neurobiological domains (ones that typically have been investigated in a manner whereby endocrine measures are not closely temporally correlated with cerebral activity).
Functional neuroimaging studies of induced sadness are beginning to demonstrate some degree of consensus. Our findings of greatest a priori interest (for sadness versus neutral induction, without investigation of the neuroendocrine measures) include increased rCBF in the left OFC, right ACC, and right insula—sites that have been identified, in part, by other investigators.13,15 To our knowledge, post hoc findings observed in this study, which include increased rCBF of Broca’s area, the left middle and superior temporal gyrus, and decreased rCBF of the left anterior temporal pole and occipital gyrus, and anterior cerebellum bilaterally, have not been observed as reliably by other investigators. Although not identified in our study, rCBF changes in the dorsolateral PFC, medial PFC, and medial temporal cortices have been observed in several different studies.13,15,33,34 Thus, integration of our results with findings from other studies suggests that a highly distributed network of cortical and subcortical structures mediates the experience of sadness.
The neural correlates of sadness, however, are merely one aspect of the complicated neurobiology of this emotion. Unification of neuroimaging and endocrine paradigms allows for in vivo correlation of hormone levels with neural activity, specifically in networks that are completely intact. Much of the research that combines neural and endocrine techniques is currently conducted on animals, where a discrete lesion is placed in the neuroanatomical region of interest, with subsequent assessment of change in cortisol levels. A possible shortcoming of lesion paradigms is their inability to assess the remote network effects of the “discrete” lesion. A lesion in a discrete portion of the PFC may have significant reverberations throughout the interacting cortical and subcortical network, of which the lesioned region is merely one component. For example, it has been demonstrated that lesions of the medial PFC and ACC alter cortisol responsiveness in rats exposed to stress.3,4 It is well established that cortisol secretion from the adrenal gland is partly dependent upon ACTH release from the anterior pituitary, which is itself dependent upon CRF release, of which the paraventricular nucleus (PVN) of the hypothalamus is a major site of production.10,35 Activity of the PVN is thought to be regulated by several neuroanatomical regions: the amygdala and bed nucleus of the stria terminalis are stimulatory, while the hippocampus is inhibitory.10 Although the medial PFC and ACC also appear to regulate the PVN, it is unclear to what degree these regions do this directly or through interaction with other neuroanatomical structures. For example, it is possible that a lesion of the medial PFC may (inadvertently) partly disinhibit the amygdala.36 Since the amygdala is known to stimulate the PVN, amygdalar disinhibition might actually affect the PVN more than a lesion intended to target the projections from the medial PFC to the hypothalamus.37 Furthermore, because the amygdala is also a site of CRF production,38 an investigation evaluating the regions relevant to regulation of the HPA axis would ideally also evaluate activity of the amygdala. Thus, in order to assess intact neural networks, neuroendocrine lesion studies may be well complemented by functional neuroimaging paradigms.
In support of the aforementioned animal studies, SPM endocrine covariate analysis in this study showed that ACTH measures were positively correlated with rCBF in the left anterior cingulate cortex and negatively correlated with rCBF in the right caudal OFC/ventromedial PFC, thus providing further evidence that the orbitomedial PFC and ACC are important regions involved in regulation of the HPA axis. In addition, ACTH measures were positively correlated with rCBF in the right insula. The complementary covariate analysis, which used peripheral cortisol levels as the covariate of interest, showed a positive correlation with rCBF in the left insula, a region that has projections to the amygdala and ACC and may, therefore, indirectly affect regulation of the HPA axis.39
Hence, our SPM covariate analysis further delineates components of the neural network regulating the HPA axis. These findings complement studies that have used sadness induction as an heuristic for investigation of the functional neuroanatomy of MDD.14 Mayberg et al. evaluated the neuroanatomical relationship between sadness and depression through comparison of the neuroanatomical regions recruited during sadness in healthy volunteers to the neuroanatomical regions that showed metabolic change in patients recently recovered from a depressive episode and showed significant overlap between these two conditions.13 As a logical extension of this heuristic since we have shown that the ventromedial prefrontal, anterior cingulate, and insular cortices may be related to regulation of the HPA axis during sadness, aberrations of these structures in depressed subjects may account for some of the suprahypothalamic dysregulation of the HPA axis observed in some subjects with MDD (in addition to the putative involvement of the amygdala and hippocampus).40
In this regard, the caudal OFC, ventromedial PFC, and anterior cingulate cortex are sites that have been repeatedly implicated in the pathophysiology of MDD. For example, a subregion of the ventromedial PFC, the subgenual PFC, has been shown to have a smaller volume in subjects with MDD when compared to healthy volunteers.41 Furthermore, after correcting for reduced volume, the subgenual PFC shows increased rCBF in the active phase of MDD.42–44 Because the caudal OFC and ventromedial PFC have direct projections to the amygdala and structures that regulate the PVN,45,46 both of which are major sites of CRF production10,38,47 metabolic aberrations of the caudal OFC/ventromedial PFC may indirectly contribute to the elevation in CRF2 and the cortisol dysregulation1 found in many depressed subjects.
Different methodological dimensions of our study also merit discussion. One limiting feature was our use of SPECT as opposed to positron emission tomography (PET). Position emission tomography offers greater resolution of deep structures such as the hippocampus and amygdala, which are structures of relevance to emotion and regulation of the HPA axis.9,10 Single photon emission comuted tomography may not provide sufficient resolution to adequately evaluate these structures. Secondly, an issue of relevance to the endocrine dimension of our paradigm was the absence of a significant change in cortisol levels in response to sadness induction. The absence of this finding may not, however, have much practical significance. Our ACTH levels did increase, and it has been shown that not all ACTH pulses necessarily result in increases in cortisol levels.35 Furthermore, this issue did not preclude our main goal, which was identification of the neuroanatomical regions involved in the regulation of cortisol and ACTH during sadness. The presence of adequate variance in hormone levels across subjects (in response to sadness induction) allowed for the use of an SPM covariate analysis to identify the regions that uniquely correlated with this variance. Thirdly, the time course of sadness induced brain activity, and subsequent PVN, pituitary, and adrenal activity is difficult to evaluate. However, the typical delay of approximately 5–10 minutes between peripheral increases in ACTH and consequent adrenal release of cortisol48 suggests that the timing of our collection of peripheral neuroendocrine measures adequately brackets the temporal window of radiotracer capture by the brain.
Our paradigm correlates central neural activity and peripheral endocrine activity through the collection of endocrine measures at time points closely bracketing the temporal window of radiotracer injection and thereby differs from psychoendocrine paradigms that assess cortisol levels at a time point(s) that may not correspond to the cerebral activity responsible for genesis of the plasma level. Thus our methodology is oriented toward a more direct functional evaluation of the HPA axis. As an extension of this paradigm, the dynamic nature of the HPA axis may be more fully evaluated by pairing serial PET scans (or possibly functional MRI) with more frequent collection of neuroendocrine measures (e.g., possibly every minute). Furthermore, follow-up studies must evaluate more patients: the sample size of eight subjects in this study (with only six subjects with adequate data for endocrine covariate analysis), was reasonable to merely investigate a new methodology. The small sample size of our study limits the generalizability of our findings and requires replication with a greater sample size. However, our demonstration of an exclusive correlation of neuroendocrine measures with the ventromedial prefrontal and anterior cingulate cortices, regions with strong theoretical backing3,49 suggests that this methodology may be a robust and reasonably specific means by which to investigate the suprahypothalamic neural network that regulates the HPA axis.
In conclusion, our study has investigated both neural and endocrine dimensions of sadness in healthy, young adult women. Sadness (independent of endocrine activity) was associated with increased rCBF of the left OFC, right ACC, and right insula, findings that have also been observed in other studies.15,33 Independent of our investigation of neural activity, we also evaluated the endocrine response to the mental state of sadness, which showed increase of ACTH levels in the sadness induction relative to the neutral induction but no statistically significant change in cortisol levels. Our primary goal, which was identification of the neuroanatomical regions that may be involved in the regulation of ACTH and cortisol during sadness, identified the orbitomedial prefrontal, anterior cingulate, and insular cortices as possible regions in the regulation of ACTH and the insula as a possible regulatory site for cortisol. These sites have been shown to be affected in MDD and may account for the increase in CRF and cortisol found in MDD. Finally, our methodology was intended to acknowledge the dynamic nature of the HPA axis and apply an omnibus evaluation to the investigation of intact networks regulating this axis.
ACKNOWLEDGMENTS
We thank R. Connors, V. Gayshan, and S. Genna of Innovative Medical Imaging, Waltham, MA; R. Zimmerman of the Department of Nuclear Medicine at Brigham and Women’s Hospital, Boston ; and S. Weise and A. Bonab of the Department of Nuclear Medicine at Massachusetts General Hospital, Boston, for their technical assistance.
Innovative Medical Imaging donated use of their SPECT camera and Nycomed-Amersham donated the radiotracer for this study. Additional support was provided by the Clinical Research Training Program, Department of Psychiatry, Harvard Medical School (W.E.O.), and NIMH grant K23 MH-01735 (D.D.D.).
Portions of this artice were presented at the 13th Annual American Neuropsychiatric Association meeting, March 9–12, 2002, La Jolla, California and the 12th Annual Rotman conference on Emotions and the Brain, March 25–26, 2002, Toronto, Ontario, Canada.
![]() |
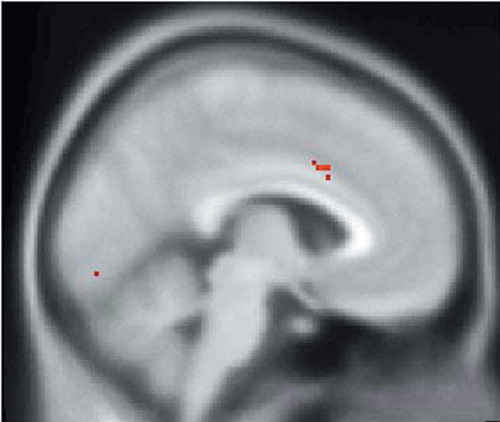
FIGURE 1. SPM99 Covariates Only Analysis of rCBF During the Sadness Condition With ACTH as the Covariate of Interest. The SPM of SPECT Data Corresponding to This Contrast is Superimposed Over a Nominally Normal MRI in Standardized Anatomical Space (Montreal Neurological Institute) for Gross Anatomical Reference. This Image is a Sagittal Section to the Left of and Parallel to the Midsagittal Plane. Voxels Exceeding the Z-score Threshold of 3.09 are Coded in Red and Signify a Positive Correlation of ACTH Levels With rCBF of the Left Anterior Cingulate Cortex
SPM=statistical parametric mapping; rCBF=regional cerebral blood flow; MRI=magnetic resonance imaging
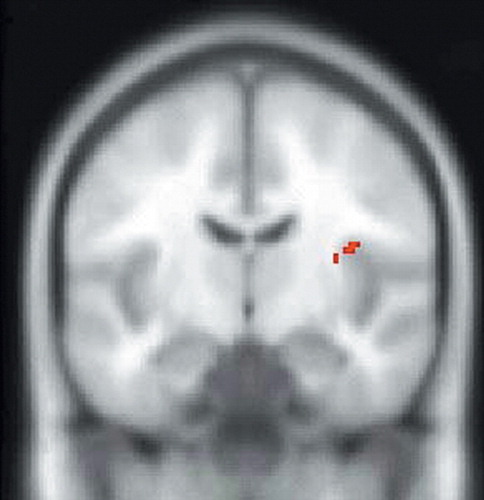
FIGURE 2. SPM99 Covariates Only Analysis of rCBF During the Sadness Condition With ACTH as the Covariate of Interest. The SPM of SPECT Data Corresponding to This Contrast is Superimposed Over a Nominally Normal MRI in Standardized Anatomical Space (Montreal Neurological Institute) for Gross Anatomical Reference. This Image is a Coronal Section. Voxels Exceeding the Z-score Threshold of 3.09 are Coded in Red and Signify a Positive Correlation of ACTH Levels With rCBF of the Right Insula
SPM=statistical parametric mapping; rCBF=regional cerebral blood flow; MRI=magnetic resonance imaging
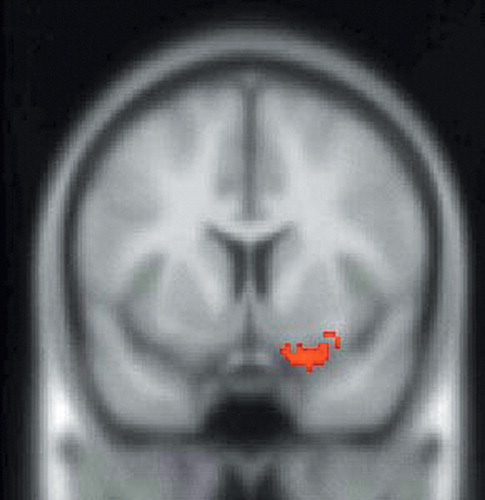
FIGURE 3. SPM99 Covariates Only Analysis of rCBF During the Sadness Condition With ACTH as the Covariate of Interest. The Statistical Parametric Map of SPECT Data Corresponding to This Contrast is Superimposed Over a Nominally Normal MRI in Standardized Anatomical Space (Montreal Neurological Institute) for Gross Anatomical Reference. This Image is a Coronal section. Voxels Exceeding the Z-score Threshold of 3.09 are Coded in Red and Signify a Negative Correlation of ACTH Levels With rCBF at the Intersection of the Right Caudal Orbitofrontal and Ventromedial Prefrontal Cortices
SPM=statistical parametric mapping; rCBF=regional cerebral blood flow; MRI=magnetic resonance imaging
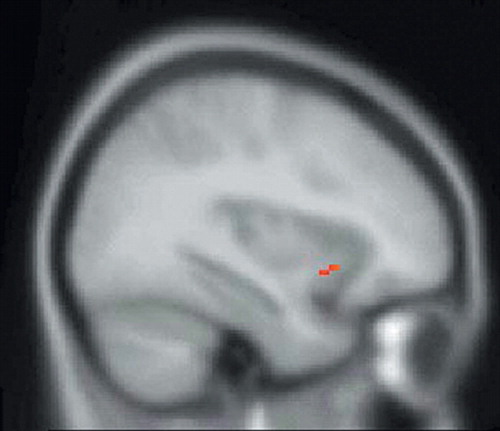
FIGURE 4. SPM99 Covariates Only Analysis of rCBF During the Sadness Condition With Cortisol As The Covariate of Interest. The SPM of SPECT Data Corresponding to This Contrast is Superimposed Over a Nominally Normal MRI in Standardized Anatomical Space (Montreal Neurological Institute) for Gross Anatomical Reference. This Image is a Sagittal Section. Voxels Exceeding the Z-score Threshold of 3.09 are Coded in Red and Signify a Positive Correlation of Cortisol Levels With rCBF of the Left Insula
SPM=statistical parametric mapping; rCBF=regional cerebral blood flow; MRI=magnetic resonance imaging
1 Carroll BJ, Martin FIR, Davies B: Resistance to suppression by dexamethasone of plasma 11-OHCS levels in severe depressive illness. Br Med J 1968; 3:285–287Crossref, Medline, Google Scholar
2 Nemeroff CB, Widerlov E, Bisette G, et al: Elevated concentrations of CSF corticotropin-releasing factor-like immunoreactivity in depressed patients. Science 1984; 226:1342–1344Crossref, Medline, Google Scholar
3 Sullivan RM, Gratton A: Lateralized effects of medial prefrontal cortex lesions on neuroendocrine and autonomic stress response in rats. J Neurosci 1999; 19:2834–2840Crossref, Medline, Google Scholar
4 Diorio D, Viau V, Meaney MJ: The role of the medial prefrontal cortex (cingulate gyrus) in the regulation of hypothalamic-pituitary-adrenal responses to stress. J Neurosci 1993; 13:3839–3847Crossref, Medline, Google Scholar
5 Friston KJ: Functional and effective connectivity in neuroimaging: a synthesis. Hum Brain Mapp 1994; 2:56–78Crossref, Google Scholar
6 Mason JW: A review of psychoendocrine research on the pituitary-adrenal cortical system. Psychosom Med 1968; 30:576–607Crossref, Medline, Google Scholar
7 Brown WA, Sirota AD, Niaura R, et al: Endocrine correlates of sadness and elation. Psychosom Med 1993; 55:458–467Crossref, Medline, Google Scholar
8 Buchanan TW, Al’Absi M, Lovaloo WR: Cortisol fluctuates with increases and decreases in negative affect. Psychoneuroendocrinology 1999; 24:227–241Crossref, Medline, Google Scholar
9 Drevets WC, Price JL, Bardgett ME, et al: Glucose metabolism in the amygdala in depression: relationship to diagnostic subtype and plasma cortisol levels. Pharmacol Biochem Behav 2002; 71:431–447Crossref, Medline, Google Scholar
10 Herman JP, Cullinan WE: Neurocircuitry of stress: central control of the hypothalamic-pituitary-adrenocortical axis. Trends Neurosci 1997; 20:78–84Crossref, Medline, Google Scholar
11 Morgan MA, LeDoux JE: Differential contribution of dorsal and ventral medial prefrontal cortex to the acquisition and extinction of conditioned fear in rats. Behav Neurosci 1995; 109:681–188Crossref, Medline, Google Scholar
12 Holsboer F: Neuroendocrinology of mood disorders, in Psychopharmacology: The Fourth Generation of Progress. Edited by Bloom FE, Kupfer DJ. New York, Raven Press, 1995, pp 957–969Google Scholar
13 Mayberg HS, Liotti M, Brannan SK, et al: Reciprocal limbic cortical function and negative mood: converging PET findings in depression and normal sadness. Am J Psychiatry 1999; 156:675–682Medline, Google Scholar
14 Beauregard M, Leroux JM, Bergman S, et al: The functional neuroanatomy of major depression: an fMRI study using an emotional paradigm. Neuroreport 1998; 9:3253–3258Crossref, Medline, Google Scholar
15 Damasio AR, Grabowski TJ, Bechara A, et al: Subcortical and cortical brain activity during the feeling of self-generated emotions. Nat Neurosci 2000; 3:1049–1056Crossref, Medline, Google Scholar
16 Sirota AD, Schwartz G: Facial muscle patterning and lateralization during elation and depression imagery. J Abnormal Psychol 1982; 91:25–34Crossref, Medline, Google Scholar
17 George MS, Ketter TA, Parekh PI, et al: Gender differences in regional cerebral blood flow during transient self-induced sadness or happiness. Biol Psychiatry 1996; 40:859–871Crossref, Medline, Google Scholar
18 Schneider F, Habel U, Kessler C, et al: Gender differences in regional cerebral activity during sadness. Hum Brain Mapp 2000; 9:226–238Crossref, Medline, Google Scholar
19 Beck AT, Ward CH, Mendelson M, Mock J, Erbaugh J: An inventory for measuring depression. Arch Gen Psychiatry 1961; 4:561–571Crossref, Medline, Google Scholar
20 Watson D, Clark LA, Tellegen A: Development and validation of brief measures of positive and negative affect: the PANAS scales. J Pers Soc Psychol 1988; 54:1063–1070Crossref, Medline, Google Scholar
21 Spielberger CD, Gorsuch RL, Lushene RD: STAI Manual. Palo Alto, Calif, Consulting Psychologists Press, 1970Google Scholar
22 Vallabhajosula S, Zimmerman RE, Picard M, et al: Technetium-99m ECD: a new brain imaging agent: in vivo kinetics and biodistribution studies in normal human subjects. J Nucl Med 1989; 30:599–604Medline, Google Scholar
23 Mettler FA, Guiberteau MJ: Radioactivity and radionuclides, in Essentials of Nuclear Medical Imaging, 3rd ed. Edited by Mettler FA, Guiberteau MJ. Philadelphia, WB Saunders, 1991, pp 1–14Google Scholar
24 Velten E: A laboratory task for induction of mood states. Behav Res Ther 1968; 6:473–482Crossref, Medline, Google Scholar
25 Larsen RJ, Sinnett LM: Meta-analysis of experimental manipulations: some factors affecting the Velten mood induction procedure. Pers Soc Psychol Bull 1991; 17:323–334Crossref, Google Scholar
26 Glass GV, Peckham PD, Sanders JR: Consequences of failure to meet assumptions underlying the fixed effects analysis of variance and covariance. Review of Educational Res 1972; 42:237–288Crossref, Google Scholar
27 Smith AP, Genna S: The development of ASPECT, an annular single crystal brain camera for high efficiency SPECT. IEEE Trans Nucl Sci 1988; 35:654–658Crossref, Google Scholar
28 Smith AP, Genna S: Acquisition and calibration principles for ASPECT—a SPECT camera using digital position analysis. IEEE Trans Nucl Sci 1998; 35:740–743Crossref, Google Scholar
29 Walovitch RC, Franceschi M, Picard M, et al: Metabolism of 99mTc-L,L-ethyl cysteinate dimer in healthy volunteers. Neuropharmacology 1991; 30:283–292Crossref, Medline, Google Scholar
30 Alpert NM, Berdichevsky D, Levin Z, et al: Improved methods for image registration. Neuroimage 1996; 3:10–18Crossref, Medline, Google Scholar
31 Friston KJ, Holmes AP, Worsley KJ, et al: Statistical parametric maps: a general linear approach. Hum Brain Mapp 1995; 2:189–210Crossref, Google Scholar
32 Worsley KJ, Marrett P, Neelin P, et al: A unified statistical approach for determining significant voxels in images of cerebral activation. Hum Brain Mapp 1996; 4:58–73Crossref, Medline, Google Scholar
33 Gemar MC, Kapur S, Segal ZV, et al: Effects of self-generated sad mood on regional cerebral activity: a PET study in normal subjects. Depression 1996; 4:81–88Crossref, Medline, Google Scholar
34 George MS, Ketter TA, Parekh PI, et al: Brain activity during transient sadness and happiness in healthy women. Am J Psychiatry 1995; 152:341–351Crossref, Medline, Google Scholar
35 Krishnan KRR, Ritchie JC, Saunders W, et al: Nocturnal and early morning secretion of ACTH and cortisol in humans. Biol Psychiatry 1990; 28:47–57Crossref, Medline, Google Scholar
36 O’Reardon JP, Brotman MA, DeRubeis RJ, et al: Prefrontal-amygdala interaction and mood regulation: a perfusion fMRI study. Brain Cogn, Rotman Research Institute Abstracts, 2003; 51:184–186Google Scholar
37 Ongur D, An X, Price JL: Prefrontal cortical projections to the hypothalamus in macaque monkeys. J Comp Neurol 1998; 401:480–505Crossref, Medline, Google Scholar
38 Makino S, Shibasaki T, Yamauchi N, et al: Psychological stress increased corticotropin-releasing hormone mRNA and content in the central nucleus of the amygdala but not in the hypothalamic paraventricular nucleus in the rat. Brain Res 1999; 850:136–143Crossref, Medline, Google Scholar
39 Augustine JR: Circuitry and functional aspects of the insular lobe in primates including humans. Brain Res Rev 1996; 22:229–244Crossref, Medline, Google Scholar
40 Gold PW, Drevets WC, Charney DS: New insights into the role of cortisol and the glucocorticoid receptor in severe depression. Biol Psychiatry 2002; 52:381–385Crossref, Medline, Google Scholar
41 Ongur D, Drevets WC, Price JL: Glial reduction in the subgenual prefrontal cortex in mood disorders. Proc Natl Acad Sci USA 1998; 95:13290–13295Crossref, Medline, Google Scholar
42 Drevets WC, Ongur D, Price JL: Reduced glucose metabolism in the subgenual prefrontal cortex in unipolar depression. Mol Psychiatry 1998; 3:190–191Crossref, Medline, Google Scholar
43 Drevets WC, Price JL, Simpson JR, et al: Subgenual prefrontal cortex abnormalities in mood disorders. Nature 1997; 386:824–827Crossref, Medline, Google Scholar
44 Drevets WC: Neuroimaging studies of mood disorders. Biol Psychiatry 2000; 48:813–829Crossref, Medline, Google Scholar
45 Freedman LJ, Insel TR, Smith Y: Subcortical projections of area 25 (subgenual cortex) of the macaque monkey. J Comp Neurol 2000; 421:172–188Crossref, Medline, Google Scholar
46 Zald DH, Kim SW: Anatomy and function of the orbital frontal cortex, I: anatomy, neurocircuitry, and obsessive-compulsive disorder. J Neuropsychiatry Clin Neurosci 1996; 8:125–138Link, Google Scholar
47 Galard R, Catalan R, Castellanos JM, et al: Plasma corticotropin-releasing factor in depressed patients before and after the dexamethasone suppression test. Biol Psychiatry 2002; 51:463–468Crossref, Medline, Google Scholar
48 Gallagher TF, Yoshida K, Roffwarg HD, et al: ACTH and cortisol secretory patterns in man. J Clin Endocrinol Metab 1973; 36:1058–1068Crossref, Medline, Google Scholar
49 Gold PW, Chrousos GP: The endocrinology of melancholic and atypical depression: relation to neurocircuitry and somatic consequences. Proc Assoc Am Physicians 1999; 111:22–34Crossref, Medline, Google Scholar