Repetitive Transcranial Magnetic Stimulation and Threat Memory: Selective Reduction of Combat Threat Memory P300 Response After Right Frontal-Lobe Stimulation
Abstract
Using the event-related potential P3a component as a marker, the authors tested the efficacy of repetitive transcranial magnetic stimulation (rTMS) for reducing hyperarousability to specific threat stimuli in one Vietnam veteran with chronic posttraumatic stress disorder (PTSD), who exhibited an exaggerated P3a response to combat-related pictures. Twenty minutes of 1-Hz rTMS to the right prefrontal area effected a reduction in the P3a amplitude, whereas similar rTMS to the left prefrontal area did not. In addition to providing evidence for the effectiveness of right frontal rTMS for an exaggerated response to trauma-related stimuli, this study provides electrophysiological corroboration of subjective reports of PTSD symptoms.
Patients with posttraumatic stress disorder (PTSD) display an atypical response to trauma-related stimuli.1 PTSD symptoms such as physiological reactivity to trauma reminders, efforts to avoid those reminders, hypervigilance, and exaggerated startle response (DSM–IV) evince this disorder. Hyperarousal to inappropriate appraisals of threat2 is one of the hallmark, and perhaps most debilitating, aspects of PTSD.
It is possible to obtain objective, physiological responses to visually-presented threatening stimuli at the electrophysiological level by examining event-related potentials (ERPs). The “oddball task” is the traditional paradigm for eliciting the P300 component.3 The standard P300 task includes a class of target stimuli, which appears about 20% of the time and elicits the P300 response, and the standard stimuli, which appear 80% of the time. In a three-condition oddball task, the target still appears 20% of the time and requires that the subject give a response each time it occurs, thus requiring attention to these stimuli. The standard stimuli in this task are now displayed 60% of the time and require no response. The non-target probe is a novel stimulus that appears the remaining 20% of the time, does not require subjects' response, and is used to measure a subject's involuntary attention. In this task, the ERP related to the target is called a P300b, or P3b. The non-target probe also evokes a positive wave deflection with a peak latency earlier than that of P3b, which is maximal over frontal-area electrodes, and is called a P300a, or P3a. P3b has been proposed to reflect the intentional allocation of attentional resources to a target that a subject responds to, whereas P3a indexes the capture of attention to the novel, non-target probe.4
An increased P300 response to novel or non-target stimuli has been established as a marker of a hyperaroused response or biased attention toward specific stimuli. For example, Miltner et al.5 showed that, relative to ERPs of control subjects, spider-phobic subjects showed increased P300 amplitudes to color pictures of spiders, whereas snake-phobic individuals showed increased P300 amplitude to snake pictures. In a metaanalysis of ERP studies of PTSD, Karl et al.1 concluded that P3a amplitudes to trauma-related distracter pictures were significantly higher in PTSD trauma-exposed groups than in non-PTSD trauma-exposed groups. Attias et al.,6 for example, conducted an ERP study of the hyperarousal response in PTSD, using a three-condition oddball task in which animal pictures were targets, combat-related pictures were distracter probes, and pictures of furniture were standard stimuli. The results showed that the probes elicited enhanced P3a in veterans diagnosed with PTSD, relative to a non-PTSD control group; but since there was no emotional control condition included, it was unclear whether the enhanced P3a related to combat pictures was due to their unpleasantness in general, or their combat-relevance. Subsequently, Stanford et al.7 conducted a study with PTSD patients, using two separate three-condition oddball tasks. The two tasks were identical, except that one had trauma-related threatening words as probes, whereas the other used socially-threatening words as probes. They found that an enhanced P3a was elicited by trauma-related probes, indicating that trauma relevance, rather than unpleasantness, affected the involuntary enhanced attention to trauma-related stimuli in PTSD. Worth noting, however, is that although some studies have shown an increase in P3a with threat-related stimuli, others have shown a decrease, reflecting the heterogeneity of expression of PTSD.8 Orr, Metzger, and Pitman9 reported a reduced ability to attend to task-relevant information and a reduced P3 response to trauma-related stimuli in PTSD patients. Araki et al.10 found that, among survivors of the Tokyo subway sarin attack, those with PTSD showed significantly reduced P300 amplitudes, and this measure among the PTSD group bore a significant negative correlation with their scores in the C Cluster (Avoidance/Numbing) of the Clinically-Administered PTSD Scale (CAPS). The task used by Araki et al. did not include trauma-related stimuli. Shucard et al.11 also investigated relationships between ERP components and CAPS subscores, but used neutral stimuli. Shucard et al. found that P3 amplitude was significantly correlated with hyperarousal subscores when both control subjects and PTSD patients were included, but this correlation did not reach significance among only-PTSD patients.
Treatment for the symptoms of PTSD has included psychotherapy,12 pharmacotherapy,13 and, more recently, repetitive transcranial magnetic stimulation (rTMS).14–17 Repetitive TMS is a safe, noninvasive procedure in which regional electrical activity in the brain is influenced by a pulsed magnetic field, generated by passing current pulses through a conducting coil placed on the scalp. High-intensity current is rapidly turned on and off in the coil through the discharge of capacitors, producing a time-varying magnetic field (1.5–2.0 Tesla strength) that lasts for 100–300 μsec. The time-varying magnetic field will result in current flow in neural tissue, thereby activating underlying cortex.18 It is estimated that there is an approximately 2-cm. magnetic field penetration from the scalp surface. Repetitive TMS thus offers a noninvasive method for altering excitability of the brain. Evidence from electrophysiology studies of motor cortex indicates that low-frequency rTMS (frequency ≤1 Hz) is inhibitory, and high-frequency rTMS (frequency >1 Hz) is excitatory to underlying neural tissue.19
Both high-frequency and low-frequency rTMS to both right- and left-frontal areas have been tested for efficacy in relieving PTSD symptoms. Rosenberg et al.15 applied either 1-Hz or 5-Hz rTMS to the left dorsolateral frontal area 4 cm. rostral and 2 cm. lateral to the area where rTMS produced a motor evoked potential to the right abductor pollicis brevis (APB). There were no differences between the group that received the 1-Hz stimulation and the group that received the 5-Hz stimulation, but significant improvements on all six subscales of the Profile of Mood States (POMS) were observed. However, intrusions, or re-experiencing, a core symptom of PTSD, was not diminished. Cohen et al.16 applied either 1-Hz, 10-Hz, or sham rTMS to the right dorsolateral frontal area 5 cm. rostral to the motor cortex, which was established as where rTMS application induced APB movement 5 out of 10 times. Twenty-minutes of rTMS treatment per day for 10 days resulted in improvement on the Reexperiencing, Avoidance, and Hyperarousal CAPS subscores of the group that received the 10-Hz stimulation.
Davidson20 has suggested that whereas the left hemisphere predominantly mediates approach-based, often positive, emotional responses, the right hemisphere mediates withdrawal-based, often negative, emotional responses. Right-hemisphere lateralization for negative affective responses has also been demonstrated for fear recognition,21 and differential activation of the right hemisphere (as measured by PET [positron emission tomography] and fMRI [functional magnetic resonance imaging]) has been observed in adults who received repeatedly painful stimuli,22 experienced unpleasant tastes,23 or viewed photographs of negative emotional scenes.24 This right hemispheric–negative emotion predominance is also consistent with lateralization of the threat-processing circuit for nonverbal object threatening sounds, as demonstrated by fMRI.25 These consistent findings in both lesion-deficit and functional-activation studies strongly suggest that the threat-processing neural circuit is primarily lateralized to the right hemisphere for both the auditory and visual modality of threatening input.
The idea that stimulation of right- or left-dorsolateral frontal areas influenced different affective systems was supported by two cases reported by McCann et al.14 Two women diagnosed with PTSD had been treated for their depressive symptoms with pharmacological regiments and high-frequency rTMS to the left-frontal area. One patient's symptoms were resistant to these treatments, and the other's anxiety symptoms became so severe that the treatment was stopped. In these two cases, however, frequent low-frequency (1-Hz) rTMS applications to the right frontal area resulted in significantly reduced scores on a modified PTSD scale, as well as subjective reports of improvement. Osuch et al.17 also reported improvement in hyperarousal symptoms among PTSD patients when low-frequency rTMS was applied to the right frontal area 5 cm. rostral to the optimal site for producing a motor evoked potential in the left APB. In the Osuch et al. study, rTMS was applied to patients as they actively remembered their traumatic events. Electroencephalographic (EEG)26 and SPECT (single photon emission computed tomography)27 studies have shown that remembering the traumatic event has a greater effect in the right hemisphere than in the left. Cerebral blood flow to the right hemisphere increased more when PTSD patients were hearing trauma-related sounds,27 and alpha power decreased more in the right hemisphere in PTSD patients while they were viewing trauma-related pictures,26 whereas control subjects showed the opposite effect. However, in a study of healthy young adults, Coan and Allen28 did not find the expected relationship between alpha desynchronization indicating rightward asymmetry and withdrawal-oriented tendencies, but did find the expected relationship between leftward alpha desynchronization asymmetry and approach-oriented tendencies.
On the basis of the results of the McCann et al.14 and Osuch et al.17 studies, we chose to examine the effect of low-frequency rTMS on the evoked P3a and P3b responses of one PTSD patient who exhibited a robust pre-rTMS P3a to combat-threatening stimuli. We applied 1-Hz rTMS to the right and left prefrontal regions for 20 minutes on separate occasions. Because of the decreased hyperarousal symptoms reported in previous studies, and using the P3a as a marker of that hyperarousal, we expected to observe a reduced P3a response to trauma-related stimuli after rTMS was applied to the right-prefrontal area.
METHOD
Subject
The subject was a 58-year-old right-handed, married, male Vietnam War combat veteran, with 14 years of education. He had been diagnosed with PTSD and major depression, recurrent, by SCID (Structured Clinical Interview for DSM Disorders) criteria at the time of the study. The subject had no history of substance abuse and was a nonsmoker. His total CAPS score was reported as 68. His clinical presentation was marked by an intense, irritable affect, demonstrating significant hyperarousal and hypervigilance. He had been prescribed venlafaxine 150 mg per day at the time of the study, and had had previous trials of nefazodone, paroxetine, and paroxetine-combined-with valproate. He had been admitted to an acute psychiatry unit twice and had participated in a 7-week PTSD residential program. At the time of the study, he was unemployed and was receiving full disability from the VA. Vietnam combat experience was documented by a combat-designated military occupational specialty. The Human Use Committee of the University of Arkansas for Medical Sciences approved the research protocol, and we obtained written informed consent from the subject.
Task
The Behavioral Semantic Memory Task was a pre-rTMS ERP evaluation of responses to 50 color photographs from the International Affective Picture Set (IAPS). The stimuli in the photographs consisted of objects or people. The 50 photographs included 10 combat-threatening stimuli that had IAPS arousal and pleasantness mean ratings of 5.73 and 3.00, respectively, and were authenticated to be associated with the Vietnam War. There were 10 animal-threatening photographs, with 5.91 and 4.39 mean ratings, and 30 non-threatening stimuli that were not animals or combat-related, with ratings of 3.32 and 5.82. Each stimulus was presented twice during the initial testing session. There were 100 total stimuli, with the ratio of 60% non-target distracter stimuli (non-threatening objects), 20% target stimuli (threatening animals), and 20% probe stimuli (threatening combat-relevant stimuli that represented items present in the Vietnam theater). The instructions in this task were to push a button with the right index finger in response to pictures of animals. The subject was instructed not to respond when the picture was not of an animal. The subject was not informed that any of the stimuli would represent threatening animals or circumstances.
Procedures
Behavioral Semantic Memory Task
The stimuli were presented on a color computer video monitor, positioned approximately 1 meter in front of the subject. The stimuli subtended less than a 10-degree angle. Each stimulus was presented for 1 sec., followed by a 1-sec. fixation point, yielding a 2-sec. interstimulus interval. The subject sat in a comfortable chair in a sound-attenuated room and was told to keep his eyes focused on the center of the screen.
While the subject was performing the Behavioral Memory Task, his EEG was recorded in order to examine the P3a ERP component to the threatening combat stimuli and P3b ERP component to the target threatening animal stimuli. EEG was recorded at four sites: F3, F4, P3, and P4, and was referenced to linked mastoids. EEGs and electro-oculographs (EOG) were amplified within a bandwidth of 0.03 Hz–100Hz and digitized at 256 Hz. A linear-regression correction procedure was applied to estimate and correct the contribution of blink artifact. Trials containing horizontal or vertical eye movements other than blinks were rejected, as were those with A/D saturation of baseline drift exceeding ±50 μV. Each epoch consisted of 125 msec. before the onset of the stimulus to 1,000 msec. after stimulus onset. Epochs for three conditions—correctly recognized target threatening animals, non-target combat-related probes, and non-target non-threatening objects—were averaged for each EEG session. Each ERP average comprised at least 16 epochs. P3a and P3b amplitudes were measured from 250 msec. to 400 msec. for combat-related probe averages and 300 msec. to 650 msec. for animal target averages.
rTMS Procedures
The rTMS sessions of this study were not initiated until 1 week after completion of the initial Behavioral Semantic Memory Test with EEG recording. The rTMS study consisted of four steps: First, the patient received a shortened version of the Behavioral Semantic Memory Task while EEG was recorded from four scalp electrodes—F3, F4, P3, and P4—in order to assess immediate pre-rTMS ERP components. Second, the EEG electrodes were removed, and rTMS was applied to the right prefrontal cortex (PFC) at a frequency of 1 Hz for 20 minutes (1,200 pulses). The rTMS was delivered at 100% of the motor threshold, which was defined as the stimulus intensity that induced visually perceptible movement of the ipsilateral APB with 5 out of 10 consecutive stimulus applications,29 and was calculated for each rTMS session. After motor threshold determination, the stimulator coil was positioned 5 cm. anteriorly in the sagittal plane from the site of optimal simulation of the APB. The 1,200-pulse train was then delivered at 1 Hz at this PFC site. Third, and immediately after completion of the 20 minutes of rTMS, the electrodes were placed again on the patient's scalp at F3, F4, P3, and P4. Finally, the subject was administered a parallel version of the shortened Behavioral Semantic Memory Test for post-rTMS ERP measures. None of the stimuli presented in the pre-rTMS shortened Behavioral Semantic Memory Test was seen in the post-rTMS shortened Behavioral Semantic Memory Test. Each rTMS session took approximately 90 minutes to complete.
One week later, these same rTMS study procedures—pre-rTMS Behavioral Semantic Memory Test, with EEG, rTMS to the PFC at 1 Hz for 20 minutes, followed by post-rTMS Behavioral Semantic Memory Test with EEG—were repeated, with the exception that the rTMS was administered to the left PFC.
RESULTS
There were no adverse events associated with this procedure. We first examined the P3a and P3b components of the ERP in each condition from the full Behavioral Semantic Memory Test administered before the rTMS study in order to determine the subject's baseline.
The ERPs from the initial baseline Behavioral Semantic Memory Test with EEG showed a very robust parietal P3b in the threatening animal target averages. The subject also exhibited a notably robust P3a for threatening combat-related stimuli as well. The subject's baseline was compared with his pre- and post-rTMS ERPs derived from EEGs recorded before and after the administration of rTMS to right and left PFC. This was to determine whether the rTMS to the right or left PFC modified the P3a for combat threatening stimuli, P3b for animal threatening stimuli, or both.
Repetitive TMS to the left PFC resulted in no notable differences in the P3a or P3b ERP. However, rTMS to the right PFC dramatically decreased the P3a response to combat-related stimuli (Figure 1) by 54% in the left frontal and parietal leads and by 80% and 60% in the right frontal and parietal leads, respectively. The robust P3b response to the threatening animal target stimuli was not affected.
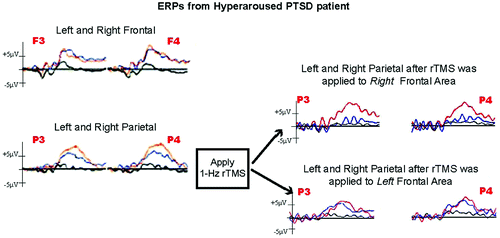
The left panel shows the waveforms from left- and right-frontal (top) and parietal (bottom) electrode sites before the application of 1-Hz repetitive transcranial magnetic stimulation (rTMS). The black waveform depicts the response to standard stimuli; the red wave is the response to the animal target stimuli; and the blue waveform is the response to the unattended combat-related stimuli. The top portion of the right panel shows the reduction in the P3a to unattended combat-related stimuli after rTMS application to the right frontal area, whereas the bottom portion shows that little change occurred after the rTMS application to the left frontal area.
DISCUSSION
By using the P3a as a marker of hyperarousal, the present study of one PTSD patient showed a transient reduction in the exaggerated response to trauma-related visual stimuli after an application of low-frequency rTMS to the right-frontal area, whereas application of low-frequency rTMS to the left-frontal area resulted in no noticeable change in the P3a. These findings supply electrophysiological correlates to the changes in subjective report of PTSD symptomatology observed in other studies of rTMS application to the right-frontal area.14,16,17 Of note, Cohen et al.16 showed significant improvement in patients' PTSD symptoms by using high-frequency rTMS, and McCann et al.14 and Osuch et al.17 showed significant improvement in PTSD symptoms using low-frequency rTMS. Brighina et al.30 found increased intracortical facilitation in migraineurs after application of low-frequency rTMS, which contrasted with the decreased facilitation observed in the control group as well as in groups in previous rTMS studies.19 Brighina et al.30 attributed this group effect to poor inhibitory circuitry subserving a hyperexcitable cortex in migraineurs. Although a group effect was observed, examination of individual subjects' responses revealed that not all subjects showed an increased facilitation. Rossi et al.31 pointed out that referring to the immediate effect of rTMS as a “virtual lesion”32 strongly suggests that rTMS is at least interfering with brain function. Thus, the findings of McCann et al.,14 Cohen et al.,16 and Osuch et al.17 imply that interference of right frontal lobe function via rTMS can temporarily ameliorate the neural underpinnings of the hyperarousal response to threatening stimuli in some patients with PTSD.
The present study, as well as previous PTSD studies using rTMS, applied the stimulation to the lateral frontal area 4 cm.–5 cm. anterior to where stimulation evoked an APB response. The specific area of the cortex that would be affected by magnetic stimulation can only be approximated, given the variation of brain topology from one individual to the next. The observed decrease in P3a amplitude to non-target, trauma-related stimuli could have been due to a decrease in activation of the dorsolateral prefrontal cortex (dlPFC; BA 9/46), which has been shown to be active in a variety of tasks, including retrieval of autobiographical memories.33 Emotional autobiographical memories have been shown to elicit more activity in the right dlPFC area in women.34 Deactivating the uncontrolled intrusion of autobiographical memories31 that mark PTSD could result in an attenuated attentional capture, whose electrophysiological correlate is the smaller P3a response.
The right ventral lateral PFC (vlPFC) and inferior frontal gyrus (BA 44, 45, 47/12)35 have shown a strong association with cognitive and emotional inhibition. Baudena et al.36 argued that the cortical area around the inferior frontal sulcus was the diffuse but highly integrated source of the frontal P3a, and that these areas' role in the orienting of attention was consistent with the attention-related variance demonstrated in the P3a component. Ermutlu et al.37 subsequently contended that the P3a was one of the markers of a multi-staged gating system whose gating efficiency is compromised under stress. Similarly, functional MRI (fMRI) studies have shown the vlPFC to be more active during tasks that require active suppression of distracting stimuli. Greater distraction, as with trauma-related stimuli, requires greater vlPFC activity. Morey et al.38 found that, relative to combat veterans without PTSD, combat veterans with PTSD showed more activation in the right vlPFC when viewing trauma-related stimuli during a visual working-memory task. This suggested that the inhibition of emotionally distracting stimuli required more effort in the PTSD group. The increase in the vlPFC activation was associated with decreased activation in the dlPFC and with increased activation of the amygdala. This was interpreted as the emotional distraction, signaled by amygdalar activation, being actively inhibited by vlPFC activation, which drew attentional resources away from the task at hand, as indicated by the decreased activation of the dlPFC.
A general principle describing the frontal lobe is the reciprocity of connections between frontal areas. The ventral PFC areas are richly connected to lateral PFC and amygdala.39,40 Although the neural pathways engaged in processing threatening and fear-inducing stimuli are likely input-modality dependent,25,41 the final common pathway appears to include two common brain regions: the right ventromedial frontal lobe and the amygdala. The engagement of these two regions in the threat-memory processing circuit has been detected by using lesion and pharmacological inactivation techniques in rats42,43 and by using PET and fMRI with humans.44,45 The right ventromedial frontal region has been purported to gate the access from object-recognition systems to the amygdala, where the fear or threat response is mediated.25,46,47 Some studies have suggested that the hyperarousal abnormalities in PTSD are related to dysfunction in this frontal-amygdalar relationship, suggesting that such dysfunction is associated with impaired responses to emotional facial stimuli,48 symptom provocation,49 or working memory50 in PTSD patients. These studies are typified by decreased activity in the medial inferior frontal region and concurrent increase in the amygdala (but see also, for example, Zubieta et al.51).
Although the limitations inherent in a single subject study must be acknowledged, the findings of the current study imply that interference with right frontal lobe functioning31 can temporarily ameliorate the neural underpinnings of the hyperarousal response to threatening stimuli in some patients with PTSD. The degree of rich and reciprocal connectivity among the dorsolateral, ventrolateral, and ventromedial prefrontal cortex, as well as their relationships to the amygdala—all of which have been found to be dysfunctional in individuals with PTSD (see, for example, Morey et al.38 and Rauch et al.52)—provides a complex combination of paths by which the rTMS interference may have affected the hyperarousal response. Future study should engage in establishing the specific roles of the relationships among the right-frontal areas in the range of PTSD symptomatology.
The use of the ERP P3a marker as an objective electrophysiological measure of the subjective reports of hyperarousal symptoms in this study extends previous findings14,16,17 of the efficacy of right-frontal rTMS application in attenuating the symptoms of PTSD.
1. : Meta-analytic review of event-related potential studies in post-traumatic stress disorder. Biol Psychol 2006; 71:123–147Crossref, Medline, Google Scholar
2. : Emotion-specific and emotion-non-specific components of posttraumatic stress disorder (PTSD): implications for a taxonomy of related psychopathology. Behav Res Ther 2004; 42:1069–1088Crossref, Medline, Google Scholar
3. : Stimulus novelty, task relevance, and the visual evoked potential in man. Electroencephalogr Clin Neurophysiol 1975; 39:131–143Crossref, Medline, Google Scholar
4. : The novelty P3: an event-related brain potential (ERP) sign of the brain's evaluation of novelty. Neurosci Biobehav Rev 2001; 25:355–373Crossref, Medline, Google Scholar
5. : Event-related brain potentials and affective responses to threat in spider/snake-phobic and non-phobic subjects. Int J Psychophysiol 2005; 57:43–52Crossref, Medline, Google Scholar
6. : Classification of veterans with post-traumatic stress disorder using visual brain evoked P3s to traumatic stimuli. Br J Psychiatry 1996; 168:110–115Crossref, Medline, Google Scholar
7. : Impact of threat relevance on P3 event-related potentials in combat-related post-traumatic stress disorder. Psychiatry Res 2001; 102:125–137Crossref, Medline, Google Scholar
8. : A review of neuroimaging studies in PTSD: heterogeneity of response to symptom provocation. J Psychiatr Res 2006; 40:709–729Crossref, Medline, Google Scholar
9. : Psychophysiology of post-traumatic stress disorder. Psychiatr Clin North Am 2002; 25:271–293Crossref, Medline, Google Scholar
10. : Association between lower P300 and smaller anterior cingulated cortex volume in patients with posttraumatic stress disorder: a study of victims of the Tokyo subway sarin attack. Neuroimage 2005; 25:43–50Crossref, Medline, Google Scholar
11. : An event-related potential study of attention deficits in posttraumatic stress disorder during auditory and visual Go/No-Go continuous performance tasks. Biol Psychol 2008; 79:223–233Crossref, Medline, Google Scholar
12. : Effective psychotherapies for posttraumatic stress disorder: a review and critique. CNS Spectr 2009; 14:32–43Medline, Google Scholar
13. : Post-traumatic stress disorder: emerging concepts of pharmacotherapy. Expert Opin Emerg Drugs 2009; 14:251–272Crossref, Medline, Google Scholar
14. : Repetitive transcranial magnetic stimulation for posttraumatic stress disorder. Arch Gen Psychiatry 1998; 55:276–279Crossref, Medline, Google Scholar
15. : Repetitive transcranial magnetic stimulation treatment of comorbid posttraumatic stress disorder and major depression. J Neuropsychiatry Clin Neurosci 2002; 14:270–276Link, Google Scholar
16. : Repetitive transcranial magnetic stimulation of the right dorsolateral prefrontal cortex in posttraumatic stress disorder: a double-blind, placebo-controlled study. Am J Psychiatry 2004; 161:515–524Crossref, Medline, Google Scholar
17. : Repetitive TMS combined with exposure therapy for PTSD: a preliminary study. J Anxiety Disord 2009; 23:54–59Crossref, Medline, Google Scholar
18. : Transcranial magnetic stimulation: a neuropsychiatric tool for the 21st century. J Neuropsychiatr 1996; 8:373–382Link, Google Scholar
19. : Therapeutic application of repetitive transcranial magnetic stimulation: a review. Clin Neurophysiol 2001; 112:1367–1377Crossref, Medline, Google Scholar
20. : Affective styles and affective disorders: perspectives from affective neuroscience. Cogn Emot 1998; 12:307–330Crossref, Google Scholar
21. : Cortical systems for the recognition of emotion in facial expressions. J Neurosci 1996; 16:7678–7687Crossref, Medline, Google Scholar
22. : Anticipatory coping of pain expressed in the human anterior cingulated cortex: a positron emission tomography study. Neurosci Lett 1999; 262:61–64Crossref, Medline, Google Scholar
23. : Aversive gustatory stimulation activates limbic circuits in humans. Brain 1998; 121:1143–1154Crossref, Medline, Google Scholar
24. : Hemispheric asymmetry for emotional stimuli detected with fMRI. Neuroreport 1998; 9:3233–3239Crossref, Medline, Google Scholar
25. : Neuroanatomic organization of sound memory in humans. J Cogn Neurosci 2006; 9:1031–1040Google Scholar
26. : Regional brain electrical activity in posttraumatic stress disorder after motor vehicle accident. J Abnorm Psychol 2006; 115:687–698Crossref, Medline, Google Scholar
27. : Regional cerebral blood flow during auditory recall in 47 subjects exposed to assaultive and non-assaultive trauma and developing or not developing posttraumatic stress disorder. Eur Arch Psychiatry Clin Neurosci 2005; 255:359–365Crossref, Medline, Google Scholar
28. : Frontal EEG asymmetry and the behavioral activation and inhibition systems. Psychophysiology 2003; 40:106–114Crossref, Medline, Google Scholar
29. : Motor threshold in transcranial magnetic stimulation: comparison of three estimation methods. Neurophysiol Clin 2006; 36:1–7Crossref, Medline, Google Scholar
30. : Facilitatory effects of 1-Hz rTMS in motor cortex of patients affected by migraine with aura. Exp Brain Res 2005; 161:34–38Crossref, Medline, Google Scholar
31. : rTMS for PTSD: induced merciful oblivion or elimination of abnormal hypermnesia? Behav Neurol 2006; 17:195–199Crossref, Medline, Google Scholar
32. : Transcranial magnetic stimulation in cognitive neuroscience: virtual lesion, chronometry, and functional connectivity. Curr Opin Neurobiol 2000; 10:232–237Crossref, Medline, Google Scholar
33. : Cerebral representation of one's own past: neural networks involved in autobiographical memory. J Neurosci 1996; 16:4275–4282Crossref, Medline, Google Scholar
34. : Gender differences in the functional neuroanatomy of emotional episodic autobiographical memory. Hum Brain Mapp 2005; 24:313–324Crossref, Medline, Google Scholar
35. : Inhibition and the right inferior frontal cortex. Trends Cogn Sci 2004; 8:170–177Crossref, Medline, Google Scholar
36. : Intracerebral potentials to rare target and distracter auditory and visual stimuli, III: frontal cortex. Electroencephalogr Clin Neurophysiol 1995; 94:251–264Crossref, Medline, Google Scholar
37. : Effects of cold stress on early and late stimulus gating. Psychiatry Res 2005; 136:201–209Crossref, Medline, Google Scholar
38. : The role of trauma-related distracters on neural systems for working memory and emotion-processing in posttraumatic stress disorder. J Psychiatr Res 2009; 43:809–817Crossref, Medline, Google Scholar
39. : The Prefrontal Cortex: Anatomy, Physiology, and Neuropsychology of the Frontal Lobe. Philadelphia, PA, Raven, 1997Google Scholar
40. : Prefrontal involvement in the regulation of emotion: convergence of rat and human studies. Curr Opin Neurobiol 2006; 16:723–727Crossref, Medline, Google Scholar
41. : Neural substrates of semantic memory. J Int Neuropsychol Soc 2007; 13:865–880Crossref, Medline, Google Scholar
42. : The role of ventromedial prefrontal cortex in the recovery of extinguished fear. J Neurosci 2000; 20:6225–6231Crossref, Medline, Google Scholar
43. : Stimulation of medial prefrontal cortex decreases responsiveness of central amygdala output neurons. J Neurosci 2003; 23:8800–8807Crossref, Medline, Google Scholar
44. : Fear extinction in rats: implications for human brain imaging and anxiety disorders. Biol Psychol 2006; 73:61–71Crossref, Medline, Google Scholar
45. : Neuroimaging and the neuroanatomy of PTSD. CNS Spectr 1998; 3(suppl 2):30–41Crossref, Google Scholar
46. : Dissociable amygdala and orbitofrontal responses during reversal fear conditioning. Neuroimage 2004; 22:372–380Crossref, Medline, Google Scholar
47. : Neural systems for orienting attention to the location of threat signals: an event-related fMRI study. Neuroimage 2006; 31:920–933Crossref, Medline, Google Scholar
48. : Exaggerated amygdala response to masked facial stimuli in posttraumatic stress disorder: a functional MRI study. Biol Psychiatry 2000; 47:769–776Crossref, Medline, Google Scholar
49. : Regional cerebral blood flow in the amygdala and medial prefrontal cortex during traumatic imagery in male and female Vietnam veterans with PTSD. Arch Gen Psychiatry 2004; 61:168–176Crossref, Medline, Google Scholar
50. : Abnormal functional connectivity in posttraumatic stress disorder. Neuroimage 2002; 15:661–674Crossref, Medline, Google Scholar
51. : Medial frontal cortex involvement in PTSD symptoms: a SPECT study. J Psychiatr Res 1999; 33:259–264Crossref, Medline, Google Scholar
52. : Neurocircuitry models of posttraumatic stress disorder and extinction: human neuroimaging research—past, present, and future. Biol Psychiatry 2006; 60:376–382Crossref, Medline, Google Scholar
53. : Selectively reduced regional cortical volumes in posttraumatic stress disorder. Neuroreport 2003; 14:913–916Crossref, Medline, Google Scholar